During the past three decades it has been my privilege to work alongside Professor Emerita Jennifer A. Clack, FRS – Jenny to her friends – on the origin of tetrapods and, more broadly, on the evolution of Devonian and Carboniferous vertebrates, initially as her first PhD student, then as long-standing collaborator and occasional sparring partner. It has been a time of extraordinary progress, during which our knowledge of the fish–tetrapod transition has grown beyond all recognition, and the new discoveries have made their way into the popular awareness as well as informing the work of the specialists. In large measure, the credit for this can be laid at Jenny's feet, both because of her own discoveries and because of the way her personal qualities have infused and brightened the research community.
The occasion of Jenny's Festschrift would be a natural starting point for a review of the progress that has been made during these years. However, right now I think something slightly different is needed, because there is an odd sense of unease pervading the research community. It has been brought about by a head-on collision between an established interpretative scenario for the origin of tetrapods, seemingly well-grounded in the body fossil record, and two sets of tetrapod trace fossils from Poland and Ireland that appear to flatly contradict it. What I present here is my personal take on the problem, as an attempt to do justice to all the available data and place it in a common temporal, environmental and phylogenetic frame of reference. The result is an outline of a new interpretative scenario for the origin of tetrapods. I argue that the early fossil record of the tetrapod lineage, and indeed of the terrestrial Devonian world as a whole, is much less complete than we have been tempted to think; that elpistostegids and tetrapods originated no later than the beginning of the Middle Devonian, and coexisted as ecologically separated radiations for at least 15 million years; that the evolution of tetrapods was driven by selection pressure towards terrestrialisation and quickly led to a reasonable degree of terrestrial competence; that all Devonian and many later tetrapods nevertheless retained a permanent dependence on the aquatic environment, allowing only relatively brief terrestrial excursions; that this continued dependence explains the repeated evolution of ‘secondarily aquatic' forms among them; and that all fully aquatic tetrapods, including Acanthostega, are likely to represent reversals from a somewhat more terrestrial ancestry.
1. Historical context: how did we get here?
The discipline of Devonian tetrapod studies was born in 1932 with the description of the genus Ichthyostega from Famennian deposits in East Greenland (Säve-Söderbergh Reference Säve-Söderbergh1932). Until that time, the earliest known tetrapods had been of mid-Carboniferous age, with only anatomically tetrapod-like fishes such as Eusthenopteron hinting at an earlier history for the lineage. The discovery of Ichthyostega not only parachuted an actual fossil taxon into a time frame previously only occupied by speculative scenarios (e.g., Barrell Reference Barrell1916), but also effectively created ‘Romer's Gap' by implying the existence of one or more tetrapod ghost lineages spanning the whole of the Early Carboniferous. Note that the term ‘tetrapod' will be used throughout this paper in the traditional sense of ‘vertebrate with digit-bearing limbs rather than paired fins'. The group thus defined is a clade encompassing the tetrapod crown group and upper part of the stem group. ‘Early tetrapods' is used to denote the paraphyletic group containing those tetrapods that fall outside the amniote and lissamphibian crown groups. The oldest ‘early tetrapods' known from body fossils are Elginerpeton, Obruchevichthys and Webererpeton from the late Frasnian (Vorobyeva Reference Vorobyeva1977; Ahlberg Reference Ahlberg1991, Reference Ahlberg and Trewin1995, Reference Ahlberg and Clack1998, Reference Ahlberg2011; Ahlberg & Clack Reference Ahlberg and Johanson1998; Ahlberg et al. Reference Ahlberg, Friedman and Blom2005b; Clément & Lebedev Reference Clément and Lebedev2014); the youngest is the temnospondyl Koolasuchus from the Early Cretaceous (Warren et al. Reference Warren, Rich and Vickers-Rich1997). All geological dates are taken from the 2017 International Chronostratigraphic Chart produced by the International Commission on Stratigraphy (http://www.stratigraphy.org/index.php/ics-chart-timescale).
Although their scientific potential was immediately apparent, research on the Greenland fossils progressed rather slowly after the ill health of Gunnar Säve-Söderbergh caused them to be reassigned to Erik Jarvik. Jarvik eventually published a detailed account of the postcranial skeleton, accompanied by descriptions of a new tetrapod genus, Acanthostega, and a large Eusthenopteron-like lobe-fin, Eusthenodon, from the same Late Devonian strata (Jarvik Reference Jarvik1952). Disregarding some minor papers (Jarvik Reference Jarvik1963, Reference Jarvik1967), the next substantive publication on Ichthyostega was Jarvik's book, Basic Structure and Evolution of Vertebrates (Jarvik Reference Jarvik1980), which was followed by a final monograph two years before his death (Jarvik 1996). This slow rate of publication, together with the puzzling morphology of Ichthyostega, the limited accessibility of the material, the sometimes strained relationship between the ‘Stockholm School' and members of the Anglo-American research community, Jarvik's categoric rejection of cladistic methodology and his adherence to the diphyletic theory of tetrapod origins (Jarvik Reference Jarvik1942, Reference Jarvik1972, Reference Jarvik1980), which found little favour in the wider community, effectively relegated Ichthyostega to the dual role of ‘interesting oddity' and stratigraphic placeholder for the origin of tetrapods (e.g., Milner et al. Reference Milner, Smithson, Milner, Coates and Rolfe1986). Acanthostega was scarcely considered due to the fragmentary nature of the material.
Two discoveries during the 1980s drastically changed both the understanding and the perceived utility of Devonian tetrapod fossils. The first was Tulerpeton from the latest Famennian of Russia (Lebedev Reference Lebedev1984). The second was, of course, the extensive new material of Acanthostega collected by Jenny Clack during the 1987 Cambridge-Copenhagen expedition to East Greenland. During the next decade and a half, both Tulerpeton and Acanthostega were further illuminated by a series of landmark papers that completely changed the picture of Devonian tetrapods and brought them into the palaeontological mainstream (Clack Reference Clack1988, Reference Clack1989, Reference Clack1994a, Reference Clackb, Reference Clack1998, Reference Clack2002a, Reference Clackb, Reference Clack2012; Coates & Clack Reference Coates and Clack1990, Reference Coates and Clack1991; Lebedev & Clack Reference Lebedev and Clack1993; Clack & Coates Reference Clack, Coates, Arsenault, Lelievre and Janvier1995; Lebedev & Coates Reference Lebedev and Coates1995; Coates Reference Coates1996; Ahlberg & Clack Reference Ahlberg and Johanson1998; Porro et al. Reference Porro, Rayfield and Clack2015). Acanthostega in particular proved enormously influential, so much so that it received the ultimate accolade of inspiring the title and cover illustration of one of Stephen Jay Gould's essay collections (Gould Reference Gould1993). A number of factors, in addition to the quality of the specimens themselves, facilitated this outcome. The material was published promptly in a series of short-to-medium-length papers in high-visibility journals; the theoretical framework was up to date, embracing cladistic analysis and rejecting the diphyletic theory of tetrapod origins; and the era of ‘Schools' was drawing to a close, replaced by a new era of greater openness and fluctuating collaborative constellations that continues to this day. Jenny was not only a beneficiary of this sea change in the mood of the community, but one of the key people that made it happen, through her openness and generosity of spirit; a scientific achievement as valuable as any, but not as easily documented or as visible to posterity as the ‘quantifiable outputs' beloved of our funding bodies.
Acanthostega has another characteristic that may have increased its impact on the field: a certain ‘morphological compatibility' with post-Devonian tetrapods. Granted, it boosts an impressive suite of primitive characteristics absent from later tetrapods, including a large lepidotrichial tail fin, a fully notochordal occiput and a scapulocoracoid that lacks a scapular blade, but in most other respects it is broadly comparable with post-Devonian ‘early tetrapods'. For example, its otic capsule resembles that of embolomeres, and its humerus carries a standard set of readily identifiable muscle attachments (Clack Reference Clack1994b, Reference Clack1998; Coates Reference Coates1996). Its strangest features, the eight-digit feet, were readily accepted as compatible with its phylogenetic position as a very deep-branching tetrapod (Coates & Clack Reference Coates and Clack1990; Gould Reference Gould1993; Coates et al. Reference Coates, Jeffery and Ruta2002). This contrasted with Ichthyostega, where the puzzles bequeathed by Jarvik (Reference Jarvik1952, Reference Jarvik1980, Reference Jarvik1996) included an incomprehensible otoccipital morphology, a humerus with muscle attachments seemingly quite different from those of other early tetrapods and an ankle morphology so peculiar that Carroll (Reference Carroll1988) simply dismissed it in favour of his own more conventional reconstruction, created without direct reference to the fossil material. New material of Ichthyostega collected during Jenny's 1987 and 1998 expeditions, together with a re-examination of Jarvik's original material, eventually allowed the morphology to be re-evaluated. Jarvik's ankle reconstruction was vindicated, but both the braincase and the humerus were reinterpreted, as was the axial skeleton (Coates & Clack Reference Coates and Clack1990; Coates et al. Reference Coates, Jeffery and Ruta2002; Clack et al. Reference Clack, Ahlberg, Finney, Dominguez Alonso, Robinson and Ketcham2003; Ahlberg et al. Reference Ahlberg, Clack and Blom2005a). The end result was a comprehensible but highly distinctive animal, clearly more terrestrially adapted than Acanthostega with a smaller tail fin and more robust postcranial skeleton, but without its appealing sense of being a believable hypothetical ancestor for later tetrapods.
The recognition that tetrapods could be distinguished from lobe-finned fishes on the basis of isolated elements such as lower-jaw fragments, humeri and pectoral girdles led to a wave of new discoveries that greatly expanded our understanding of Devonian tetrapod diversity and distribution (Ahlberg Reference Ahlberg1991, Reference Ahlberg1995; Ahlberg et al. Reference Ahlberg, Luksevics and Lebedev1994, Reference Ahlberg, Clack, Luksevics, Blom and Zupins2008; Daeschler et al. Reference Daeschler, Thomson and Amaral1994; Ahlberg & Clack Reference Ahlberg and Clack1998; Daeschler Reference Daeschler2000; Zhu et al. Reference Zhu, Ahlberg, Zhao and Jia2002; Clément et al. Reference Clément, Ahlberg, Blieck, Blom, Clack, Poty, Thorez and Janvier2004; Lebedev Reference Lebedev2004; Shubin et al. Reference Shubin, Daeschler and Coates2004). However, with the partial exception of Ventastega (Ahlberg et al. Reference Ahlberg, Luksevics and Lebedev1994, Reference Ahlberg, Clack, Luksevics, Blom and Zupins2008; Ahlberg & Clack Reference Ahlberg and Clack1998), none of these new tetrapods was complete enough to provide an overall impression of the gestalt of the animal. As a result, the Greenland tetrapods – and, in particular, Acanthostega – continued to dominate the discussion and scenario-building about the fish–tetrapod transition (Ahlberg & Milner Reference Ahlberg, Luksevics and Lebedev1994; Clack & Coates Reference Clack, Coates, Arsenault, Lelievre and Janvier1995; Clack Reference Clack2002a, Reference Clackb, Reference Clack2005, Reference Clack2006). One of the most striking points about Acanthostega, compared to later aquatically adapted tetrapods, was that it appeared to retain genuine fish characters rather than display convergent solutions to the problems of life in water. This led naturally to the conclusion that Acanthostega was primitively aquatic, and had acquired its tetrapod characteristics such as digits, enlarged pelvis and sacrum without ever leaving the water (Clack & Coates Reference Clack, Coates, Arsenault, Lelievre and Janvier1995; Clack Reference Clack2002a, Reference Clackb, Reference Clack2005, Reference Clack2006). Ichthyostega was interpreted as both more terrestrially adapted and more crownward.
At the same time as this flood of new data was changing the perceptions of Devonian tetrapods, a second piece of the jigsaw fell into place with the description of articulated material of the ‘elpistostegids' Elpistostege, Panderichthys and Tiktaalik (Vorobyeva Reference Vorobyeva1980, 1995; Schultze & Arsenault Reference Schultze and Arsenault1985; Vorobyeva & Schultze Reference Vorobyeva, Schultze, Schultze and Treub1991; Boisvert Reference Boisvert2005; Brazeau & Ahlberg Reference Brazeau and Ahlberg2006; Daeschler et al. Reference Daeschler, Shubin and Jenkins2006; Shubin et al. Reference Shubin, Daeschler and Jenkins2006, Reference Shubin, Daeschler and Jenkins2014; Boisvert et al. Reference Boisvert, Mark-Kurik and Ahlberg2008; Downs et al. Reference Downs, Daeschler, Jenkins and Shubin2008). Elpistostege (Westoll Reference Westoll1938) and Panderichthys (Gross Reference Gross1941) had previously been known only from tantalising fragments, whereas Tiktaalik was a new discovery. The described material now includes a partial skull and partial vertebral column of Elpistostege, almost the whole of Panderichthys and all of Tiktaalik except the tail. A complete, articulated specimen of Elpistostege is currently under description. Elpistostegids provide a glimpse of the first stage of the fish–tetrapod transition, before paired fins turned to limbs; they are not only anatomically tetrapod-like, like Eusthenopteron and other ‘osteolepiform' tetrapodomorph fishes, but have a body morphology approaching that of early tetrapods – elongated, crocodile-like, with raised eyes and a tail fin resembling that of Acanthostega. In age they range from latest Givetian to mid-Frasnian, whereas the earliest tetrapod body fossils are late Frasnian.
Up until 2010, the body fossil evidence from elpistostegids and Devonian tetrapods appeared to combine almost seamlessly into a coherent picture of the fish–tetrapod transition: tetrapods had evolved from elpistostegids sometime during the Frasnian, with novelties such as digits and a sacrum evolving in an aquatic environment (perhaps associated with ‘clambering' substrate locomotion in spatially complex settings like flooded forest vegetation) and real terrestrialisation beginning only later (Ahlberg & Milner Reference Ahlberg and Milner1994; Clack & Coates Reference Clack, Coates, Arsenault, Lelievre and Janvier1995; Clack Reference Clack2002a, Reference Clackb, Reference Clack2005, Reference Clack2006). However, the description of Tiktaalik gave a slightly different nuance to the story by stressing the presence of terrestrial adaptations in this elpistostegid (Daeschler et al. Reference Daeschler, Shubin and Jenkins2006; Shubin et al. Reference Shubin, Daeschler and Jenkins2006). Devonian tetrapod trackways were known, but were held to be of similar age as the body fossils (Clack Reference Clack1997).
This tidy picture was upended by the publication in January 2010 of well-preserved tetrapod footprints with digit impression from an early Middle Devonian (Eifelian) locality, Zachełmie Quarry, in Poland (Niedzwiedzki et al. Reference Niedzwiedzki, Szrek, Narkiewicz, Narkiewicz and Ahlberg2010). Together with the secure dating of the long-recognised tetrapod footprints from Valentia Island in Ireland as late Givetian (Williams et al. Reference Williams, Sergeev, Stössel, Ford, Higgs, Friend and Williams2000; Stössel et al. Reference Stössel, Williams and Higgs2016), this discovery implies that tetrapods have a ghost lineage extending back almost 20 million years before the earliest known tetrapod body fossils, and ten million years before the earliest elpistostegids. It is fair to say that these footprint data, unlike every previous advance in our knowledge of Devonian tetrapods, got a mixed reception. Some engaged enthusiastically with them and used them as a jumping-off point for examining the quality of the tetrapod fossil record (Friedman & Brazeau Reference Friedman and Brazeau2010), others were puzzled (Janvier & Clément Reference Janvier and Clément2010) and yet others went to considerable lengths to dismiss the footprint record by arguing that they might have been made by fishes (King et al. Reference King, Shubin, Coates and Hale2011; Lucas Reference Lucas2015; Falkingham & Horner Reference Falkingham and Horner2016). An argument was also put forward, on the basis of a biomechanical interpretation of Ichthyostega, that the trackways might belong to tetrapods of some sort but could not have been made by animals resembling the known Devonian body fossil taxa (Pierce et al. Reference Pierce, Clack and Hutchinson2012). And so here we are, in 2018, still locked in an impasse between two fundamentally incompatible timelines for the origin of tetrapods, each with its followers. How do we move forward?
2. Understanding the transition: the meaning of ‘halfway up'
As a problem of adaptive evolution, the fish–tetrapod transition has something in common with the second verse of the old nursery rhyme, The Grand Old Duke of York:
What exactly does it mean to be ‘halfway up', and when in the evolution of tetrapods did this phase occur? We can safely assume that a long transitional period would have been needed to acquire and fine-tune the adaptations needed for terrestrial life, but how long exactly? And was this transitional phase an evolutionary one-way street from water to land? Were they all marching up the hill? It is tempting to think so, because progressivist notions of evolution are hard to shake off and, of course, the overall end result of the process really was a transition from water to land, but we should be mindful that evolution has no overall aim and that small-scale reversals and parallelisms are commonplace phenomena.
Understanding the water-to-land transition is made more difficult by the limited relevance of modern analogues. The environmental context of the present day combines physical constants that are unchanged since the Devonian (the density and other physical properties of water and air, the salinity of the sea, the pull of gravity) with other physical features where the specific values have changed to some degree (for example, the oxygen content of the atmosphere) – and with terrestrial ecosystems that are utterly unlike those of the Devonian, crowded with well-adapted potential competitors and predators that were not there 380 million years ago. This means that a modern-day inhabitant of the ‘halfway up' zone, such as a mudskipper, faces pretty much the same physical problems as a Devonian transitional fish–tetrapod, but has a radically different (and, realistically, more limited) range of ecological options to explore. It also means that the lifestyles of the Devonian transitionals may have been quite different from any modern analogues. To take an obvious example, there is nothing alive today resembling an elpistostegid, and it is probably safe to assume that its lifestyle is not replicated by any modern animal.
How, then, can we make biological interpretations of these organisms that are both detailed and rigorous? I think the key is to itemise the animal as far as possible into discrete characters and consider the functional significance of each; this begins to constrain the interpretations of its mode of life, removing options until what remains is an ‘envelope of possibility' within which its actual (and unknowable) lifestyle can be assumed to be contained. For example, the dentition of Acanthostega shows that it was a predator, most probably a fish-eater, while its lateral-line canals and tail fin show that it spent a substantial proportion of its time in the water and never allowed its skin to dry out (Clack Reference Clack1994a; Coates Reference Coates1996; Ahlberg & Clack Reference Ahlberg and Clack1998). These few points already eliminate a wide range of imaginable lifestyles. A more complete itemisation will further constrain the interpretation. Note, however, that some of the constraints are not very tight: the lateral-line canals and tail fin do not, on their own, allow us to determine whether Acanthostega was wholly aquatic or just habitually aquatic. They only rule out habitual to complete terrestriality.
In a similar manner, the environmental setting of the fish–tetrapod transition can only be studied in terms of specific environmental data for specific taxa. But even these data have limitations. The palaeoenvironmental context of a body fossil shows us where the dead body ended up, and may or may not be informative about its living environment. Trackway data have the great advantage that they show living tetrapods operating in specific environments, but the glimpses they provide are painfully brief: for example, Zachełmie track Muz. PGI (Museum of the Polish Geological Institute) 1728.II.16 (Niedzwiedzki et al. Reference Niedzwiedzki, Szrek, Narkiewicz, Narkiewicz and Ahlberg2010, fig. 2a) probably captures no more than 15seconds in the life of a small tetrapod that, just then, was performing a lateral sequence walk in a shallow coastal-plain lake (see Section 5). We have no way of knowing what it did later that day, never mind with the rest of its life.
I would argue that four main strands of evidence can be informative regarding the degree of terrestrial adaptation of any particular taxon: evidence for substrate locomotion, for weight support, for aerial exposure of parts (or all) of the body and for terrestrial feeding. However, the relationship of these to each other and to terrestriality is not straightforward. Substrate locomotion can be performed at any water depth down to the abyssal plain of the ocean, as exemplified today by ogcocephalid batfishes (Bertelsen & Pietsch Reference Bertelsen, Pietsch, Paxton and Eschmeyer1998). Recent work on the little skate Leucoraja has uncovered a Hox transcription factor-dependent program for appendage innervation, remarkably similar to that in tetrapods and apparently homologous with it, which plays a key role in allowing the pelvic fins to perform walking movements (Jung et al. Reference Jung, Baek, D'Elia, Boisvert, Currie, Tay, Venkatesh, Brown, Heguy, Schoppik and Dasen2018). A basic capacity for underwater walking may thus have been present already at the gnathostome crown group node (see also King et al. Reference King, Shubin, Coates and Hale2011). Aerial exposure can be employed by free-swimming fishes at the top of the water column, such as the ‘four-eyed' fish Anableps, which has dorsally projecting eyes adapted to aerial vision that it uses to locate the flying insects on which it feeds (Nelson et al. Reference Nelson, Grande and Wilson2016). On the other hand, evidence for substrate locomotion and aerial exposure in one and the same taxon does suggest activity in extremely shallow water, where the surface and bottom are brought close together. Weight-bearing adaptations are perhaps the most interesting category because they are directly linked to leaving the supporting embrace of the water and having to resist the pull of gravity. Evidence for terrestrial feeding is potentially highly informative about the palaeoecology and behaviour of the animal, but as we shall see it tends to be subtle and ambiguous.
The main characteristics I will examine in relation to these four strands of evidence are the position and size of the eyes, the development of the lateral-line canals, the strength of the axial skeleton, the general morphology of the paired appendages and limb girdles, the morphology of the elbow, the presence or absence of a sacrum, the condition of the gill cover, and the morphology and dentition of the jaws. These characters are known from a range of elpistostegids and tetrapods, and can thus be considered in a comparative context. In addition, I will examine two interesting but unique pieces of evidence from single taxa: the ontogenetic data from Acanthostega (Sanchez et al. Reference Sanchez, Tafforeau, Clack and Ahlberg2016) and the evidence provided by a limb-bone fracture in Ossinodus (Bishop et al. Reference Bishop, Walmsley, Phillips, Quayle, Boisvert and McHenry2015).
There is a further aspect to some of these adaptations that makes the functional, ecological and evolutionary interpretation of early tetrapods even more complex, and which has perhaps not received sufficient attention. Once acquired, they tend not to be lost again, even if the lineage returns to a wholly aquatic lifestyle. A telling present-day example is furnished by the most aquatic salamanders, such as the ‘Congo eel' Amphiuma. This is an eel-shaped, permanently aquatic salamander with extremely reduced limbs that lack any weight-bearing capacity. Nevertheless, it possesses limbs with digits, a flexed elbow, limb girdles of characteristic tetrapod form and a vertebral column with zygapophyses (Low Reference Low1929). These structures only make sense as vestiges of a more terrestrial ancestry, and are invariably absent in morphologically and ecologically similar but primitively aquatic fishes such as the lungfishes Lepidosiren and Protopterus (Goodrich Reference Goodrich1930). Thus, when evaluating the significance of these characters in a particular taxon, we also need to consider whether they tell us about the immediate adaptive needs of the animal or simply about its evolutionary history – and whether we can tell these signals apart.
3. Elpistostegids
The body fossil record gives the impression that the elpistostegids were a short-lived transitional stage between conventional tetrapodomorph fishes and tetrapods, but the footprint record (see Section 4) shows that this cannot be true. The phylogenetic evidence placing the elpistostegids immediately anti-crownward to the Devonian tetrapods is compelling; this, in turn, implies that the elpistostegids originated no later than the early Eifelian and continued to exist alongside the tetrapods until the mid-Frasnian. The two most fully described elpistostegids are Panderichthys from the late Givetian of the Baltic States and Tiktaalik from early Frasnian of Ellesmere Island, Canada (Vorobyeva Reference Vorobyeva1980, Reference Vorobyeva1995; Vorobyeva & Schultze Reference Vorobyeva, Schultze, Schultze and Treub1991; Ahlberg et al. Reference Ahlberg, Clack and Luksevics1996; Boisvert Reference Boisvert2005; Brazeau & Ahlberg Reference Brazeau and Ahlberg2006; Daeschler et al. Reference Daeschler, Shubin and Jenkins2006; Shubin et al. Reference Shubin, Daeschler and Jenkins2006, Reference Shubin, Daeschler and Jenkins2014; Boisvert et al. Reference Boisvert, Mark-Kurik and Ahlberg2008; Downs et al. Reference Downs, Daeschler, Jenkins and Shubin2008). Elpistostege from Miguasha, Québec, Canada, is broadly contemporary with Tiktaalik. In addition to some fragmentary specimens (Westoll Reference Westoll1938; Schultze & Arsenault Reference Schultze and Arsenault1985), Elpistostege is now known from a complete articulated individual, discovered in 2013, which has been figured in some detail on the website of Societé des établissements de plein air du Québec (Sépaq) but has not yet been formally described (Matton & Lemieux Reference Matton and Lemieux2013). Although it would be inappropriate to trespass on the description of such an exceptionally important specimen, it is, I think, legitimate to note that it closely resembles Panderichthys and Tiktaalik in general form. The head is superficially crocodile-like, with close-set and dorsally positioned eyes under raised bony ‘eyebrows' (a feature already known from previous specimens), the body is slender, the tail is elongate and the paired appendages carry lepidotrichia.
In most respects, Tiktaalik is more tetrapod-like than Panderichthys, and it is always recovered crownward to Panderichthys in phylogenetic analyses. As it is also younger, this has been interpreted as a case of stratophylogenetic fit. However, the fragmentary late Givetian genus Livoniana, which occurs together with Panderichthys, has a lower-jaw morphology more tetrapod-like than that of Tiktaalik (Ahlberg et al. Reference Ahlberg, Luksevics and Mark-Kurik2000). Elpistostege closely resembles Tiktaalik in some respects, notably the proportions of the head (Schultze & Arsenault Reference Schultze and Arsenault1985; Daeschler et al. Reference Daeschler, Shubin and Jenkins2006), but the new complete specimen also reveals differences between the two genera.
An important new perspective on the fish–tetrapod transition was provided by MacIver et al. (Reference MacIver, Schmitz, Mugan, Murphey and Mobley2017) with what they call the ‘buena vista hypothesis'. They noted that the eyes of elpistostegids and tetrapods are not only shifted into a dorsal position on the skull, relative to the lateral position they occupy in osteolepiform fishes such as Eusthenopteron, but are also proportionately enlarged. Computer simulations indicate that such enlargement of the eyes provides no significant advantage in typical fresh water, but produces huge increases in visual acuity and sensitivity in air (MacIver et al. Reference MacIver, Schmitz, Mugan, Murphey and Mobley2017). There is thus good reason to believe that this change in eye position and morphology is an adaptation to aerial vision.
Elpistostegids show unmistakable adaptations for substrate locomotion and, arguably, also for weight support (Figs 1–3). It is instructive in this regard to compare them with the large Late Devonian (probably Frasnian) tristichopterid Mandageria from Canowindra, Australia (Johanson & Ahlberg Reference Johanson and Ahlberg1997), which is similar in size to an elpistostegid and also has an elongated, long-snouted head, but retains dorsal and anal fins, a short and deep caudal fin and small laterally positioned eyes. In Mandageria, like in Eusthenopteron, the shoulder girdle has a small scapulocoracoid with a posteriorly oriented glenoid, the humerus is short and the ribs are very small (Johanson & Ahlberg Reference Johanson and Ahlberg1997). In elpistostegids, on the other hand, the scapulocoracoid is enlarged with increased lateral exposure, the glenoid is rotated laterally and the humerus is elongated (Vorobyeva Reference Vorobyeva1995; Shubin et al. Reference Shubin, Daeschler and Jenkins2006; Boisvert et al. Reference Boisvert, Mark-Kurik and Ahlberg2008). In Tiktaalik, which has the best preserved and most fully described pectoral fin skeleton of any elpistostegid, the shapes of the joint surfaces between the distal elements suggests a capacity for hyperextension, likely associated with upwards flexion of the fin as it was pressed against the substrate by the weight of the body (Shubin et al. Reference Shubin, Daeschler and Jenkins2006). These characteristics strongly suggest that the pectoral fins were adapted for ‘walking' locomotion, with endoskeletons long enough to lift the body off the substrate and laterally rotated glenoids to increase stride length. Compared to tristichopterids, the ribs are longer and blade-like or flanged (Jarvik Reference Jarvik1980; Schultze & Arsenault Reference Schultze and Arsenault1985; Vorobyeva & Schultze Reference Vorobyeva, Schultze, Schultze and Treub1991; Johanson & Ahlberg Reference Johanson and Ahlberg1997; Shubin et al. Reference Shubin, Daeschler and Jenkins2006). (In the partial vertebral column of Elpistostege described by Schultze & Arsenault (Reference Schultze and Arsenault1985), the ribs were misidentified as neural arches.) This looks like an adaptation for strengthening the dorsal body-wall musculature of the thoracic-abdominal area, probably to confer a degree of rigidity when the body is lifted off the substrate by the paired appendages (Shubin et al. Reference Shubin, Daeschler and Jenkins2006).
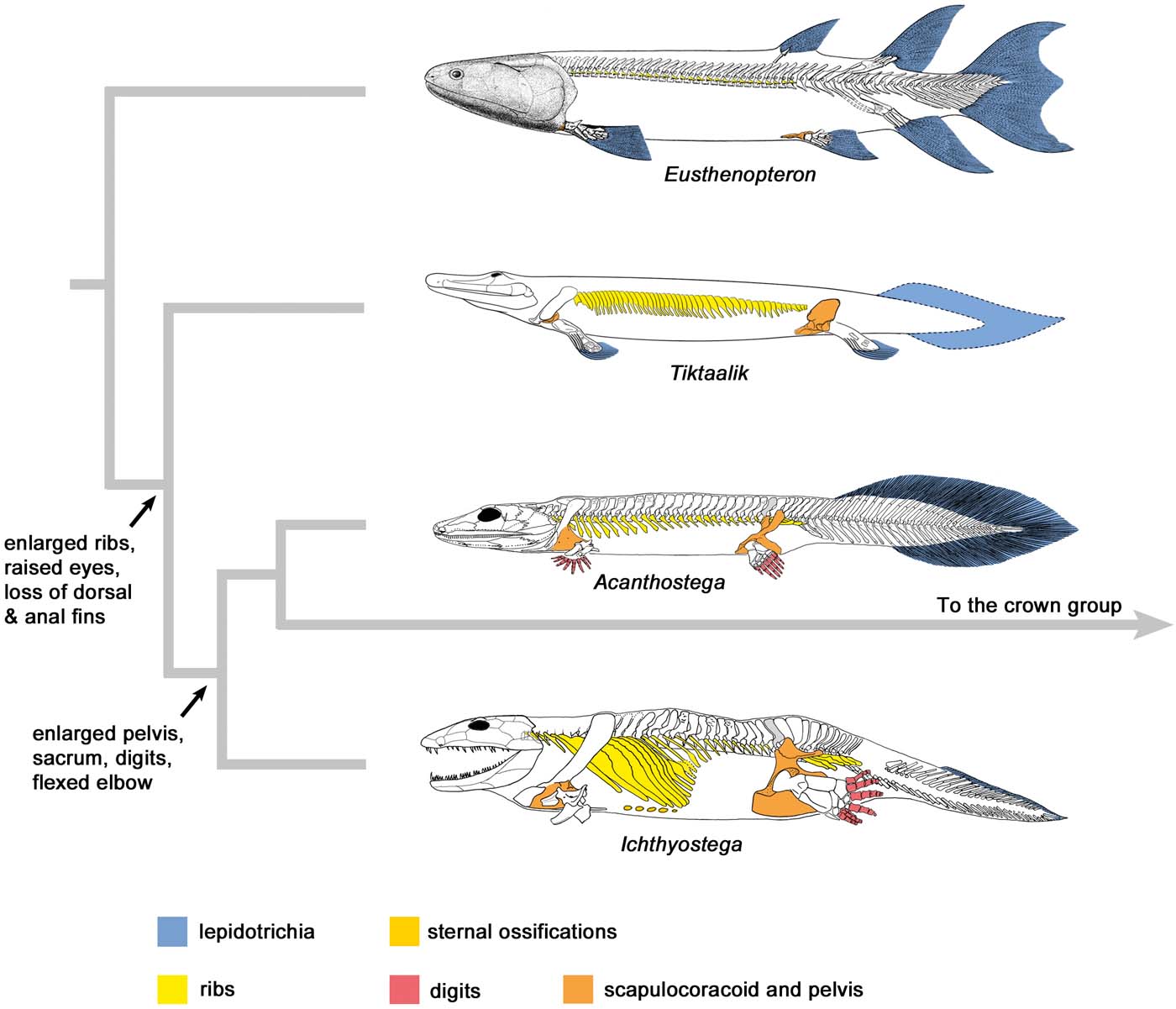
Figure 1 Simplified phylogeny spanning the fish–tetrapod transition, illustrating the major anatomical changes. Note the enormous enlargement of the ribcage and pelvis. Ribs are present in Eusthenopteron, but so small that they are difficult to see. The taxa are arranged from most aquatically adapted at the top to most terrestrially adapted at the bottom. In the colour coding, blue denotes an aquatic adaptation, and yellow to red colours represent adaptations for walking and weight support. The placement of Acanthostega crownward to Ichthyostega reflects its possession of a deltopectoral crest (see Fig. 2). Eusthenopteron modified from Jarvik (Reference Jarvik1980), Tiktaalik from Shubin et al. (Reference Shubin, Daeschler and Jenkins2014), Acanthostega and Ichthyostega from Ahlberg et al. (Reference Ahlberg, Clack and Blom2005a, Reference Ahlberg, Friedman and Blomb).
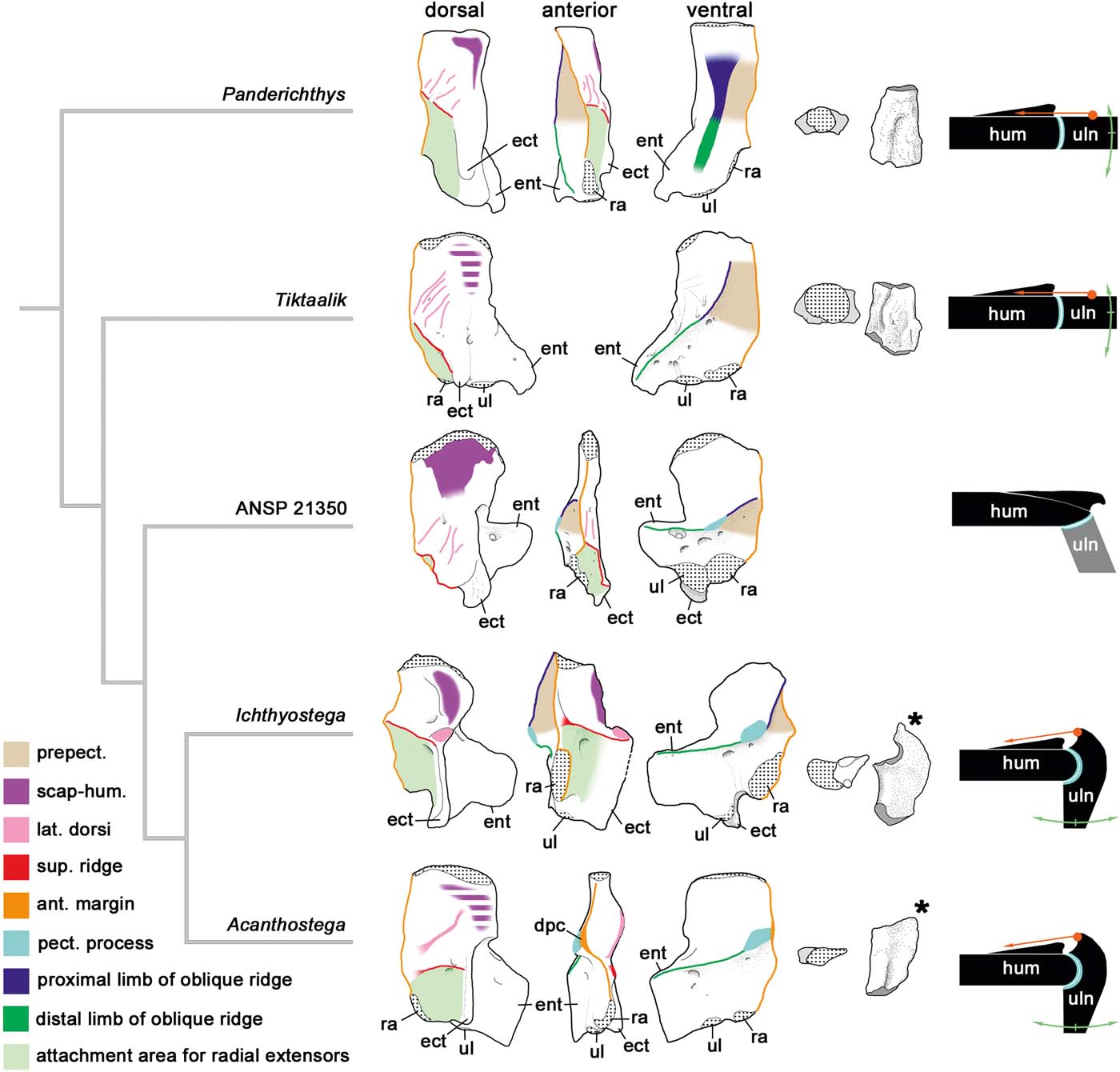
Figure 2 Origin of the flexed elbow. On the left, humeri of a range of elpistostegids and tetrapods in dorsal, anterior and ventral views, with muscle attachment processes colour-coded. In the middle, ulnae of the same taxa in proximal and dorsal views (no ulna is associated with ANSP 21350). Asterisks indicate the olecranon processes of Ichthyostega and Acanthostega. On the right, schematic models of their elbows. In these models an orange dot+arrow indicates the probable insertion and direction of the triceps brachii muscle, and green curved arrows represent the approximate range of movement of the ulna. Middle and left-hand parts modified from Ahlberg (Reference Ahlberg2011). Abbreviations: ect = ectepicondyle; ent = entepicondyle; ra = radial facet; ul = ulnar facet; hum = humerus; uln = ulna; lat. dorsi = latissimus dorsiu attachment; prepect. = prepectoral space; scap-hum. = scapulo-humeral muscle attachment; ant. margin = anterior margin of humerus; pect. process = pectoral process; sup. ridge = supinator ridge; dpc = deltopectoral crest.

Figure 3 Pelvic morphology of an elpistostegid and three early tetrapods, lateral view, anterior to the left. Not to scale. Tiktaalik modified from Shubin et al. (Reference Shubin, Daeschler and Jenkins2014); Ichthyostega, new reconstruction, based on data from Jarvik (Reference Jarvik1996); Acanthostega, new reconstruction, based on data from Coates (Reference Coates1996); Eryops modified from Pawley & Warren (Reference Pawley and Warren2006).
In contrast to these adaptations, elpistostegids retain some ‘fish' characteristics that are lost in tetrapods and point to a habitually aquatic life. Most importantly, the paired fins retain complete lepidotrichial fin webs of conventional lobe-finned fish appearance (Fig. 1). In Panderichthys, where these are perfectly preserved, the distal parts of the lepidotrichia are close-spaced and finely jointed (Vorobyeva Reference Vorobyeva2000). It has been claimed that the lepidotrichia of Tiktaalik are reduced in length (Shubin et al. Reference Shubin, Daeschler and Jenkins2006), but the published figures show that they are incompletely preserved distally; when complete, they were probably little different from those of Panderichthys. These delicate fin webs are clearly optimised for swimming in the water column, not substrate locomotion, and it is also significant in this context that the pectoral fin endoskeleton has a straight elbow (Fig. 2). The unusually robust and blade-like radius, present in both Panderichthys and Tiktaalik (Shubin et al. Reference Shubin, Daeschler and Jenkins2006; Boisvert et al. Reference Boisvert, Mark-Kurik and Ahlberg2008), may have acted as a protective guard for the more vulnerable posterodistal parts of the pectoral fin during ‘walking'. Overall, the condition of the paired fins suggests that swimming movement in the water column was still of substantial importance for elpistostegids.
With regard to both the construction of the lower jaw and the layout of the dentition, elpistostegids are essentially unmodified lobe-finned fishes (Ahlberg & Clack Reference Ahlberg and Johanson1998; Daeschler et al. Reference Daeschler, Shubin and Jenkins2006). By far the largest teeth are the paired fangs on the coronoids and anterior end of the dentary in the lower jaw, and on the ectopterygoids, dermopalatines and vomers in the upper jaw. Upper- and lower-jaw dentitions are very similar. In Devonian lobe-finned fishes, jaws of this type are associated with complete bony gill covers (Jarvik Reference Jarvik1972, Reference Jarvik1980; Johanson & Ahlberg Reference Johanson and Ahlberg1997) and prey capture was almost certainly based on suction (Anderson et al. Reference Anderson, Friedman and Ruta2013). Among the elpistostegids, Panderichthys has a complete gill cover, whereas Tiktaalik has lost the opercular and subopercular bones but retains large gular plates (Vorobyeva & Schultze Reference Vorobyeva, Schultze, Schultze and Treub1991; Downs et al. Reference Downs, Daeschler, Jenkins and Shubin2008). This suggests that suction continued to play an important part in their feeding.
The overall impression given by the character complement is that these large predators (Tiktaalik may have reached 2.5m in length) were habitually aquatic but made extensive use of substrate locomotion, sometimes in water shallow enough to require a measure of body weight support – probably meaning 30cm deep or less, considering the size of the animal. They frequently raised their eyes above the surface of the water. The elongated, diphycercal caudal fin suggests an anguilliform mode of slow tail-propelled swimming, not dissimilar to that seen today in eels and benthic sharks (Gillis Reference Gillis1996). Close comparison of Panderichthys and Tiktaalik furnishes evidence of small but interesting differences in their modes of substrate locomotion (Boisvert Reference Boisvert2005; Shubin et al. Reference Shubin, Daeschler and Jenkins2006, Reference Shubin, Daeschler and Jenkins2014; Boisvert et al. Reference Boisvert, Mark-Kurik and Ahlberg2008). The pectoral fin skeleton of Panderichthys is more limb-like than that of Tiktaalik, with a long ulna and short ulnare, contrasting with the similar-sized ulna and ulnare of Tiktaalik; in life, the pectoral fin lobe of Panderichtys presumably had a more definite demarcation between ‘forearm' and ‘wrist'. By contrast, Tiktaalik has an enlarged pelvis (Figs 1, 3), albeit still without a sacrum, whereas in Panderichthys the pelvis and pelvic fins are both small and apparently of limited mobility. Vorobyeva & Kuznetsov (Reference Vorobyeva, Kuznetsov and Mark-Kurik1992) interpreted the substrate locomotion of Panderichthys as tripodal and mudskipper-like, with the body supported by the pectoral fins anteriorly and the tail posteriorly, an interpretation that still appears valid in the light of more recent data. Tiktaalik, on the other hand, may have had a quadrupedal movement pattern where the pelvic fins played a greater part.
The difficulty comes when trying to narrow this ‘envelope of possibility' down to a specific lifestyle. The Fram Formation of Ellesmere Island, which yields Tiktaalik, consists of river and floodplain sediments (Daeschler et al. Reference Daeschler, Shubin and Jenkins2006). The Gauja and Lode Formations of Latvia, which contain Panderichthys and Livoniana, form part of the long-lived and very large delta complex known as the Main Devonian Field (Luksevics Reference Luksevics2001) – specifically, the middle to proximal region of the late Givetian incarnation of this delta (Pontén & Plink-Björklund Reference Pontén and Plink-Björklund2007). Psammosteid heterostracans, large filter-feeding, jawless vertebrates that occur abundantly in the Eifelian to Frasnian Formations of the Main Devonian Field where they appear to be inhabitants of marine-influenced environments (Obruchev & Mark-Kurik Reference Obruchev and Mark-Kurik1965; Blieck Reference Blieck1985; Schultze & Cloutier Reference Schultze, Cloutier, Schultze and Cloutier1996), are present in the Fram Formation (Kiaer Reference Kiaer1915; Tarlo Reference Tarlo1965). Psammosteids are also associated with the poorly known Parapanderichthys stolbovi (Vorobyeva Reference Vorobyeva, Kuznetsov and Mark-Kurik1992) from the early Frasnian of Stolbovo on the River Syas in Leningrad Oblast, on the eastern edge of the Main Devonian Field (Luksevics Reference Luksevics2001). Finally, the Elpistostege-yielding Escuminac Formation of Miguasha, Québec, is interpreted as estuarine (Hesse & Sawh Reference Hesse and Sawh1992; Cloutier Reference Cloutier2013). It thus appears that all known elpistostegids inhabited the lower reaches of tropical river systems and/or their deltas and estuaries.
But why were they apparently taking an interest in the world above the surface? It seems unlikely that they were looking out for danger, given that they themselves were formidable predators. Terrestrial prey seems a more plausible object of interest. Large terrestrial arthropods, both arthropleurids and scorpions, had already evolved by the Early Devonian (Størmer 1976; Shear et al. Reference Shear, Gensel and Jeram1996) and were probably far more abundant than their scarce fossil remains suggest. They could plausibly be taken from the water's edge by a large predator adapted to operating in the shallows and supporting its body out of water for short periods. The retention of an essentially fish-like, suction-adapted jaw apparatus might seem to argue against this hypothesis, but the functional flexibility of such a mouth should not be underestimated. In a startling modern-day example, European catfish (Silurus glanis) in the Tarn River in France have learned how to catch pigeons that come down to drink, and now engage habitually in this feeding behaviour (Cucherousset et al. Reference Cucherousset, Boulêtreau, Azémar, Compin, Guillaume and Santoul2012). Silurus glanis is similar to an elpistostegid in size, and is a slow anguilliform swimmer, but its head is extremely short-snouted and its mouth is wide and transverse; it should thus, if anything, be less well-adapted to snatching prey from the bank than an elpistostegid.
Another possibility, in a tidal environment, is scavenging on dead and moribund fishes stranded at low tide (Vorobyeva & Kuznetsov Reference Vorobyeva, Kuznetsov and Mark-Kurik1992). The idea of elpistostegids as tidal specialists, using their substrate-locomoting and weight-supporting abilities to remain active during low tide, is attractive but untestable and, in any case, probably not applicable to Tiktaalik. What is clear, however, is that elpistostegids were very different from conventional tetrapodomorph fishes, even from superficially similar forms such as Mandageria; they had made a decisive shift away from a weightless life in the water column towards substrate locomotion, weight support and interaction with the terrestrial environment (Fig. 1).
4. Tetrapods: morphology and function
The earliest tetrapods retained certain components of the elpistostegid body plan, such as the vaguely crocodile-like shape with raised eyes and a low elongate caudal fin, but coupled these with a number of innovations that appear to be adaptations for better weight support, more efficient substrate locomotion, improved aerial vision and possibly a shift from sucking to snapping prey capture (Fig. 1). The main structural novelties are the digits (accompanied by the loss of lepidotrichia on the paired appendages), the flexed elbow (Fig. 2), the enlarged hind limb, the ischium and sacrum (Fig. 3) and the (incipient) zygapophyses. In addition, the scapulocoracoid is enlarged, the glenoid and acetabulum rotated laterally and the eyes proportionately bigger compared to elpistostegids (Clack Reference Clack2012; MacIver et al. Reference MacIver, Schmitz, Mugan, Murphey and Mobley2017). On the head, it is noticeable that the lateral-line canals are well developed on the lower jaw, cheeks and snout (Lebedev & Clack Reference Lebedev and Clack1993; Jarvik Reference Jarvik1996; Ahlberg & Clack Reference Ahlberg and Clack1998), but tend to fade out on the skull table (Clack Reference Clack2002a, Reference Clackb; Ahlberg et al. Reference Ahlberg, Clack, Luksevics, Blom and Zupins2008). This supports the inference that the eyes were habitually held above the surface (MacIver et al. Reference MacIver, Schmitz, Mugan, Murphey and Mobley2017). The bony gill cover has been lost in its entirety, and the lower jaw substantially reconfigured as regards both construction and dentition (Ahlberg & Clack Reference Ahlberg and Clack1998). The anterior part of the jaw is proportionately more slender than in lobe-finned fishes and elpistostegids; the difference is obvious to the eye and can also be discerned in the morphometric analysis of Anderson et al. (Reference Anderson, Friedman and Ruta2013, fig. 2a). The coronoids have become slender and the coronoid fangs reduced in size or lost altogether, whereas the marginal teeth have become much larger. This enlargement of the marginal dentition is also seen in the upper jaw, but the paired fangs of the inner palatal arcade are retained, thus creating an asymmetry between upper and lower dentitions.
Before examining these morphological traits in detail, we must consider the Devonian tetrapod trackway record and its reliability (Fig. 4). Setting aside some isolated ‘footprints' of disputed identity, such as ‘Notopus petri' isp. from the Emsian of Brazil (Leonardi Reference Leonardi1983), which has been reinterpreted as a possible starfish resting trace (Rocek & Rage Reference Rocek and Rage1994), the track sites can be listed in stratigraphic order as follows. Eifelian: Zachełmie Quarry, Holy Cross Mountains, Poland (Niedzwiedzki et al. Reference Niedzwiedzki, Szrek, Narkiewicz, Narkiewicz and Ahlberg2010; Qvarnström et al. Reference Qvarnström, Szrek, Ahlberg and Niedzwiedzki2018); Givetian: Valentia Slate, Valentia Island, Ireland (Stössel Reference Stössel1995; Stössel et al. Reference Stössel, Williams and Higgs2016); Late Devonian, probably Frasnian: Tarbat Ness, Scotland (Rogers Reference Rogers1990; Marshall et al. Reference Marshall, Rogers and Whiteley1996); Late Devonian, probably Famennian: Genoa River, Victoria, Australia (Warren & Wakefield Reference Warren and Wakefield1972). All these localities contain multiple footprints arranged in regular trackways (Fig. 4), some with body drags and some without. The footprints have consistent well-constrained morphologies, typically oval and quite deep (but see below (this section) for further discussion of the Zachełmie footprints); digit impressions are present in prints from Zachełmie (Niedzwiedzki et al. Reference Niedzwiedzki, Szrek, Narkiewicz, Narkiewicz and Ahlberg2010) and Genoa River (Warren & Wakefield Reference Warren and Wakefield1972; Clack Reference Clack1997). Two putative tetrapod trackways from the Silurian or earliest Devonian of Australia, respectively from Kalbarri in Western Australia (McNamara Reference McNamara2014) and Glenisla farmstead in Victoria (Warren et al. Reference Warren, Jupp and Bolton1986; Clack Reference Clack1997), will not be considered further here, as I am not convinced that they show diagnostic tetrapod characteristics. Nevertheless, they are both highly interesting ichnofossils deserving of further study.

Figure 4 Devonian tetrapod track sites. Zachełmie photos by Grzegorz Niedzwiedzki, reproduced with permission. Valentia Island photo from Stössel et al. (Reference Stössel, Williams and Higgs2016). Tarbat Ness photo from Rogers (Reference Rogers1990). Genoa River photo from Warren & Wakefield (Reference Warren and Wakefield1972). Scale bars = 50cm (Zachełmie and Valentia Island trackways); 10cm (Zachełmie footprint and Tarbat Ness and Genoa River trackways).
As already mentioned, the publication of the Zachełmie tracks provoked some negative responses, directed partly at this discovery and partly at the Devonian trackway record in general. All these responses asserted that tracks like those attributed to tetrapods could, in fact, by some means or another, be generated by fishes. However, none of these claims stands up to scrutiny.
King et al. (Reference King, Shubin, Coates and Hale2011) recorded underwater walking by a small individual of the African lungfish Protopterus moving over a sheet of Perspex, noting the contact points between its fins and the substrate. The movement pattern resembles a tetrapod trackway, but the apparent morphological similarity between the points of substrate contact and actual tetrapod footprints simply reflects the method of recording and would not be duplicated in an actual sediment trace left by such long, threadlike fins. The authors' statement that the body was lifted off the substrate by the fins in a tetrapod-like manner is contradicted by the film clips published with the paper, which show that the bottom of the tank is in constant contact with the throat region and near-constant contact with the tail of the fish during the locomotory cycle; the pelvic region is lifted up, but a trace fossil produced by a fish moving in this way would, nevertheless, contain a continuous body drag. It should also be noted that Protopterus has an extremely derived paired fin morphology, different from Devonian stem lungfishes (Ahlberg & Trewin Reference Ahlberg and Trewin1995), and even more different from tetrapodomorph fishes (Jarvik Reference Jarvik1948, Reference Jarvik1980), suggesting that it may not be very relevant for the interpretation of Devonian ichnofossils.
Falkingham & Horner (Reference Falkingham and Horner2016), working with a different setup where a Protopterus was moving across aerially exposed mud, recorded a curious form of locomotion where the lungfish repeatedly bit into the sediment with its mouth and used this as a pivot to lever its body forward. The authors claimed that the resulting traces closely resemble some of the supposed Devonian tetrapod tracks, but despite backing up their argument with a somewhat tendentious figure (where a very short section of one of the Valentia Slate trackways is compared with their lungfish bite traces without acknowledging that the former is tectonically deformed, a critically important point in this context, which Stössel (Reference Stössel1995) explained in some detail in his original description), it is clear from even a cursory inspection that this is incorrect: the lungfish crawling trace completely fails to capture the regular pattern, footprint morphology and digit impressions of the Devonian tracks. Actual lungfish mouth impressions, in the form of feeding traces, have recently been discovered in the Early Devonian of Poland (Szrek et al. Reference Szrek, Salwa, Niedzwiedzki, Dec, Ahlberg and Uchman2016) and do not look anything like tetrapod tracks.
Lucas (Reference Lucas2015) acknowledged the tetrapod identity and lateral-sequence-walk pattern of some Devonian trackways, including those from Valentia Island, but claimed that the Zachełmie traces – which the author had not, to my knowledge, examined in person – could have been formed by a combination of different kinds of fish activity, including feeding traces and coprolite deposits. In fact, the mechanisms proposed by Lucas (Reference Lucas2015) would not be adequate to explain the characteristics of the Zachełmie ichnofossils, which include deep and morphologically consistent digit impressions as well as obvious trackways (Niedzwiedzki et al. Reference Niedzwiedzki, Szrek, Narkiewicz, Narkiewicz and Ahlberg2010). A forthcoming detailed description of the Zachełmie track assemblage will address these issues. Suffice it to say for now that the tetrapod identity of the Zachełmie, Valentia Island, Tarbat Ness and Genoa River tracks is upheld; the separate question raised by Pierce et al. (Reference Pierce, Clack and Hutchinson2012), whether they are compatible with the skeletal morphology of known Devonian tetrapods and, more especially, with Ichthyostega, is considered later in this section.
So, what do the Devonian tetrapod tracks actually tell us? In addition to information about the living environment and locomotory behaviour, they provide some intriguing glimpses of the soft anatomy of the appendages. Devonian tetrapod feet are usually reconstructed as webbed, with relatively long and slender digits (e.g., Clack Reference Clack2005), but comparative anatomy and the footprint record both suggest that this is incorrect. The elements that tend to be interpreted in the life reconstructions as the most proximal phalanges of the digits are, in fact, the metacarpals/tarsals; a comparison with extant tetrapods indicates that these elements should be embedded in the palm/sole, the edge of which should run across the proximal ends of the first phalanges proper. The toes should thus be shorter than in these life reconstructions. The Zachełmie footprints show short, triangular toes with no claws, no webbing and no separate phalangeal pads (Fig. 4). The shape match to the hind foot of Ichthyostega, if the latter is reconstructed with an anatomically correct sole extending onto the bases of the first phalanges, is strikingly close (Niedzwiedzki et al. Reference Niedzwiedzki, Szrek, Narkiewicz, Narkiewicz and Ahlberg2010). In footprints that appear to have been made by the foot entering the sediment at an angle, the toe impressions are short, rounded ‘dimples' in the distal margin; this matches the condition in the Late Devonian Genoa River footprints from Australia (Warren & Wakefield Reference Warren and Wakefield1972; Clack Reference Clack1997). Some of the Zachełmie footprints have long sole impressions that appear to represent not only the sole of the foot proper but also the flexor surface of the lower leg (Niedzwiedzki et al. Reference Niedzwiedzki, Szrek, Narkiewicz, Narkiewicz and Ahlberg2010). This is consonant with the suggestion from the body fossil record that the earliest tetrapods, as represented by Ichthyostega and Acanthostega, had flat ankles of limited flexibility (Coates Reference Coates1996).
The importance of these features is that they do not look like adaptations for paddling (which benefits from webbing) or clambering through vegetation (which requires flexible digits that can curl and grasp) but for walking substrate locomotion, with the short, stiff, pointed toes providing purchase on the sediment. This conclusion is reinforced by the presence of well-defined sole-pad impressions in some of the Zachełmie footprints (Niedzwiedzki et al. Reference Niedzwiedzki, Szrek, Narkiewicz, Narkiewicz and Ahlberg2010). The replacement of the paired fin webs of elpistostegids by feet like this implies the enhancement of walking ability at the expense of swimming ability.
A similar argument can be made for the flexed elbow (Fig. 2). With a single probable exception (see below), all known tetrapod elbows, other than those of extremely derived secondarily aquatic forms such as whales and ichthyosaurs, have a flexed morphology with the main extensor musculature (the triceps brachii) attaching to an olecranon process located proximally on the postaxial margin of the ulna. Such an elbow is capable of considerable flexion but cannot be hyperextended past the straight position where the ulna lies in line with the humerus. By contrast, Tiktaalik and Panderichthys, as well as less crownward tetrapodomorph fishes, have a straight elbow without an olecranon process (Andrews & Westoll Reference Andrews and Westoll1970; Shubin et al. Reference Shubin, Daeschler and Jenkins2006; Boisvert et al. Reference Boisvert, Mark-Kurik and Ahlberg2008; Ahlberg Reference Ahlberg, Beznosov, Luksevics and Clack2011), with a much smaller range of flexion but probably some capacity for hyperextension (Fig. 2). Ichthyostega has long been recognised as having a permanently flexed elbow (Jarvik Reference Jarvik1952, Reference Jarvik1980, Reference Jarvik1996), with the radius articulating ventrally on the humerus in a position that precludes full straightening of the joint. Nevertheless, the presence of a strongly developed olecranon process indicates that the elbow was capable of a powerful, if not necessarily very long, extension movement, which must have formed an important part of its locomotory cycle (Pierce et al. Reference Pierce, Clack and Hutchinson2012). Acanthostega was described by Coates (Reference Coates1996) as having a primitive elbow without an olecranon process. This became a key component of the interpretation of this genus as a very primitive, and primitively aquatic, tetrapod that had acquired digits in advance of significant weight-bearing adaptations (Janis & Farmer Reference Janis and Farmer1999; Carroll & Holmes Reference Carroll, Holmes and Hall2007; Coates & Ruta Reference Coates, Ruta and Hall2007). However, the elbow of Acanthostega is, in fact, of the characteristic tetrapod type with an olecranon process, albeit small and poorly ossified (Ahlberg Reference Ahlberg2011); the elpistostegid elbow, which really lacks an olecranon, is quite different (Fig. 2).
The flexed elbow of tetrapods has three positive functional effects and one negative. On the positive side, it lowers the foot relative to the shoulder, enhances the weight-bearing role of the forearm and increases forelimb stride length while keeping the foot close to the body. These effects all serve to enhance walking ability. On the negative side, it makes the forelimb less streamlined and probably compromises the ability of the distal part to serve as an effective control surface during movement in the water column. Thus, as with the replacement of the fin web by digits, walking ability is enhanced at the expense of swimming ability.
The one apparent exception to this type of elbow among early tetrapods is the late Famennian tetrapod humerus ANSP (Academy of Natural Sciences, Philadelphia) 21350 from the Catskill Formation (Shubin et al. Reference Shubin, Daeschler and Coates2004; Ahlberg Reference Ahlberg2011). The ulna is unfortunately unknown, but the distal end of the humerus is drastically different from other known tetrapods as well as elpistostegids (Fig. 2). In these animals the ulnar articulation is always distal on the humerus. The change from the straight elbow of elpistostegids to the flexed elbow of Ichthyostega and Acanthostega (and later tetrapods) is achieved entirely by remodelling the ulna, changing the orientation of its proximal articulation and creating an olecranon process to carry the extensor musculature. In ANSP 21350, on the other hand, the whole distal end of the humerus has rotated so that the radial and ulnar facets (which are flat and confluent) face ventrally; structures on the dorsal surface of the humerus, such as the supinator ridge, have shifted distally (Ahlberg Reference Ahlberg2011). The effect is to create a flexed elbow by different means, probably without the development of an olecranon process (Fig. 2).
This independent invention of a flexed elbow is interesting from several angles. Setting aside the autapomorphic distal end, the general morphology of ANSP 21350 is very primitive and positions it as the phylogenetically least crownward tetrapod humerus (Shubin et al. Reference Shubin, Daeschler and Coates2004; Ahlberg Reference Ahlberg2011). The elbow appears to have had limited mobility, judging by the flatness of the articular surfaces, and will thus have enhanced the weight-bearing and body-raising capacity of the forelimb but not its stride length. It appears that there was significant selective pressure towards a weight-bearing forelimb at a very early stage of tetrapod evolution, leading to the evolution of (at least) two separate solutions to the problem.
The tetrapod hind limb also furnishes evidence of the enhancement of walking ability and weight support at the expense of swimming ability. In addition to the replacement of the fin web by digits and the enlargement of the entire appendage, the major changes here are the reorientation of the acetabulum from posterior to lateral, the emergence of the ischium and the creation of a sacral attachment by means of a modified rib that articulates with the ilium (Figs 1, 3). The first two have the effect of turning the pelvic appendage into a laterally projecting structure with a propulsive power stroke. Interestingly, neither feature is present in Tiktaalik, even though the pelvis is enlarged relative to Panderichthys or tetrapodomorph fishes (Shubin et al. Reference Shubin, Daeschler and Jenkins2014). The typical shape of a tetrapodomorph fish pelvic fin is a small, posterolaterally projecting hydrofoil that presumably had a minor steering role during tail-propelled swimming. A large, laterally projecting limb with digits would cause greater drag and would probably have compromised the tail-propelled swimming somewhat, though it could of course have been used for paddling locomotion.
Early tetrapods typically have proportionately longer tails than elpistostegids and lobe-finned fishes (Fig. 1), and both Acanthostega and Ichthyostega show skeletal modifications of the tail base (enlarged post-sacral ribs, in Ichthyostega also large fan-shaped neural arches) that suggest enlarged attachments for the axial musculature and perhaps a decoupling of tail undulation from the presacral trunk (Coates Reference Coates1996; Ahlberg et al. Reference Ahlberg, Clack and Blom2005a, Reference Ahlberg, Friedman and Blomb). Gray (Reference Gray1968) argued that the long tail of tetrapods also serves to balance the weight load on the pelvis, by acting as a counterweight to the presacral trunk. However, while this applies in a terrestrial setting, the initial driving force for evolving a long tail could simply have been the functional decoupling of tail undulation from hindlimb movement in an aquatic environment.
The sacral attachment, by contrast, is a ‘smoking gun' for weight support. Some modern fishes use quadrupedal action of the paired fins for swimming (Latimeria, Neoceratodus) or underwater walking (the epaulette shark, Hemiscyllium), but none has evolved the equivalent of a sacrum. The only sacrum analogue in a modern fish is found in a small cave-dwelling teleost from Thailand, Cryptotora thamicola, which uses its paired fins in a tetrapod-like manner to climb up steep rock surfaces in fast-flowing subterranean rapids and waterfalls (Flammang et al. Reference Flammang, Suvarnaraksha, Markiewicz and Soares2016). The pseudo-sacrum here is clearly an adaptation to counteract the force of the flowing water.
In short, the evolutionary modifications that turned the paired appendages from elpistostegid paired fins into tetrapod limbs all carry a strong, consistent, unambiguous signal of a functional shift from swimming to walking and weight support. This equally applies to the evolution of zygapophyses in the vertebral column (Pierce et al. Reference Pierce, Ahlberg, Hutchinson, Molnar, Sanchez, Tafforeau and Clack2013). It is also worth noting that the modification of the squamation from a whole-body covering still present in Panderichthys, Tiktaalik and probably Elpistostege (Vorobyeva Reference Vorobyeva1980; Schultze & Arsenault Reference Schultze and Arsenault1985; Vorobyeva & Schultze Reference Vorobyeva, Schultze, Schultze and Treub1991; Daeschler et al. Reference Daeschler, Shubin and Jenkins2006; Matton & Lemieux Reference Matton and Lemieux2013) to a distinct belly armour composed of gastralia occurred at this time, Acanthostega furnishing the earliest known example of such a belly armour (Coates Reference Coates1996). A final intriguing though indirect piece of evidence is provided by the Australian Early Carboniferous (Viséan) tetrapod Ossinodus (Bishop et al. Reference Bishop, Walmsley, Phillips, Quayle, Boisvert and McHenry2015). Phylogenetically, Ossinodus is consistently recovered as a Whatcheriid-grade tetrapod, immediately crownward to the Devonian taxa discussed here (Warren & Turner Reference Warren and Turner2004; Warren 2007); its postcranial morphology is slightly more derived, but there are no fundamental differences relative to the Devonian forms. A radius of Ossinodus carries a large proximal callus that has been investigated with μCT (micro computed tomography) and found to represent a healed fracture, almost certainly caused by the animal falling from a height and landing badly on its front leg (Bishop et al. Reference Bishop, Walmsley, Phillips, Quayle, Boisvert and McHenry2015). This is thus direct evidence of terrestrial activity some distance from the water's edge, in a tetrapod only slightly more derived than Acanthostega.
Before going on to consider how these conclusions impact our understanding of Devonian tetrapods in general and Acanthostega in particular, we must return to the conflict between the trackway data and the analysis of limb mobility in Ichthyostega undertaken by Pierce et al. (Reference Pierce, Clack and Hutchinson2012). The tetrapod tracks from Zachełmie and Genoa River appear to have been made in shallow water, but some of the Valentia Island tracks are terrestrial, as are the Tarbat Ness tracks (Rogers Reference Rogers1990; Stössel et al. Reference Stössel, Williams and Higgs2016). The footprints are in all cases quite deeply impressed, suggesting that even in the subaquatic tracks the body was not entirely supported by the water. All three localities show quadrupedal tracks with distinct manus and pes impressions, both apparently imprinted with the sole of the foot flat to the sediment. The Valentia Island, Tarbat Ness and Genoa River tracks show lateral sequence walks, where the feet move in a diagonal pattern, left front together with right hind and so on, as in a modern salamander (Fig. 4), and one short example of this mode of walking is also preserved at Zachełmie (Niedzwiedzki et al. Reference Niedzwiedzki, Szrek, Narkiewicz, Narkiewicz and Ahlberg2010). Pierce et al. (Reference Pierce, Clack and Hutchinson2012) claimed that Ichthyostega could not place its hind foot flat on the ground, and was not capable of a lateral sequence walk, on account of the limited range of axial rotation in its limbs.
While the pelvic acetabula of Tiktaalik (Shubin et al. Reference Shubin, Daeschler and Jenkins2014) and tetrapodomorph fishes such as Eusthenopteron and Gooloogongia (Andrews & Westoll Reference Andrews and Westoll1970; Johanson & Ahlberg Reference Johanson and Ahlberg2001) are unremarkable cup-shaped structures, not too different in shape from those of many extant tetrapods, those of Devonian tetrapods are decidedly strange (Coates Reference Coates1996; Jarvik Reference Jarvik1996). In both Acanthostega and Ichthyostega the acetabulum is obliquely strap-shaped, with the narrow anteroventral part extending to the anterior extremity of the pubis; the posterior margin is marked by a distinct notch (Fig. 3). A pelvic specimen of Acanthostega preserved with the femur in articulation (Coates Reference Coates1996, fig. 19h) shows that the head of the femur fits into the posterodorsal part of the acetabulum. In Ichthyostega, this part of the acetabulum has less height than in Acanthostega, slopes more strongly and is bounded ventrally by a buttress that fits into the intertrochanteric fossa on the ventral surface of the femoral head. In the forelimb, the glenoid of the shoulder girdle is elongate with a slight spiral twist in both genera. Pierce et al. (Reference Pierce, Clack and Hutchinson2012) argued from these observations and from computer modelling experiments that the range of limb movement was limited in Ichthyostega, especially as regards axial rotation of the humerus and femur, restricting its terrestrial locomotory ability to a forelimb-propelled symmetrical ‘crutching' movement somewhat like a giant mudskipper; the hind limbs would have functioned only as paddles and would have had little or no role in terrestrial locomotion. They also noted that the acetabula and glenoids of other Devonian tetrapods, where known, are broadly similar to those of Ichthyostega and probably imply similar limitations to their locomotory abilities.
Pierce et al.'s (Reference Pierce, Ahlberg, Hutchinson, Molnar, Sanchez, Tafforeau and Clack2013) general conclusion that the limbs of Devonian tetrapods had tightly constrained movement arcs, different from both their fish predecessors and later tetrapods, is clearly supported by the morphological data. The question is whether Ichthyostega was really quite as tightly constrained as they argue, and whether this also applies to other early tetrapods. The same general form of hip- and shoulder-joint morphology in fact persisted into the tetrapod crown group. The temnospondyl Eryops, for example, has a sloping acetabulum with an anterior extension and a posterior notch, little different from that of Acanthostega (Fig. 3). We can thus probably assume on the basis of phylogenetic inference that all Devonian tetrapods conformed to this pattern, with minor variations. But as the footprint data show, some of them were perfectly capable of performing lateral sequence walks with the hind feet flat on the ground and the toes facing laterally (Warren & Wakefield Reference Warren and Wakefield1972; Clack Reference Clack1997). The simplest explanation is that these track makers had a degree of shoulder and hip rotation somewhat greater than that reconstructed for Ichthyostega, within the limits of an essentially similar joint morphology, and that this sufficed to allow lateral sequence walking. Bilaterally symmetrical tracks, more similar to the movement pattern of Ichthyostega inferred by Pierce et al. (Reference Pierce, Ahlberg, Hutchinson, Molnar, Sanchez, Tafforeau and Clack2013), are present at Zachełmie along with a single lateral sequence track (Niedzwiedzki et al. Reference Niedzwiedzki, Szrek, Narkiewicz, Narkiewicz and Ahlberg2010). The locomotory capabilities of Ichthyostega itself can potentially be tested in the future, following the recent discovery of tetrapod trace fossils from the Famennian of East Greenland (G. Niedzwiedzki, H. Blom & B. Kear, pers. comm. 2016).
The evidence from body fossils and trackways points unambiguously to a much greater degree of engagement with the terrestrial environment in Devonian tetrapods than in elpistostegids. The tetrapod body has been systematically reconfigured for improved substrate locomotion and weight support, with the hind limbs in particular acquiring a greater propulsive and supporting role. Arguments that these structural changes occurred in order to facilitate paddling locomotion in water (Pierce et al. Reference Pierce, Clack and Hutchinson2012) fail to explain what would drive the initial selection pressure away from fins that were already optimised for swimming, and likewise fail to explain the origin of the sacrum, a weight-supporting structure that is unnecessary for aquatic locomotion. In the case of Ichthyostega, the skeleton also manifests other terrestrial adaptations such as a large ribcage, a reduced tail fin and proportionately large limb girdles (Fig. 1). The terrestrial adaptations effectively define one edge of the ‘envelope of possibility' of Devonian tetrapod lifestyles. The other edge of the envelope is defined by the aquatic adaptations that these tetrapods retain. The analytically most important of these adaptations, because it is the most widely known, is the persistence of a well-developed lateral-line system in all known Devonian tetrapods (Lebedev & Clack Reference Lebedev and Clack1993; Jarvik Reference Jarvik1996; Ahlberg & Clack Reference Ahlberg and Johanson1998; Clack Reference Clack2002a, Reference Clackb; Ahlberg et al. Reference Ahlberg, Clack, Luksevics, Blom and Zupins2008). A lateral-line canal system is not only useless in air but a positive liability that will be damaged by desiccation unless the skin is kept moist. The lepidotrichial tail fin has similar significance, but is only known with certainty in Acanthostega and Ichthyostega (Jarvik Reference Jarvik1952, Reference Jarvik1996; Coates Reference Coates1996), although probable tetrapod lepidotrichia are also associated with Ventastega (Ahlberg et al. Reference Ahlberg, Clack, Luksevics, Blom and Zupins2008). The lateral-line canal system persists into the tetrapod crown group (Carroll Reference Carroll1988, Reference Carroll2009); a single tantalising specimen of a Carboniferous embolomere tail with supraneural radials and scattered lepidotrichia hints that this may also be true for the tail fin (Clack Reference Clack2011).
Thus, we can conclude that Devonian tetrapods, and many post-Devonian forms, remained firmly tied to the water even as they expanded their sphere of operations on land. They must have spent a substantial proportion of every day with the face and body submerged, but probably with the eyes above water and the skull table just awash for much of the time. Ossinodus exemplifies this ‘envelope of possibility' and provides a momentary glimpse of the actual lifestyle contained within it: it has well-developed lateral-line canals that it must have kept immersed in water for many hours each day, but on one occasion it left the water and climbed high enough (perhaps up a river bank, or a sloping fallen tree trunk?) to fracture its radius when it lost its footing and fell (Bishop et al. Reference Bishop, Walmsley, Phillips, Quayle, Boisvert and McHenry2015). It nevertheless managed to make it back to the water and eventually made a full recovery, as shown by the healed state of the fracture.
The final part of the lifestyle puzzle to consider is feeding. Here, the evidence is ambiguous. Most Devonian tetrapods have dentitions of sharp, conical, gently recurved teeth that look like those of present-day piscivores (Ahlberg & Clack Reference Ahlberg and Johanson1998). The exception is Ichthyostega, where the marginal teeth on the maxilla are sharply recurved and somewhat blade-like (Jarvik Reference Jarvik1996, pl. 7:2). One individual of Acanthostega has two fin spine fragments from a chondrichthyan or acanthodian caught in its lower jaw (see Section 5), providing unambiguous evidence of piscivory. Anderson et al. (Reference Anderson, Friedman and Ruta2013) argue, on the basis of a morphometric analysis of mandibular outlines, that feeding mechanics remained substantially unchanged across the fish–tetrapod transition, and only really began to diversify away from this ancestral condition – which they consider to be linked to suction feeding powered by the orobranchial chamber – during the early evolution of amniotes. However, this argumentation implies that the substantial changes in jaw construction and dentition seen in Devonian tetrapods, as well as the complete loss of the bony gill cover, had, at most, a marginal impact on the functional mechanics of the feeding system: an unsatisfactory conclusion that fails to explain why these changes occurred at all. I would argue that these changes do, in fact, represent the first stage of a shift away from suction feeding towards snapping. Many Devonian tetrapods were no doubt primarily fish-eaters, but as we have seen with the example of the pigeon-catching catfish, a jaw apparatus optimised for aquatic prey capture can still allow for remarkable behavioural flexibility.
5. Tetrapods: environments
To properly understand the significance of these tetrapod innovations, and to try to explain the mismatch between the footprint and body fossil records, we need to examine the living environments of the earliest tetrapods. Of course, it is impossible to achieve an overview of this topic such as you could produce for a modern-day animal group. All we have is a small number of positive statements that one or more tetrapods were present in a particular environment at a particular time. On a planetary scale, these pinpricks of light in the darkness of deep time are almost infinitesimally small. We need to keep in mind Donald Rumsfeld's unfairly derided aphorism about ‘unknown unknowns'; most of the ecological, morphological and environmental diversity of the earliest tetrapods no doubt falls into this category and might surprise us greatly if we could suddenly see it. Forced to extrapolate from our meagre store of data points, we are always in danger of joining the dots wrongly. But what do we know?
The earliest definite tetrapod fossils are the footprints from Zachełmie in Poland, an Eifelian locality dated to approximately 390 Ma (Fig. 5) (Qvarnström et al. Reference Qvarnström, Szrek, Ahlberg and Niedzwiedzki2018). The Zachełmie palaeoenvironment, initially thought to be intertidal (Niedzwiedzki et al. Reference Niedzwiedzki, Szrek, Narkiewicz, Narkiewicz and Ahlberg2010), has recently been re-examined in greater detail and reinterpreted (Qvarnström et al. Reference Qvarnström, Szrek, Ahlberg and Niedzwiedzki2018). The locality actually seems to represent a series of shallow and possibly saline ephemeral lakes on a coastal plain, which were evidently quite close to the sea as the non-marine succession is punctuated by two inwash deposits of marine microfossils and a brief episode of marine-influenced water conditions. Some small streams may have fed into the lakes, bringing the modest amount of clay mineral that is found in the sediment, but there is no evidence for any connection to a large river system. The lakes contained a depauperate invertebrate fauna, represented by a trace fossil assemblage, but there is virtually no evidence of fish apart from a single Undichna swimming trace (Qvarnström et al. Reference Qvarnström, Szrek, Ahlberg and Niedzwiedzki2018) and one fish scale (Piotr Szrek, pers. comm. 2018). Low vegetation grew around the water's edge, evidenced by soil horizons with small root casts, and large arthropods made Beaconites-type burrows in the soil. Rare halite pseudomorphs suggest hot, dry conditions and occasionally hypersaline water conditions, though it is also possible that the salt crystals formed later from brine penetrating the sediment from below (Jaworska Reference Jaworska2017). Tetrapods of varying sizes, the largest probably more than 2m in length judging by the size of the footprints (Niedzwiedzki et al. Reference Niedzwiedzki, Szrek, Narkiewicz, Narkiewicz and Ahlberg2010), were active in these lakes (Fig. 6b).

Figure 5 Timescale for the elpistostegid and tetrapod fossil record of the Middle and Late Devonian. Numbers in boxes denote ages of stage boundaries. The red line indicates the age of the earliest tetrapod footprints (left) and body fossils (right), showing the temporal mismatch between the two. The ages of Tarbat Ness and Genoa River are approximate but all others are tightly constrained. Acanthostega, Ichthyostega and Tiktaalik are reproduced from Fig. 1, Tulerpeton from Lebedev (Reference Lebedev1984), Jakubsonia from Lebedev (Reference Lebedev2004), Elginerpeton from Ahlberg et al. (Reference Ahlberg, Clack and Blom2005a, Reference Ahlberg, Friedman and Blomb), Zachelmie tracks from Niedzwiedzki et al. (Reference Niedzwiedzki, Szrek, Narkiewicz, Narkiewicz and Ahlberg2010), Valentia Island tracks from Stössel et al. (Reference Stössel, Williams and Higgs2016), Tarbat Ness tracks from Rogers (Reference Rogers1990) and Genoa River tracks from Warren & Wakefield (Reference Warren and Wakefield1972). Panderichthys is represented by a photo of a reconstruction model produced by Esben Horn in collaboration with the author; reproduced with permission. Because of space limitations, not all body fossil taxa are shown. Livoniana is contemporary with Panderichthys; Parapanderichthys with Tiktaalik and Elpistostege; Obruchevichthys and Webererpeton with Elginerpeton; and Densignathus, Hynerpeton, ANSP 21350 and Ventastega with Acanthostega and Ichthyostega.

Figure 6 Block diagrams of idealised Devonian landscapes showing the distribution of elpistostegids and tetrapods inferred from fossil locality data. (a) Guide diagram showing the following features: 1. delta of permanent river (e.g., Gauja Formation), 2. floodplain of permanent river with avulsion ponds (e.g., Fram Formation, Catskill Formation), 3. short-lived lake on coastal plain (e.g., Zachełmie), 4. permanent river that breaks up into ephemeral channels (e.g., Valentia Slate, possibly Britta Dal Formation), 5. inland sabkha and dune fields (e.g., Tarbat Ness). (b) Middle Devonian to early Frasnian: elpistostegids occupy the lower parts of permanent river systems whereas tetrapods are present in the upper parts of river basins, in sabkha environments and in coastal lakes. The uppermost elpistostegid represents Tiktaalik, the lower two represent Panderichthys and Elpistostege. The tetrapods represent, from top to bottom, Valentia Island, Tarbat Ness and Zachełmie. (c) Late Frasnian to Famennian: the elpistostegids have disappeared and all the environments are occupied by tetrapods. The tetrapods represent, from top to bottom, the Britta Dal Formation, Genoa River, the Catskill Formation, the Sosnogorsk Formation (and possibly Andreyevka-2) and the tetrapod localities in the Main Devonian Field (Velna Ala, Pavari, Ketleri). Some of these localities contain multiple tetrapod species.
Slightly younger than Zachełmie is the Valentia Slate Formation from Valentia Island, SW Ireland, with a minimum age of 384.9 Ma (mid–late Givetian) provided by radiometric dating of the overlying Enagh Tuff (Stössel et al. Reference Stössel, Williams and Higgs2016). The Valentia Slate contains several tetrapod trackway localities with numerous long quadrupedal tracks showing unambiguous lateral sequence walk locomotion (Stössel Reference Stössel1995; Stössel et al. Reference Stössel, Williams and Higgs2016). The maximum size of the tetrapods is approximately 1m, which is similar in size to Ichthyostega. Interestingly, the Valentia Slate is only marginally older than the end-Givetian Gauja Formation of the Main Devonian Field, which marks the first appearance of Panderichthys in the Baltic mega-delta and, thus, the beginning of the elpistostegid body fossil record. Both lay in the tropics on the south-eastern margin of Laurussia, but the environments were very different. The Valentia Slate represents a proximal part of the Munster Basin, close to the highlands that formed its northern boundary (Stössel et al. Reference Stössel, Williams and Higgs2016). The sea lay at least 200km away to the south. In a generally arid landscape, the highlands seem to have captured rainfall, giving rise to permanent streams that flowed south to eventually lose themselves in ephemeral channels on the floodplain (Fig. 6b). The tetrapod trackways occur exclusively in association with sandy river channels and immediately adjacent environments, not in the fine-grained sediments of the lower floodplain. Some of the trackways were made in very shallow water, some apparently on land (Stössel et al. Reference Stössel, Williams and Higgs2016).
Two Late Devonian tetrapod trackway localities with less tightly constrained ages also need to be considered: Tarbat Ness in Scotland, which is probably Frasnian, and Genoa River in Victoria, Australia, which is probably Famennian (Warren & Wakefield Reference Warren and Wakefield1972; Rogers Reference Rogers1990). The Tarbat Ness trackway (Fig. 4) is short and lacking in anatomical detail, but shows a typical lateral sequence pattern very similar to the Valentia Slate and Genoa River tracks (Rogers Reference Rogers1990). The tetrapod was apparently walking on a moist, sandy sabkha surface, in a semi-arid landscape with migrating dune fields, about 100km from the nearest sea (Fig. 6b); ephemeral rivers issued from higher ground to the NE, but did not reach the trackway locality (Marshall et al. Reference Marshall, Rogers and Whiteley1996). An associated ichnofauna of large terrestrial arthropod tracks and smaller burrows provides a hint of the other inhabitants (Rogers Reference Rogers1990). The Genoa River trackway locality, which I visited in late 2017 on an expedition organised by John Long, lies within the proximal, sand-dominated part of the fluviatile Combyingbar Formation (Fig. 6c), which is very poor in body fossils but has yielded a single large tetrapodomorph fish jaw near the track site (Holland Reference Holland2010).
It should be immediately obvious that these localities have a great deal in common. They do not represent tidal, deltaic environments or the lower reaches of big rivers. All were located far inland, except Zachełmie, which nevertheless resembles the others in lying on a dry plain. At Valentia Island and Tarbat Ness, we have direct evidence that the tetrapods were moving about on land. At Zachełmie the tracks are subaquatic, but it is difficult to envisage the shallow ephemeral lakes with their depauperate fauna providing a food source sufficient to sustain 2-m predatory tetrapods for any length of time; they more likely foraged elsewhere, perhaps on land (Qvarnström et al. Reference Qvarnström, Szrek, Ahlberg and Niedzwiedzki2018). Two other points are of importance. Firstly, at all these localities body fossils are either absent (Zachełmie, Tarbat Ness) or rare (Valentia Island, Genoa River). They thus demonstrate that tetrapods from the Middle Devonian onwards were active in environments that do not tend to be captured by the body fossil record. In effect, these trackways give us a privileged glimpse of one of the ‘unknown unknowns'. Secondly, the geographical distribution is worth noting. Valentia Island, Tarbat Ness and Zachełmie all cluster together in southern Laurussia, but Genoa River is a real outlier on the eastern part of Gondwana, halfway round the planet from the others. This distribution, coupled with a similar pattern for body fossils – most Devonian tetrapods are Laurussian, but Metaxygnathus comes from Australia, and Umzantsia and Tutusius from a high-palaeolatitude locality in South Africa (Campbell & Bell Reference Campbell and Bell1977; Ahlberg & Clack Reference Ahlberg and Clack1998; Gess & Ahlberg Reference Gess and Ahlberg2018) – suggests that tetrapods were globally distributed by the end of the Devonian, if not before.
It is possible that the tetrapod body fossil record begins already with the Givetian genus Livoniana, which occurs alongside Panderichthys in the Gauja Formation (Ahlberg et al. Reference Ahlberg, Luksevics and Mark-Kurik2000). Livoniana has a lower-jaw morphology more tetrapod-like than that of Tiktaalik, but no other part of the body is known. The earliest definite tetrapod remains are those of Elginerpeton from Scotland, Obruchevichthys from Latvia and Webererpeton from Russia, all late Frasnian (Vorobyeva Reference Vorobyeva1977; Ahlberg Reference Ahlberg1991, Reference Ahlberg1995, Reference Ahlberg and Johanson1998, 2011; Ahlberg & Clack Reference Ahlberg and Johanson1998; Ahlberg et al. Reference Ahlberg, Friedman and Blom2005b; Clément & Lebedev Reference Clément and Lebedev2014). Obruchevichthys and Webererpeton both come from the Main Devonian Field, the delta complex that had been inhabited by Panderichthys and Livoniana some ten million years previously. Both are known from isolated lower-jaw fragments (Ahlberg Reference Ahlberg and Trewin1995; Ahlberg & Clack Reference Ahlberg and Johanson1998; Clément & Lebedev Reference Clément and Lebedev2014).
The Elginerpeton material is more extensive, consisting of numerous lower-jaw fragments that, together, represent almost the whole ramus, along with premaxillae, shoulder girdle fragments, pelvic fragments and a probable femur. Elginerpeton is a very distinctive tetrapod: it is large (probably with a body length of at least 1.5m, assuming normal early tetrapod proportions), with a slender elongate lower jaw and a narrow, pointed snout, suggesting a specialist piscivore, quite unlike the spade-shaped snout familiar from Ichthyostega, Acanthostega and Ventastega (Jarvik Reference Jarvik1996; Clack Reference Clack2002a, Reference Clackb; Ahlberg et al. Reference Ahlberg, Clack, Luksevics, Blom and Zupins2008). The shoulder girdle and pelvis are robust and broadly resemble those of Ichthyostega, as far as can be determined from the preserved fragments; the ilium has a typical tetrapod form and a sacral contact seems to have been present (Ahlberg Reference Ahlberg and Johanson1998). The femur, albeit incomplete at both ends, shows pronounced torsion that would result in the flexor surface of the lower leg facing posteriorly – a good shape for a paddle but not for a walking leg. All in all, Elginerpeton gives the impression of being predominantly, if not completely, aquatic.
The Elginerpeton locality, Scat or Scaat Craig (both spellings occur in the literature), is south of Elgin, only about 43km SE of Tarbat Ness, and, more importantly, on the northern flank of the central highlands of Scotland. These mountains had formed during the Late Silurian–Early Devonian and were probably still of alpine proportions during the Frasnian. The fossil-bearing deposit is a coarse sandstone containing small pebbles and mud clasts, overlying a conglomerate of well-rounded cobbles (pers. obs.). All the vertebrate material apart from small elements like scales is broken and shows clear evidence of transport. Elginerpeton is accompanied by a fish assemblage that includes placoderms (e.g., Newman Reference Newman2005), sarcopterygians (Ahlberg Reference Ahlberg1992) and the psammosteid Psammosteus falcatus, also known from the Main Devonian Field (Obruchev & Mark-Kurik Reference Obruchev and Mark-Kurik1965). This assemblage clearly derives from a large permanent water body, most likely a river flowing N or NE from the mountains, but unfortunately the transported nature of the material precludes a more detailed interpretation. The presence of Psammosteus falcatus is interesting in view of its occurrence in the deltaic environments of the Main Devonian Field.
Slightly younger tetrapods are known from the early Famennian of Sosnogorsk, Komi Republic, and Gornostayevka Quarry, Oryol Region, both in Russia. The Sosnogorsk tetrapod is currently under description; see Ahlberg et al. (Reference Ahlberg, Beznosov, Luksevics and Clack2011) for a preliminary note. The Gornostayevka tetrapod, Jakubsonia, was described by Lebedev (Reference Lebedev2004). Jakubsonia was found in a marine nearshore deposit, but the fragmentary material has been transported. The Sosnogorsk tetrapod comes from what appears to be a brackish coastal lagoon on the east coast of Laurussia, separated from the sea by an exposed former barrier reef (Fig. 6c). The deposit contains a rich fish fauna, abundant charophyte algae and invertebrate burrows referable to Thalassinoides isp. and Balanoglossites isp. (Luksevics et al. Reference Luksevics, Beznosov and Sturis2017). Here the tetrapod material, comprising a large number of bones from several individuals, shows no sign of transport. Interestingly, the latest Famennian Andreyevka-2 locality near Tula, Russia, which yielded Tulerpeton, appears to represent a very similar environment (Lebedev Reference Lebedev and Mark-Kurik1992).
These early body fossil localities have a quite different character from the trackway localities previously discussed. With the sole exception of Scat Craig, they are all interpreted as deltaic, estuarine, lagoonal or nearshore marine. Scat Craig appears to have been located some distance inland, in the upper part of its sedimentary basin judging by the coarseness of the sediment, but the fish fauna suggests a large, permanent river connected to the sea. These characteristics are reminiscent of the elpistostegid localities of the late Givetian to early Frasnian.
Later Famennian tetrapod localities include Pavari and Ketleri in Latvia, which yield Ventastega (Ahlberg et al. Reference Ahlberg, Luksevics and Lebedev1994, Reference Ahlberg, Clack, Luksevics, Blom and Zupins2008; Luksevics & Zupins Reference Luksevics and Zupins2004); Waterloo Farm in South Africa, which yields Umzantsia and Tutusius (Gess & Ahlberg Reference Gess and Ahlberg2018); and the Catskill Formation localities in Pennsylvania such as Red Hill, source of Hynerpeton, Densignathus, the humerus ANSP 21350 and an unnamed Whatcheriid-like tetrapod (Daeschler et al. Reference Daeschler, Thomson and Amaral1994, Reference Daeschler, Clack and Shubin2010; Daeschler Reference Daeschler2000; Shubin et al. Reference Shubin, Daeschler and Coates2004). Pavari is interpreted as a shallow, nearshore marine, tidally influenced environment (Luksevics & Zupins Reference Luksevics and Zupins2004) in a late phase of the Main Devonian Field delta complex. Red Hill, on the other hand, is non-marine and represents a combination of channel, overbank and pond environments on a floodplain characterised by repeated avulsions (Cressler et al. Reference Cressler, Daeschler, Slingerland and Peterson2010); in general terms, the floodplain was coastal, bounded by an inland sea to the west, but Red Hill was quite far inland. The terrestrial environment was richly vegetated, subject to occasional wildfires and inhabited by arthropods, including trigonotarbids and myriapods. A taxonomically and morphologically diverse fish fauna ranged from the small actinopterygian Limnomis to the giant tristichopterid Hyneria, which may have reached a length of 3m (Cressler et al. Reference Cressler, Daeschler, Slingerland and Peterson2010). The Belgian Famennian localities of Strud and Becco, which have yielded fragmentary material of an Ichthyostega-like tetrapod and a probable Whatcheriid, are also fluvial coastal-plain localities (Clément et al. Reference Clément, Ahlberg, Blieck, Blom, Clack, Poty, Thorez and Janvier2004; Olive et al. Reference Olive, Ahlberg, Pernègre, Poty, Steurbaut and Clément2016). Unlike Pavari, Red Hill and the Belgian localities, all of which occupy palaeolatitudes of no more than 30°S, Waterloo Farm lay at more than 70°S, inside the Antarctic Circle (Gess & Ahlberg Reference Gess and Ahlberg2018). It is an estuarine locality containing a mixed fauna of marine and non-marine taxa including chondrichthyans, arthrodires, antiarchs, acanthodians, coelacanths, a large Hyneria-like tristichopterid and the earliest known lamprey (Gess et al. Reference Gess, Coates and Rubidge2006; Gess Reference Gess, Linol and de Wit2016). Terrestrial arthropods are represented by the scorpion Gondwanascorpio (Gess Reference Gess2013). Despite the high-latitude location, the locality yields a rich flora, including the cosmopolitan progymnosperm Archaeopteris (Anderson et al. Reference Ahlberg, Beznosov, Luksevics and Clack1995). Thus, the climate must have been reasonably temperate.
Among all the Devonian tetrapod body fossil localities, those of East Greenland stand out because of their extensiveness and the quality of the tetrapod material. Ichthyostega and Acanthostega are still the only Devonian tetrapods for which reliable whole-body reconstructions are available (Coates Reference Coates1996; Ahlberg et al. Reference Ahlberg, Clack and Blom2005a; Pierce et al. Reference Pierce, Clack and Hutchinson2012); a third genus, Ymeria, is known only from jaw and palate material (Clack et al. Reference Clack2012). In contrast to most of the localities discussed so far, the position of the East Greenland sedimentary basin was mid-continental in Laurussia, lying immediately to the west of a north–south-trending mountain range created by the Caledonian orogeny; the eastern flank of the same mountain chain seems to have been the source of the rivers that fed the Baltic mega-delta (Tovmasjana Reference Tovmasjana2013). The sedimentology and palaeoenvironment of the Celsius Bjerg Group, which contains the Famennian tetrapod-bearing strata of East Greenland, were examined in detail by Astin et al. (Reference Astin, Marshall, Blom and Berry2010). The oldest formation, the Elsa Dal Formation, represents braided river systems with lakes in the upper part, but has yielded no tetrapods. The succeeding Aina Dal Formation represents meandering rivers and muddy overbank deposits. Here, Acanthostega and Ichthyostega both appear for the first time, accompanied by fish fauna, including the antiarch Remigolepis and the large Hyneria-like tristichopterid Eusthenodon (Jarvik Reference Jarvik1952). The Aina Dal Formation is followed by the unfossiliferous playa lake deposits of the Wimans Bjerg Formation, and then by the Britta Dal Formation, which was the principal focus of interest for Astin et al. (Reference Astin, Marshall, Blom and Berry2010). The two most famous tetrapod mass occurrences, the Ichthyostega mass-death deposit of the so-called 1174 horizon on Sederholms Bjerg (Jarvik Reference Jarvik1996) and the Acanthostega mass-death deposit on Stensiö Bjerg (Clack Reference Clack1988; Astin et al. Reference Astin, Marshall, Blom and Berry2010), both lie within this formation. It is interpreted as a large ephemeral braided fluvial system (more than 200km long and 50km wide) flowing northwards and gradually dissipating through an arid landscape. Seasonal rains brought silt-laden water down into this system, slowly building up deep vertisols, but every so often (presumably with intervals of decades to centuries) much bigger rainfalls caused the ephemeral rivers to go into spate and flood the whole area, carrying sand that was deposited in a more or less continuous but channel-carved sheet. In the aftermath of these flood events, pools and billabongs dotted the floodplain and may have persisted from one wet season to the next, allowing the area to be colonised by fish, tetrapods and vegetation (Astin et al. Reference Astin, Marshall, Blom and Berry2010). In general terms, this late Famennian system seems quite similar to the late Givetian Valentia Slate, but it contains a much richer body fossil record.
The Ichthyostega and Acanthostega mass-death deposits are both associated with sandstone beds and, thus, with major flooding events. Unfortunately, the Ichthyostega deposit of the 1174 horizon was largely dug out by the Danish–Swedish expeditions of the mid-20th Century, without detailed sedimentological notes being taken, with the result that the precise depositional environment cannot now be reconstructed. The Acanthostega deposit has been less completely excavated and could be interpreted in detail by Astin et al. (Reference Astin, Marshall, Blom and Berry2010). It represents a small channel peripheral to a major channel system, which retained water for some time after the floodwaters had drained away. A school of Acanthostega individuals, which had been swept down into the ephemeral river system by the flood, found refuge for a time in this pool but eventually died as the water disappeared.
Astin et al. (Reference Astin, Marshall, Blom and Berry2010) recognised that the depositional environment of the Britta Dal Formation was not the normal living environment of the animals found in it; everything has been swept down from another unpreserved environment somewhere upstream. This must have been a sizable, permanent water body, as its inhabitants included large lobe-finned fishes such as Holoptychius and Eusthenodon. Once again we are brought back to the surprisingly useful conceptual model of Rumsfeld, because a single fossil specimen sharply illuminates the difference between a ‘known unknown' – in this case the source environment for this vertebrate fauna, which we know must have existed somewhere just outside our field of view – and the ‘unknown unknowns' that lie beyond. One specimen of Acanthostega from the mass-death occurrence, MGUH VP (Vertebrate Palaeontology Collection, Geological Museum, University of Copenhagen) 1300a, has fragments of two fin spines caught in its lower jaw (Ahlberg & Clack Reference Ahlberg and Clack1998, fig. 3). The spines have been identified by Michael Coates as most probably deriving from a ctenacanth shark. Their position in the jaw strongly suggests that they are the result of a life interaction: the Acanthostega ate, or at least bit, the shark, and the fin spines stuck in its gums. But decades of intensive fossil collecting in the Celsius Bjerg Group, beginning in 1928, has never uncovered a single specimen of a ctenacanth shark, or indeed of any similar spine-bearing fish such as an acanthodian. The importance of this mismatch can scarcely be overstated. It implies that there were two unknown environments ‘off stage': one of them, the immediate source of the biota that was flushed out into the preservation environment of the Britta Dal Formation during floods, contained the familiar Celsius Bjerg Group fish fauna but no sharks; the other environment, which was not sampled at all by fossil preservation processes, contained sharks – and who knows what else. Acanthostega could move between the two unknown environments, and at least one individual (but possibly the whole shoal) did so shortly before being flushed out onto the plain of death and preservation that created the Britta Dal Formation. Were it not for MGUH VP 1300a, we would have no idea about the existence of the second environment, which was certainly used by Acanthostega and may, for all we know, have been its primary home.
After the initial submission of this manuscript, a further twist to the story of the East Greenland environment was provided by Goedert et al. (Reference Goedert, Lécuyer, Amiot, Arnaud-Godet, Wang, Cui, Cuny, Douay, Fourel, Panczer, Simon, Steyer and Zhu2018), who used a stable isotope analysis of δ13C, δ18O and δ34S to show a euryhaline environmental signal in the bones of a range of Devonian vertebrates, including tetrapods from East Greenland. Unfortunately, the precise source localities of their tetrapod bones, all of which came from the collections amassed by the Danish–Swedish expeditions in the 1930s–1950s, are not specified in the paper; they may include material from both the Aina and Britta Dal formations, but will not be from the Acanthostega mass-death locality, which had not yet been discovered at that time. A euryhaline lifestyle would not in itself be surprising, given the well-established association between certain other Devonian tetrapods and marine-influenced environments, and it is certainly possible that the mystery environment where Acanthostega met the spine-bearing fish was marine or brackish. However, all recent palaeocontinental reconstructions for the Famennian place East Greenland in the middle of continental Laurussia, nearly 1000km from the nearest coast. If the reconstructed coastlines are correct, this implies migration over remarkably long distances; for comparison, the chinook salmon run of the Yukon River reaches up to 3000km inland from the river mouth (Eiler et al. Reference Eiler, Masuda, Spencer, Driscoll and Schreck2014), but salmon are powerful swimmers and it is difficult to imagine Acanthostega managing a similar performance.
To summarise, the tetrapod-yielding localities of the Devonian fall into several distinct geographical and environmental categories (Fig. 6). The Valentia Island, Genoa River, Tarbat Ness, East Greenland, Catskill Formation and Scat Craig localities appear to represent inland environments, 100km or more from the nearest coast. At all except Tarbat Ness, which is a sabkha deposit, the sediment has been laid down by flowing water. The Catskill Formation and Genoa River lay at a palaeolatitude of about 30°S, but all the other localities belong to the southern arid belt, 10–15°S of the equator. Although the Catskill Formation environment may have been less arid, the occurrence of wildfires nevertheless suggests a seasonally dry regime. Thus, somewhat counterintuitively, dry inland environments appear to have been important tetrapod habitats from at least the late Middle Devonian. Nevertheless, all these environments except Tarbat Ness contained large fishes that would have required permanent water. Zachełmie, Sosnogorsk and possibly Andreyevka-2 are coastal-plain lakes or lagoons, but Sosnogorsk contains a rich assemblage of fish and tetrapod body fossils, whereas Zachełmie lacks them altogether. A third, very different type of environment is represented by Velna-Ala in Latvia (which yields Obruchevichthys), Gornostayevka in Russia (which yields Jakubsonia), Pavari and Ketleri in Latvia (which yield Ventastega) and Waterloo Farm in South Africa (which yields Umzantsia and Tutusius). These localities are all deltaic to tidal shallow marine. Velna-Ala, Pavari and Ketleri are located in the Main Devonian Field and are, in a sense, direct successors to the Panderichthys localities of the late Givetian.
Perhaps the most striking thing about this pattern of occurrences is that there is no temporal trend from coastal, tidal and deltaic/estuarine environments towards dry inland environments, as might be expected if the record reflected the origin of tetrapods and their colonisation of the land (Fig. 6). If anything, the coastal and river-mouth environments seem to be colonised later than the inland environments; the fact that these environments in the Givetian and early Frasnian contain elpistostegids but not tetrapods suggests that the absence of tetrapods there is real and not just due to failure of preservation.
6. Ichthyostega and Acanthostega at home
The picture of Devonian tetrapods that emerges from this overview of their morphology and living environments differs substantially from the established ‘aquatic origin' scenario centred around Acanthostega. While it is true that all Devonian tetrapods retained strong ties to the water, demonstrated by their possession of well-developed lateral-line canals, they also show a range of structural innovations (digits, flexed elbow, sacrum, reinforced vertebral column) that only make sense as adaptations for terrestrial activity. Trackway evidence shows that they were, indeed, active on land, and that at least some were able to perform lateral sequence walks, as early as the Middle Devonian. ‘Halfway up' in this case seems to mean a lifestyle stretched between aquatic and terrestrial poles, presumably on a daily cycle. Against this background, it is now time to reconsider Ichthyostega and Acanthostega, the only reasonably complete Devonian tetrapods. What do they actually tell us?
It is obvious at a glance that Ichthyostega and Acanthostega are very different animals (Fig. 1). Ichthyostega has proportionately larger limbs and girdles, a real ribcage with long and deeply overlapping thoracic ribs, a partly ossified sternum, a lumbar region with short, non-overlapping ribs, regionalised neural-arch morphologies and a tail with a narrow lepidotrichial fringe (Jarvik Reference Jarvik1952, Reference Jarvik1996; Ahlberg et al. Reference Ahlberg, Clack and Blom2005a; Pierce et al. Reference Pierce, Clack and Hutchinson2012, Reference Pierce, Ahlberg, Hutchinson, Molnar, Sanchez, Tafforeau and Clack2013). In Acanthostega the limbs and girdles are small, the ribs short and undifferentiated, the sternum unossified, the vertebral column less regionalised and the tail fin large and leaf-shaped (Coates Reference Coates1996). However, these differences are almost entirely matters of proportion; with one important exception (see below, this section), the underlying bauplan is essentially identical. The conclusion that Ichthyostega is more terrestrially adapted than Acanthostega is inescapable, irrespective of the exact degree of mobility of its limbs (Pierce et al. Reference Pierce, Clack and Hutchinson2012). Further light has recently been thrown on the lifestyle of Acanthostega through the investigation of its limb-bone histology by synchrotron microtomography (Sanchez et al. Reference Sanchez, Tafforeau, Clack and Ahlberg2016). This showed that limb-bone ossification began very late, after a juvenile stage with cartilaginous limb skeletons that probably lasted several years. Evidently, Acanthostega was entirely, or at least overwhelmingly, aquatic as a juvenile. As an adult it probably had a greater degree of terrestrial competence, but its large and deep tail fin suggests that it was very much a habitually aquatic animal. It is interesting to note in this context that Ventastega, which occurs in a tidal deposit and is associated with long lepidotrichia suggesting an Acanthostega-like tail, appears to have had a very lightly ossified postcranial skeleton; no limb bones have been found, and both pelvis and scapulocoracoid are partly unossified (Ahlberg et al. Reference Ahlberg, Clack, Luksevics, Blom and Zupins2008).
This aquatic adaptive profile has commonly been regarded as primitive for tetrapods, with Acanthostega in effect being viewed as a sort of elpistostegid with legs. Even setting aside the point that elpistostegids already show adaptations for weight-bearing and a modest degree of terrestrial activity (see Section 3), there are several problems with this hypothesis. It has never satisfactorily explained what selection pressure would drive the evolution of features such as flexed elbows and laterally oriented appendages, which would certainly make the animal less well-adapted to swimming, or a sacrum, which is a weight-supporting structure that is not needed for underwater propulsion. In short, it could never explain why a persistently aquatic animal would not simply remain a fish. Another side to the problem is revealed by a close examination of the humeral muscle attachments (Fig. 2). The great majority of recent phylogenies place Ichthyostega crownward to Acanthostega, probably because of its terrestrial adaptations (e.g., Ruta & Clack Reference Ruta and Clack2006; Warren Reference Warren2007; Ahlberg et al. Reference Ahlberg, Clack, Luksevics, Blom and Zupins2008; Clack et al. Reference Clack2012). However, the humerus of Acanthostega carries a deltopectoral crest, essentially similar to our own (Coates Reference Coates1996), whereas Ichthyostega has distinct deltoid and pectoral processes separated by a prepectoral space (Callier et al. Reference Callier, Clack and Ahlberg2009; Ahlberg Reference Ahlberg, Beznosov, Luksevics and Clack2011). This fundamentally aligns Ichthyostega with less crownward members of the tetrapod stem group such as ANSP 21350, Tiktaalik and Panderichthys (Callier et al. Reference Callier, Clack and Ahlberg2009; Ahlberg Reference Ahlberg, Beznosov, Luksevics and Clack2011). Initially, in elpistostegids and less crownward tetrapodomorph fishes, there was no pectoral process at all, only a tall but undifferentiated middle region of the oblique ventral ridge (Fig. 2). The pectoral process is first seen in incipient form in ANSP 21350 (Callier et al. Reference Callier, Clack and Ahlberg2009; Ahlberg Reference Ahlberg, Beznosov, Luksevics and Clack2011). Its evolution and subsequent preaxial displacement to merge with the deltoid process relates to the subdivision and elaboration of appendage musculature across the fish–tetrapod transition (Molnar et al. Reference Molnar, Diogo, Hutchinson and Pierce2017). To my mind, this is fairly compelling evidence that Acanthostega is, in fact, more crownward than Ichthyostega, notwithstanding the consensus of recent phylogenies.
If Acanthostega is a relatively crownward Devonian tetrapod, and has adaptations for terrestrial locomotion that cannot really be explained in the context of its evidently aquatic lifestyle, could it be that this lifestyle is, to some extent, a reversal from a more terrestrially adapted ancestor? It is widely agreed that many later ‘early tetrapods', ranging from Crassigyrinus and colosteids to stereospondyls such as Metoposaurus, were, to varying degrees, secondarily aquatic (Carroll Reference Carroll2009). This has never been proposed for Acanthostega, because its retention of genuine ‘fish' characters such as lepidotrichia and gill arches (Coates & Clack Reference Coates and Clack1991; Coates Reference Coates1996; Janis & Farmer Reference Janis and Farmer1999) appear to rule out such an evolutionary trajectory. But these characters are not unique to Acanthostega among Devonian tetrapods; notably, a small lepidotrichial tail fin is also present in the much more terrestrial-looking Ichthyostega (Jarvik Reference Jarvik1952, Reference Jarvik1996), while internal gills appear to be widely distributed among early tetrapods up to and including temnospondyls (Schoch & Witzmann Reference Schoch and Witzmann2010). This raises an interesting hypothetical question: if reversal to a more fully aquatic lifestyle were to occur in a taxon that had not yet lost its ‘fish' characters, so that the adaptation back to aquatic life simply involved re-emphasising these characters (for example, making the tail fin bigger again), would it possible to tell from the resulting morphology what had happened, or would it be indistinguishable from the primitive state? It is possible that Acanthostega, far from being a uniquely primitive tetrapod showing the evolution of tetrapod characteristics in the context of an aquatic lifestyle, is relatively derived among Devonian tetrapods and presents the earliest known example of a secondary reduction in terrestriality.
7. Putting it all together
The single most important take-home message from this survey of the evidence is that the fossil record of the fish–tetrapod transition is actually very poor and consists mostly of gaps. It is only when we give this fact proper consideration that it becomes possible to begin fitting together the few pieces of information we do have, using a combination of phylogenetic analysis, environmental interpretation and careful dating.
There is now a broad morphological and molecular consensus that lungfishes are the extant sister group of tetrapods (e.g., Lu et al. Reference Lu, Zhu, Long, Zhao, Senden, Jia and Qiao2012; Amemiya et al. Reference Amemiya2013). The lungfish and tetrapod total groups must thus be equally old. The earliest known unambiguous stem-group lungfish is Diabolepis from the Lochkovian (earliest Devonian) of Yunnan, China, approximately 415 million years old (Chang & Yu Reference Chang and Yu1984; Chang Reference Chang, Arsenault, Lelievre and Janvier1995). This is thus a minimum age for the beginning of the tetrapod stem group; i.e., for the origin of the tetrapodomorph fishes (Ahlberg Reference Ahlberg1991). From later Early Devonian strata there is quite a diversity of stem lungfishes, as well as porolepiform fishes, which constitute a basal branch in the lungfish stem group (Ahlberg Reference Ahlberg1991). The tetrapod stem group, on the other hand, is extremely poorly represented in the Early Devonian; only two genera are known, Tungsenia from the Pragian and Kenichthys from the Emsian, both from Yunnan (Zhu & Ahlberg Reference Zhu and Ahlberg2004; Lu et al. Reference Lu, Zhu, Long, Zhao, Senden, Jia and Qiao2012). Both are small cosmine-covered fishes, probably similar in general outline to Middle Devonian ‘osteolepidids' such as Osteolepis and Thursius, but possessed of some unique primitive characters that show them to occupy phylogenetically basal positions among tetrapodomorphs. In Laurussia, tetrapodomorph fishes do not appear in the fossil record until the beginning of the Eifelian, shortly before the Zachełmie footprints were made – and these fishes are small cosmine-covered osteolepidids (e.g., Jarvik Reference Jarvik1948, Reference Jarvik1980). If our tetrapodomorph phylogeny is correct, which seems likely given the congruent character distributions and the topological consistency of recent analyses (e.g., Ahlberg & Johanson Reference Ahlberg and Clack1998; Daeschler et al. Reference Daeschler, Shubin and Jenkins2006; but see Zhu et al. Reference Zhu, Ahlberg, Zhao and Jia2017), the origin of the tetrapods, elpistostegids, tristichopterids and rhizodonts must all be datable to the earliest Eifelian or earlier. This seems like a realistic proposition given that it allows a time frame of some 24 million years from the origin of the tetrapod total group in the Lochkovian (see also Friedman & Brazeau Reference Friedman and Brazeau2010), but there is not a single piece of body fossil evidence to support it.
The next problem we encounter is the central theme of this paper: that tetrapods, represented by footprints, occur in the fossil record well before the elpistostegids (Fig. 5). The Zachełmie tracks are ten million years older than Panderichthys, but even the younger and less controversial Valentia Slate trackways, which include a number of long lateral sequence walks made in very shallow water or on land (Stössel et al. Reference Stössel, Williams and Higgs2016), are slightly older than Panderichthys. It should be remembered here that Panderichthys is generally regarded as the least crownward elpistostegid (Daeschler et al. Reference Daeschler, Shubin and Jenkins2006; Ahlberg et al. Reference Ahlberg, Clack, Luksevics, Blom and Zupins2008). The more tetrapod-like Tiktaalik is of early Frasnian age, probably between two and four million years younger than Panderichthys. The idea of Tiktaalik as an immediate predecessor of tetrapods, which has been promulgated both in the scientific literature (Daeschler et al. Reference Daeschler, Shubin and Jenkins2006; Shubin et al. Reference Shubin, Daeschler and Jenkins2006) and in innumerable popular presentations, must thus be discarded if the footprint evidence is accepted; instead, Tiktaalik and Elpistostege are cast in the role of late survivors of the elpistostegid radiation.
The idea of tetrapods and elpistostegids living side by side from at least the beginning of the Eifelian until the mid-Frasnian, a time span of some 15 million years, is startling but not as strange as it sounds. It is tempting to view elpistostegids simply as an intermediate step in a directional evolutionary progression, but of course they were nothing of the sort; like all organisms, they were adaptively optimised for their own lifestyle and not ‘on their way' to anywhere. An instructive comparison is provided by another evolutionary transition to a new living environment, namely the origin of birds. The transition from terrestrial theropod dinosaurs to flying birds occurred within the bird stem group, as documented in great detail by a fossil record considerably richer than that of stem tetrapods (e.g., Foth & Rauhut Reference Friedman and Brazeau2017, and references therein). The uppermost part of the stem group consists of birds that were evidently capable of flight but lacked parts of the crown-group character complement. Below them lies a stem segment occupied by a range of groups such as microraptorids and oviraptorids, which have well-developed plumage including small ‘wings' but retain a conventional theropod skeletal morphology and were presumably not capable of flapping flight. For the purposes of comparison, we can equate the former with Devonian tetrapods and the latter with elpistostegids. Archaeopteryx from the Tithonian (Late Jurassic) of Germany, approximately 150 million years old (Foth & Rauhut Reference Foth and Rauhut2017), is arguably (depending on the phylogenetic position of Epidexipteryx) the oldest and least crownward members of the ‘bird' stem segment. The less crownward lineages such as microraptorids must thus have originated earlier. However, it is not until the Early Cretaceous Jehol biota from Liaoning, China, that we get a good fossil record of these feathered theropods, which are then living alongside quite advanced flying birds (Zhou Reference Zhou2014). The Jehol biota is Aptian, about 30 million years younger than Archaeopteryx, and, in fact, birds and feathered theropods continued to coexist long after that. So, while a theropod like Microraptor is a highly informative morphological intermediate that illuminates the origin of birds, it is in no sense a temporal intermediate or ancestor of birds. Against this background there is nothing implausible about a putative 15 million year coexistence of elpistostegids and tetrapods.
The big difference between the bird and tetrapod examples is that birds and feathered theropods occur side by side in the same deposit, whereas tetrapods and elpistostegids do not. The obvious explanation is that the environmental and ecological separation between feathered theropods and birds was essentially vertical – ground versus air – whereas that between elpistostegids and tetrapods was horizontal, relating to occupation of different parts of the landscape (Fig. 6). We have seen that elpistostegids were present in the lower reaches of major rivers, and in deltas and estuaries, from the late Givetian until the mid-Frasnian. Although we need to be careful about statements of absence in the tetrapod fossil record, because they are so easily confused with mere absence of evidence, it is probably fair to say that tetrapods were not present – or at least not abundant – in these environments at that time. If they had been reasonably common they would almost certainly have been found alongside the elpistostegids. Judging by the footprint evidence, the tetrapods were living in the upper parts of river drainages and around shallow water bodies on the coastal plains. These environments were not conducive to the preservation of body fossils.
The most puzzling aspect of the elpistostegids is their complete absence from the fossil record during the Eifelian and early part of the Givetian. The Baltic mega-delta was already in existence at the beginning of the Middle Devonian (Tovmasjana Reference Tovmasjana2013), and the fossil assemblages of its Eifelian and early Givetian strata have been studied extensively (e.g., Obruchev & Mark-Kurik Reference Obruchev and Mark-Kurik1965; Lyarskaya Reference Lyarskaya1981), without any trace of elpistostegids being found. Presumably they were somewhere else, either environmentally or biogeographically, but at present we have no evidence as to where.
The final piece of the jigsaw that we need to consider is the disappearance of the elpistostegids from their fluvial and deltaic environments, and their replacement in these environments by tetrapods (Fig. 6). This replacement, which took place during the mid–late Frasnian, has in the past been interpreted as representing the evolution of tetrapods from elpistostegids, more or less in real time. In fact, the elpistostegids simply went extinct and were replaced by tetrapods moving into the habitats they had occupied. However, while this general conclusion is robustly supported by the modest amount of available data, the details of the replacement process still elude us. Were the last elpistostegids outcompeted by tetrapods, or did the latter simply move in to fill vacant niches following the extinction of the elpistostegids? Just how precise was the ecological replacement? Was it aquatic tetrapods similar to Acanthostega that moved into these environments? At present there is no way of knowing, although the somewhat Acanthostega-like morphology of Ventastega hints at an affirmative answer to the last question.
8. A new scenario
Here, then, is the bare outline of a new scenario for the origin of tetrapods (Figs 5–7): the tetrapod stem group originated during the Lochkovian, most probably in the South China microcontinent. The earliest members were small cosmine-covered fishes. By the beginning of the Eifelian, some 25 million years later, elpistostegids and primitive tetrapods had evolved, though there is at present no direct evidence for the exact timing or location of these evolutionary events. In contrast to the earliest members of the stem group, the elpistostegids and tetrapods were quite big animals, typically 1m or more in length. Their evolution involved a two-stage process of adaptation to substrate locomotion, weight support and aerial vision, evidently in response to selection for activity in very shallow water and along the shoreline. Elpistostegids did not take the process very far, retaining adaptations for water-column swimming such as paired fin webs, straight elbows and posteriorly oriented pelvic fins. Tetrapods acquired a suite of additional adaptations for weight support and substrate walking, including flexed elbows, digits, laterally projecting hindlimbs and a sacrum, all of which were either unnecessary or positive encumbrances in the water column. These features indicate a more terrestrial lifestyle than that of elpistostegids. The terrestrialisation process involved a certain amount of parallelism between closely related lineages, as shown by the independent evolution of two different types of flexed elbow. Although their limb mobility was limited compared to modern tetrapods, some at least were able to perform a lateral sequence walk. Nevertheless, both elpistostegids and tetrapods remained firmly tied to the water, as shown by the retention of lateral-line canals. The position of the eyes on top of the head in both groups, and the loss of lateral-line canals specifically on the skull table in tetrapods (the condition in elpistostegids is unknown), suggest that much of the time in the water was spent in a crocodile-like pose with the eyes projecting above the surface. The loss of adaptations for water-column swimming in tetrapods may reflect a tendency to inhabit smaller water bodies, where this mode of locomotion would be less important, as well as a simple trade-off with increased terrestrial competence. Both groups were predators; their involvement with the terrestrial environment suggests that they sought some of their prey on land, but there is no direct evidence of this, and both elpistostegids and tetrapods seem to have been primarily piscivorous.

Figure 7 Diagram of the stages of the fish–tetrapod transition. Note that this is not a phylogenetic hypothesis but a representation of a step sequence. The steps from Phase 1 to 2 and from Phase 2 to 3 appear to be unique cladogenetic events, but the two alternative steps from Phase 3 to 4 both occurred many times, in parallel, in different tetrapod lineages. The two Phase 4 tetrapods shown here are both temnospondyls and presumably retained aquatic reproduction, but the origin of amniotes would also fit on the upper branch of Phase 4. Archegosaurus modified from Witzmann & Brainerd (Reference Witzmann2017). Cacops modified from a drawing by S. W. Williston, available at https://commons.wikimedia.org/wiki/File:Cacops.jpg. Eusthenopteron, Tiktaalik and Ichthyostega from Fig. 1.
During the Middle Devonian, continuing into the Late Devonian, tetrapods established themselves in relatively dry and often seasonal environments with rivers or pools, ranging from coastal plains to the upper parts of river basins (Fig. 6). Elpistostegids colonised the tidal zone of deltas and estuaries, and the lower reaches of rivers, during the late Givetian and early Frasnian. They are only known from Laurussia, whereas tetrapods also inhabited Gondwana, but this apparent difference may be a sampling artefact. By the middle of the Frasnian the elpistostegids appear to have died out and tetrapods colonised the environments that they had previously inhabited. Tetrapods continued to undergo morphological evolution and diversification during the Late Devonian. The timing of the various steps is not known, but in addition to structural innovations such as the evolution of the deltopectoral crest there was morphological diversification giving rise to forms like the large and probably piscivorous Elginerpeton, the aquatic Acanthostega and the more terrestrial Ichthyostega. The most aquatic tetrapods probably represent reversals from a more terrestrial ancestry. By the late Famennian it was common for two or more tetrapods to occur in the same fauna, and derived forms like Tulerpeton, with a limb morphology approaching that of post-Devonian tetrapods, had evolved.
Although this article focuses principally on the Devonian, one further important step in the process of terrestrialisation took place during the Carboniferous and will be considered briefly here. At the end of the Devonian the most terrestrially adapted tetrapods that we know of still had elaborate lateral-line systems, which must imply that they spent a substantial proportion of their time in the water (Fig. 7). The duration, and thus length, of any excursions onto land must have been short enough to avoid drying out of the skin. During the Carboniferous and Early Permian, tetrapods began to appear with reduced lateral-line systems or none at all: examples include the anthracosaurs Eoherpeton and Anthracosaurus (Panchen Reference Panchen1975, Reference Panchen1977), the stem amniote Silvanerpeton (Ruta & Clack Reference Ruta and Clack2006) and dissorophoid temnospondyls such as Doleserpeton (Sigurdsen & Bolt Reference Sigurdsen and Bolt2010). This does not imply a complete departure from the water; aquatic larvae with external gills are known from the stem amniote Discosauriscus (Klembara Reference Lebedev1995) as well as from temnospondyls and extant amphibians (Witzmann Reference Witzmann2004), so this mode of reproduction must have been present at the tetrapod crown group node, and there is no reason to doubt that the entire tetrapod stem group was characterised by aquatic reproduction. But whereas the Devonian tetrapods all kept a tight association with water into adulthood, these Carboniferous forms seem to have become at least semi-independent of water as adults, perhaps to the same degree as terrestrial lissamphibians today. Before the end of the Carboniferous, the evolution of the amniote egg had freed one tetrapod lineage from the water for good.
This brings us back to the meaning of ‘halfway up' in the context of the fish–tetrapod transition. To my mind, the most striking characteristic of the process is that terrestrial adaptations were initially acquired without any corresponding detachment from the aquatic environment (Fig. 7). Elpistostegids were clearly habitually aquatic and reasonably well equipped for swimming in the water column. The earliest tetrapods to some extent sacrificed water-column swimming ability for improved terrestrial competence, but their retention of elaborate and fish-like lateral-line canals suggests that they did not spend much less time in the water than elpistostegids. We know less about their gills, which is unfortunate given their physiological importance as respiratory and excretory surfaces (Janis & Farmer Reference Janis and Farmer1999), but such evidence as we have suggests that they were retained (Coates & Clack Reference Coates and Clack1991; Schoch & Witzmann Reference Schoch and Witzmann2010; Witzmann & Brainerd Reference Witzmann and Brainerd2017). All in all, we appear to be looking at animals that required frequent access, for prolonged periods, to water deep enough to immerse themselves, even as they actively explored those parts of the terrestrial environment that were within their reach (Fig. 6). It seems that ‘halfway up' for the earliest tetrapods meant not so much ‘neither up nor down' as ‘both up and down at the same time'.
There is no real equivalent to this mode of life today, and in fact it did not prove very long-lasting among tetrapods (Fig. 7). In some groups, as we have seen, loss of the lateral-line canals (and no doubt other changes to the skin, and perhaps the gills) eventually allowed the adults to become less dependent on water; other groups went the other way and abandoned the terrestrial part of their life in favour of a wholly aquatic lifestyle. As I have previously argued, even Acanthostega may, to some degree, have been secondarily aquatic, descended from more terrestrially competent ancestors. What is incontestable is that more derived ‘early tetrapods' again and again gave rise to what are usually described as secondarily aquatic lineages. Distantly related forms such as the stem tetrapod Crassigyrinus (Panchen Reference Panchen1985; Panchen & Smithson Reference Panchen and Smithson1990), the embolomere Archeria (Holmes Reference Holmes1989) and the stereospondyl Metoposaurus (Sulej Reference Sulej2007) all converged on a morphology characterised by small, feeble limbs and well-developed lateral-line canals. It is important to appreciate that these animals were not ‘secondarily aquatic' in the sense that a crocodile is; rather, they were tetrapods whose ancestors had never let go of the water, and which eventually reduced or abandoned the terrestrial part of their lives (Fig. 7). In the end, as fully terrestrial tetrapods including early amniotes diversified, the ‘halfway up' lifestyle that had first brought our ancestors onto the land became increasingly uncompetitive and was abandoned.
9. Afterword: Jenny's role in the revolution
Looking back on the past 30 years of early tetrapod research, I am struck again and again by the central role that Jenny Clack has played in this field. She has been the hub around which the rest of us have revolved. Of course, quite a lot of the tetrapod research I have discussed here has been done by others, sometimes with Jenny's involvement and sometimes not, but I suspect that very little of it would have happened without the tremendous impetus provided by the discovery of the Acanthostega mass-death deposit and the long series of publications that followed from it. Certainly, my own research trajectory would have been very different; for one thing, without the opportunity to see the Acanthostega material emerging from the rock and being allowed to scrutinise it in detail, I would probably not have been able to recognise the fragmentary remains of Elginerpeton and Ventastega when they first came to my attention. The key thing here is not just the quality and importance of the Acanthostega material, but the generosity with which Jenny made it available even to those of us who were not actively involved in its description. The contrast with the preceding era of Devonian tetrapod studies could hardly be more stark, and the extraordinary progress of these three decades should stand in their totality as a monument to Jenny's positive influence.
Nor has this influence come to an end. In recent years it has been a pleasure to watch the TW:eed team, under Jenny's leadership, begin to turn Romer's supposed ‘Gap' into something resembling a crowded railway station platform thronged with newly discovered tetrapods and other animals. Other contributions to this volume will showcase various aspects of that project. One of the most satisfying aspects of this research is the way that it is bringing together the Devonian and Carboniferous chapters in the story of tetrapod evolution, especially now that recent fieldwork in East Greenland by Henning Blom, Ben Kear and Grzegorz Niedzwiedzki has uncovered tetrapod material and other relevant fossils in the latest Famennian Stensiö Bjerg and Obrutschew Bjerg formations, which are considerably younger than the classic Acanthostega and Ichthyostega localities. The time gap between these latest Devonian tetrapod-bearing strata in East Greenland and the earliest Carboniferous strata in the Scottish Borders is trivial. Temporal and taphonomic gaps may bedevil the study of the fish–tetrapod transition, and I have argued here for the importance of giving them their due recognition, but, 86 years after the discovery of Ichthyostega, the granddaddy of them all may finally be in the process of closing.
10. Acknowledgements
My thanks go first and foremost to Jenny Clack, for her unstinting support, interest, kindness and generosity over the years, and above all for inviting me onto the East Greenland expedition of 1987 that proved to be such a watershed for our discipline. My fellow members of the symposium organising committee, Tim Smithson and Marcello Ruta, did a magnificent job and carried a much greater part of the administrative burden than I. Innumerable colleagues have helped to develop my ideas about the fish–tetrapod transition through valuable discussions and access to precious material. I would especially like to thank, in alphabetical order (and in addition to Tim and Marcello, who also belong here): Jason Anderson, Mahala Andrews, Pavel Beznosov, Hans-Christian Bjerring, Henning Blom, Catherine Boisvert, Ulf Borgen, Mee-mann Chang, Gaël Clément, Michael Coates, Peter Forey, Brian Gardiner, Henry Gee, Philippe Janvier, Erik Jarvik, Zerina Johanson, Tom Kemp, Oleg Lebedev, John Long, Ervins Luksevics, Elga Mark-Kurik, Andrew and Angela Milner, Grzegorz Niedzwiedzki, Alec Panchen, Colin Patterson, Stephanie Pierce, Alex Ritchie, Sophie Sanchez, Hans-Peter Schultze, Paul Tafforeau, Kate Trinajstic, Emilia Vorobyeva, Stan Wood, Min Zhu and Ivars Zupins. Rob Clack has been a good friend, discussion partner and much-valued occasional fieldwork collaborator over the course of three decades. My research is funded by a Wallenberg Scholarship from the Knut and Alice Wallenberg Foundation.