Table of Contents
Preamble
-
Introduction
-
Methodology and Evidence Review
-
Organization of the Writing Committee
-
Document Review and Approval
-
Health Policy Objectives
-
Top 10 Take-Home Messages
-
-
Permanent Pacemakers
-
Introduction
-
Isolated Sinus Node Dysfunction
-
Isolated Congenital Complete Atrioventricular Block
-
Atrioventricular Block: Other Considerations
-
Postoperative Atrioventricular Block
-
Congenital Heart Disease: Specific Considerations
-
Post Cardiac Transplantation
-
Neuromuscular Diseases and Other Progressive Conduction Diseases
-
Neurocardiogenic Syncope
-
Cardiac Channelopathies
-
Inflammation/Infection
-
-
Implantable Cardioverter Defibrillators
-
Introduction
-
General Recommendations for ICD Therapy
-
ICD Indications for Cardiac Channelopathies
-
Long QT Syndrome
-
Catecholaminergic Polymorphic Ventricular Tachycardia
-
Brugada Syndrome
-
-
ICD Indications for Cardiomyopathies
-
Hypertrophic Cardiomyopathy
-
Restrictive Cardiomyopathy
-
Arrhythmogenic Cardiomyopathies
-
Nonischemic Dilated Cardiomyopathies
-
-
ICD indications for Congenital Heart Disease
-
-
Insertable Cardiac Monitors
-
CIED Lead Management
-
CIED Follow-up and Ancillary Testing
-
Special Considerations
-
CIEDs and Magnetic Resonance Imaging
-
CIEDs and Sports Participation
-
CIEDs in Low- and Middle-Income Countries
-
Shared Decision-Making
-
-
Knowledge Gaps and Future Research
-
References
-
Appendix 1 Author Relationships With Industry
-
Appendix 2 Reviewer Relationships With Industry
This article has been copublished in Heart Rhythm, JACC: Clinical Electrophysiology, Indian Pacing and Electrophysiology Journal, and Cardiology in the Young.
Preamble
Guidelines for the implantation of cardiovascular implantable electronic devices (CIEDs) have evolved since the initial American College of Cardiology (ACC)/American Heart Association (AHA) pacemaker guidelines in 1984. Reference Frye, Collins and DeSanctis1 CIEDs have evolved to include novel forms of cardiac pacing, the development of implantable cardioverter defibrillators (ICDs), and the introduction of devices for long-term monitoring of heart rhythm as well as other physiologic parameters. In view of the increasing complexity of both devices and patients in the current era, practice guidelines, by necessity, have become increasingly specific. One aspect of this evolution is the “2018 ACC/AHA/HRS Guideline on the Evaluation and Management of Patients With Bradycardia and Cardiac Conduction Delay,” Reference Kusumoto, Schoenfeld and Barrett2 which included specific recommendations for patients >18 years of age. This age-specific threshold was established in view of the need for differing indications for CIEDs as well as size-specific technology factors in younger patients. Therefore, this document has been developed to update and further delineate indications for the use and management of CIEDs in pediatric patients, defined as ≤21 years of age, in recognition that there is often overlap in the care of patents between 18 and 21 years of age.
This document is an expert consensus statement intended to focus primarily on the indications for CIEDs in the setting of specific disease/diagnostic categories. This consensus statement will also provide guidance regarding the management of CIEDs for rhythm disorders in pediatric patients and address some of the deterrents to CIED access in low- and middle-income countries and strategies to circumvent them.
Recommendations are presented in a modular or knowledge chunk format, in which each section includes a table of recommendations, a brief synopsis, and recommendation-specific supportive text. Reference Levine, O’Gara and Beckman3 However, this document is not intended to provide an exhaustive review of all aspects of pacemakers, ICDs, and insertable cardiac monitors (ICMs), as this information is easily accessible in electronic searches or textbooks. Furthermore, specific recommendations, such as heart rate criteria for pacemaker implantation, even when supported by substantial data, do not replace the need for clinical judgment and patient-specific decision-making. As a final introductory comment, to avoid clinical overlap, the indications and management of cardiac resynchronization therapy and physiological pacing will be addressed in the anticipated “2022 HRS Expert Consensus Statement on Cardiac Physiological Pacing for the Avoidance and Mitigation of Heart Failure,” which will include a specific section on pediatric and congenital heart disease (CHD).
Introduction
Methodology and evidence review
The principles in the development of this document are 1) new recommendations and any changes to previous recommendations are based on data, when possible; 2) these recommendations are consistent with current ACC/AHA/Heart Rhythm Society (HRS) guidelines when reasonable; Reference Kusumoto, Schoenfeld and Barrett2–Reference Indik, Gimbel and Abe19 and 3) all recommendations are critically reviewed, initially by the writing committee and editors, followed by the Pediatric and Congenital Electrophysiology Society (PACES) executive committee, and subsequently by external HRS, ACC, AHA, and Association for European Paediatric and Congenital Cardiology (AEPC) representatives. Any revisions or additions to existing recommendations will require approval of at least 80% by the members of the PACES writing committee. Specific prior guidelines and consensus statements relevant to CIEDs that have been referenced as the basis for recommendations in this document are acknowledged below and recognized in the specific sections (Table 1).
Table 1. Guidelines, expert consensus statements, and reports Cited
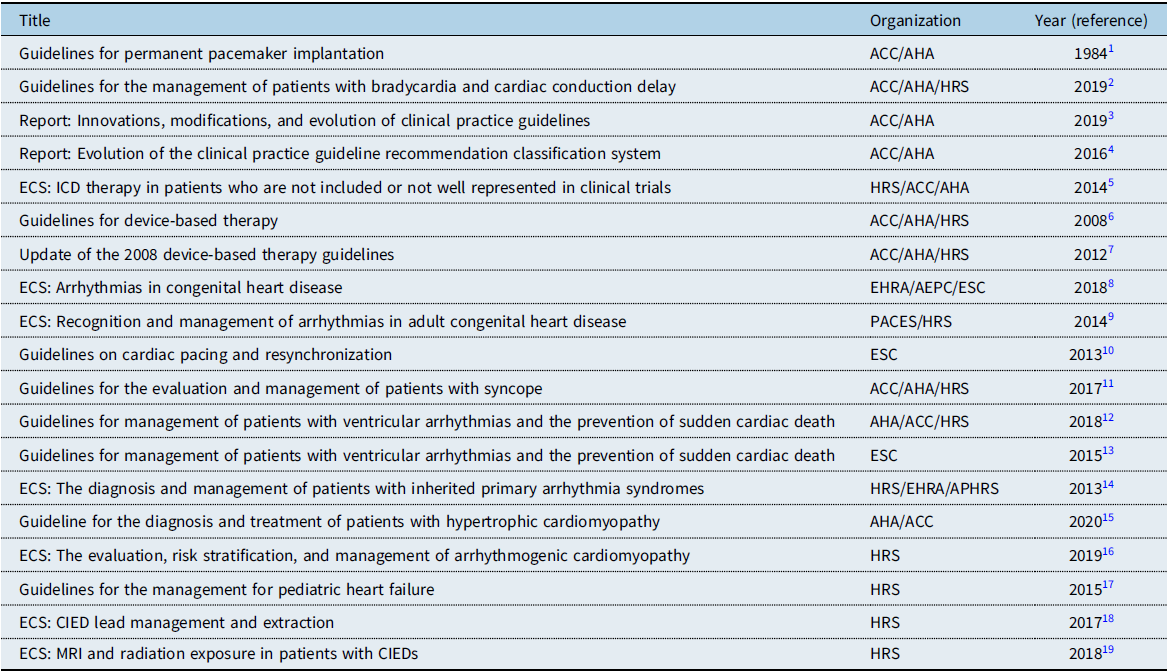
ECS = expert consensus statements; EHRA = European Heart Rhythm Association; ESC = European Society of Cardiology.
These recommendations have been developed consistent with standard guideline methodology, i.e., with both a class of recommendation (COR) and a level of evidence (LOE) (Table 2). Reference Halperin, Levine and Al-Khatib4 The class of the recommendation indicates the strength of recommendation, based on the estimated magnitude or certainty of benefit in proportion to risk. The level of evidence rates the quality of scientific evidence supporting the intervention on the basis of the type, quantity, and consistency of data from clinical trials and other sources. Due to the lack of randomized clinical trials (RCTs) in pediatric patients, these LOE recommendations will be limited to class B-NR (limited populations), class C-LD (very limited populations), or C-EO (consensus expert opinion, case studies, or standard of care). It is important to emphasize that a recommendation with a level of evidence C-EO does not imply that the recommendation is weak. Many of the questions addressed in this (and other) documents either do not lend themselves to clinical trials or are rare disease entities. Reference Kusumoto, Calkins and Boehmer5 However, there may be unequivocal expert consensus that a particular intervention is either effective or necessary. The final evidence tables for the recommendations are included in Supplemental Appendix 3 and summarize the evidence used by the writing committee to formulate these recommendations. References selected and published in this document are intended to be representative and not all-inclusive. Variations between previously published adult and pediatric CIED recommendations as well as new pediatric-specific recommendations are listed in Supplemental Appendix 4.
Table 2. Class of Recommendation and Level of Evidence Categories*

*Adapted from Halperin, et al.Reference Halperin, Levine and Al-Khatib4
Organization of the writing committee
The writing committee consisted of members of PACES who were selected by the PACES executive committee. The writing committee members included junior and senior pediatric electrophysiologists as well as allied health professionals and represented diverse genders, countries, and cultures. The writing committee also included external representatives from the ACC, AHA, HRS, and AEPC. Prior to final publication, all committee members were required to verify their specific contributions to this document. Appendix 1 lists writing committee members’ relevant relationships with industry.
Document review and approval
Following internal review by the PACES executive committee, this document was then reviewed by the PACES writing committee. Following considerations of these comments and approval by an independent PACES reviewer, the recommendations were opened for public comment to PACES members. An official reviewer each nominated by HRS, ACC, AHA, and AEPC provided independent external review. This document was then approved for publication by the PACES executive committee and endorsed by all collaborators and the Asia Pacific Heart Rhythm Society (APHRS), the Indian Heart Rhythm Society (IHRS), and the Latin American Heart Rhythm Society. Appendix 2 lists reviewers’ relevant relationships with industry.
Health policy objectives
The purpose of this document is to provide guidance to clinicians for the management of pediatric patients who may require a CIED, with a primary focus on the indications for device implantation. The document will be useful to pediatric cardiologists, cardiac surgeons, cardiac intensivists, anesthesiologists, and arrhythmia specialists. This document supersedes the pediatric CIED recommendations made in “ACC/AHA/HRS 2008 Guidelines for Device-Based Therapy of Cardiac Rhythm Abnormalities” Reference Epstein, Dimarco and Ellenbogen6 and “2012 ACCF/AHA/HRS Focused Update of the 2008 Guidelines for Device-Based Therapy of Cardiac Rhythm Abnormalities.” Reference Tracy, Epstein and Darbar7 This document is expected to provide support for clinicians and patients to allow for appropriate device use, appropriate device management, follow-up, and appropriate reimbursement in pediatric patients.
Top 10 take-home messages
-
1. In patients with isolated sinus node dysfunction (SND), there is no minimum heart rate or maximum pause duration where permanent pacing is absolutely recommended. Establishing a temporal correlation between symptoms and bradycardia is critical in the decision as to whether permanent pacing is indicated.
-
2. Young patients with impaired ventricular function or abnormal cardiovascular physiology may be symptomatic due to sinus bradycardia or the loss of atrioventricular (AV) synchrony at heart rates that do not produce symptoms in individuals with normal cardiovascular physiology.
-
3. Although the average ventricular rate in newborns and infants with congenital complete atrioventricular block (CCAVB) provides an objective measure regarding the decision for pacemaker implantation, additional factors may equally influence the decision/timing of pacemaker implant. These include birth weight (size), congenital heart defects, ventricular function, and other comorbidities.
-
4. In patients with postoperative AV block, a period of observation for at least 7–10 days before pacemaker implantation remains advised; in select cases, earlier pacemaker implantation may be considered if AV block is not expected to resolve due to extensive injury to the cardiac conduction system.
-
5. Atrial pacing with antitachycardia pacing capabilities is reasonable for CHD patients with recurrent intra-atrial reentrant tachycardia when medication and catheter ablation are not effective.
-
6. There is increased recognition of the need for pacemaker implantation in conditions such as Kearns-Sayre syndrome or certain neuromuscular disorders due to the unpredictable progression of conduction disease.
-
7. The cause of sudden cardiac arrest (SCA) remains undefined in nearly 50% of pediatric survivors. ICD implantation is recommended provided completely reversible causes have been excluded, other treatments that may be beneficial are considered, and meaningful survival is anticipated.
-
8. The decisions for implantation of an ICD for primary prevention in cardiac channelopathies or cardiomyopathies remain guided by limited and, at times, conflicting data. Consideration of patient-specific factors and shared decision-making are critically important.
-
9. In pediatric patients with nonischemic dilated cardiomyopathy (NIDCM), primary prevention ICD implantation for left ventricular ejection fraction (LVEF) 35%, in the absence of other risk factors, is not clearly supported by published data.
-
10. In patients with indications for implantation of a CIED, shared decision-making and patient/family-centered care are endorsed and emphasized. Treatment decisions are based on the best available evidence and patient’s preferences.
Permanent pacemakers
Introduction
The most common indications for permanent pacemaker implantation in children, adolescents, and patients with CHD may be classified as 1) symptomatic sinus bradycardia, 2) advanced second- or third-degree AV block, either congenital or acquired, and 3) pacing for the prevention or termination of tachyarrhythmias. Reference Epstein, Dimarco and Ellenbogen6 In general, many of the indications for pacemaker implantation in children and adolescents (defined as <19 years of age) are similar to those in adults. Reference Kusumoto, Schoenfeld and Barrett2 However, there are several important differences in infants and children. These patients have faster heart rates, and therefore standards for what is considered normal are age-dependent variables; whereas a heart rate of 45 bpm may be a normal in an adolescent, the same rate in a newborn or infant indicates profound bradycardia. In addition, young patients with impaired ventricular function or abnormal physiology may be symptomatic due to sinus bradycardia or loss of AV synchrony at heart rates that do not produce symptoms in individuals with normal cardiovascular physiology. Reference Hernández-Madrid, Paul and Abrams8,Reference Khairy, Van Hare and Balaji9 Hence, the indications for pacemaker implantation in young patients need to be based on the correlation of symptoms with relative bradycardia rather than absolute heart rate criteria.
Significant technical challenges may complicate device and lead implantation in small patients or those with abnormalities of venous or intracardiac anatomy. Epicardial pacemaker lead placement and use of device technology in innovative ways often need to be considered to provide pacing in the youngest patients. Reference Chang, Carter, Bar-Cohen, Shah, Rhodes and Kaltman20–Reference Moore and Shannon22 Any pacemaker system used in a young patient may need to be utilized for multiple decades, and consideration of the long-term consequences from device and lead failure plays a role in implantation of pediatric devices.
Bradycardia and associated symptoms in children are often transient (e.g., breath-holding spells) and therefore may not require permanent pacemaker therapy. Conversely, there are other conduction system disorders that may rapidly progress (e.g., neuromuscular disorders) that may require prophylactic pacemaker implantation for disease-specific indications. In addition, as risk factors for cardiac conditions such as the channelopathies are better defined, the indications for device placement in these patients may evolve rapidly.
The goal of this section is to provide an update regarding the indications for permanent pacemaker implantation in pediatric patients. A summary of the recent literature is provided as a framework for clinicians to make individual decisions about pacing in these patients. As the pediatric and CHD populations represent unique groups of patients, clinical judgment and patient-specific decision-making are of the highest importance.
Isolated sinus node dysfunction

Recommendation-specific supportive text
SND refers to physiologically inappropriate atrial rates, due to either sustained bradycardia or abrupt pauses in the intrinsic cardiac rhythm. In patients with isolated sinus bradycardia without symptoms due to cerebral or systemic hypoperfusion, there is no minimum heart rate or maximum pause duration where permanent pacing is recommended. Establishing a temporal correlation between symptoms and age-related bradycardia is of paramount importance when determining whether permanent pacing is needed.
Nonrandomized studies in both children and adults have demonstrated that pacing can provide symptomatic improvement when symptoms, particularly syncope and pre-syncope, are clearly attributable to SND. Reference Breivik, Ohm and Segadal23–Reference Chiu, Lin and Wang26 However, there is no clear evidence that pacing in the setting of isolated SND without symptoms improves outcomes.
In symptomatic patients with SND, atrial-based pacing is generally recommended over single-chamber ventricular pacing. Reference Kusumoto, Schoenfeld and Barrett2,Reference Kardelen, Celiker, Ozer, Ozme and Oto28 Furthermore, the decisions regarding pacemaker implantation for SND in patients with CHD or channelopathies should be made on an individualized basis and are discussed further in the corresponding sections. Reference Gillette, Wampler and Shannon29
Isolated congenital complete
Atrioventricular block

Recommendation-Specific Supportive Text
Although the average ventricular rate in newborns (≤30 days old) and infants (≤12 months old) with isolated CCAVB provides an objective measure regarding the decision for pacemaker implantation, additional factors may equally influence the decision/timing of pacemaker implant. These include birth weight (size), ventricular dysfunction, and other comorbidities. Reference Glatz, Rhodes and Gayno42 Furthermore, although symptoms such as poor feeding or tachypnea in the neonate may be due to multiple causes, they may be indicative of low cardiac output secondary to bradycardia. Therefore, a lower limit heart rate of 50 bpm is recommended for pacemaker implantation when overt symptoms related to low cardiac output do not appear to be present. One additional point of emphasis is that use of heart rate criteria for newborn or infant pacing should be based on heart rate consistency rather than a single measurement in time. Reference Michaëlsson and Engle34,Reference Pinsky, Gillette and Garson37
Beyond the first year of life, permanent pacemaker implantation is generally indicated in symptomatic patients. Contemporary studies suggest that approximately 66% of neonates and infants diagnosed with isolated CCAVB will undergo pacemaker implantation during their first year of life and that 90% of patients with CCAVB will undergo pacemaker implantation by 20 years of age. Reference Jaeggi, Hamilton and Silverman30 Long-term natural history studies have demonstrated progressive left ventricular dysfunction and mitral insufficiency with cardiovascular mortality in the fourth or fifth decade of life in patients with CCAVB who did not undergo pacemaker implantation. Reference Michaëlsson and Engle33,Reference Michaëlsson and Engle34,Reference Moak, Barron and Hougen43 On the other hand, some patients with CCAVB will develop left ventricular cardiomyopathy despite pacing due to either antibody-mediated myocarditis or pacing-induced dyssynchrony. Reference Moak, Barron and Hougen43,Reference Janoušek, van Geldorp and Krupičková44
Atrioventricular block: other considerations

Recommendation-specific supportive text
The diagnosis of advanced AV block during late childhood or adolescence is an uncommon but well-recognized phenomena. Advanced AV block may be congenital, may be related to infiltrative diseases, or may remain idiopathic. At times, late-onset AV block may be paroxysmal and quite difficult to document. Reference Silver, Pass and Hordof49
Exercise stress testing can be useful to detect the site and significance of AV block. Generally, supra-His block resolves with exercise by increased sympathetic tone. When second- and third-degree degree AV block are observed during exercise, conduction disturbance within the His-Purkinje system is suspected. Although progression to advanced second- and third-degree AV block during exercise is rare, it is associated with a poor prognosis in the absence of a pacemaker. Reference Yandrapalli, Harikrishnan, Ojo, Vuddanda and Jain47,Reference Bonikowske, Barout, Fortin-Gamero, Lara, Kapa and Allison48
With the exception of infiltrative or inflammatory causes of advanced AV block, the criteria for pacemaker implantation are similar to those for CCAVB. Permanent pacemaker implantation may be considered for advanced idiopathic AV block in adolescents with an acceptable ventricular rate, a narrow QRS complex, and normal ventricular function, based on an individualized consideration of symptoms and the risk/benefit ratio.
Postoperative atrioventricular block

Recommendation-specific supportive text
Postoperative AV block complicates 3–8% of congenital heart surgeries, with 1–3% of patients requiring permanent pacemaker implantation for persistent postoperative AV block. Reference Anderson, Czosek and Knilans56–Reference Liberman, Pass and Hordof58 A very poor prognosis has been established for CHD patients with permanent postoperative AV block who do not receive permanent pacemakers. Reference Krongrad54,Reference Villain, Ouarda and Beyler55 Among patients who do regain AV conduction following a period of transient AV block, ≥85% have recovery of AV conduction by postoperative day 7 and ≥95% AV conduction by postoperative day 10. Reference Weindling, Saul and Gamble50,Reference Romer, Tabbutt and Etheridge51 Although patients who spontaneously regain AV conduction have a favorable prognosis, Reference Tracy, Epstein and Darbar7 there is a small but definite risk of late-onset complete AV block in transient postoperative AV block patients, with onset occurring as early as months, to as late as decades, following surgery. Reference Aziz, Serwer and Bradley52,Reference Krongrad54,Reference Villain, Ouarda and Beyler55 Limited data suggest that some patients with a history of transient postoperative advanced second- or third-degree AV block may be at risk for late-onset AV block or sudden cardiac death (SCD) if they have postoperative bifascicular block on the electrocardiogram (ECG) that was not present preoperatively. Reference Krongrad54,Reference Villain, Ouarda and Beyler55 Permanent pacemaker implantation may also be considered for transient postoperative third-degree AV block that reverts to intact AV node conduction when there is concern about the late development of AV block in patients with forms of CHD associated with progressive conduction abnormalities such as discordant AV connections, AV septal defects, and heterotaxy syndromes. Reference Huhta, Maloney and Ritter59,Reference Moore and Aboulhosn60
Congenital heart disease: specific considerations
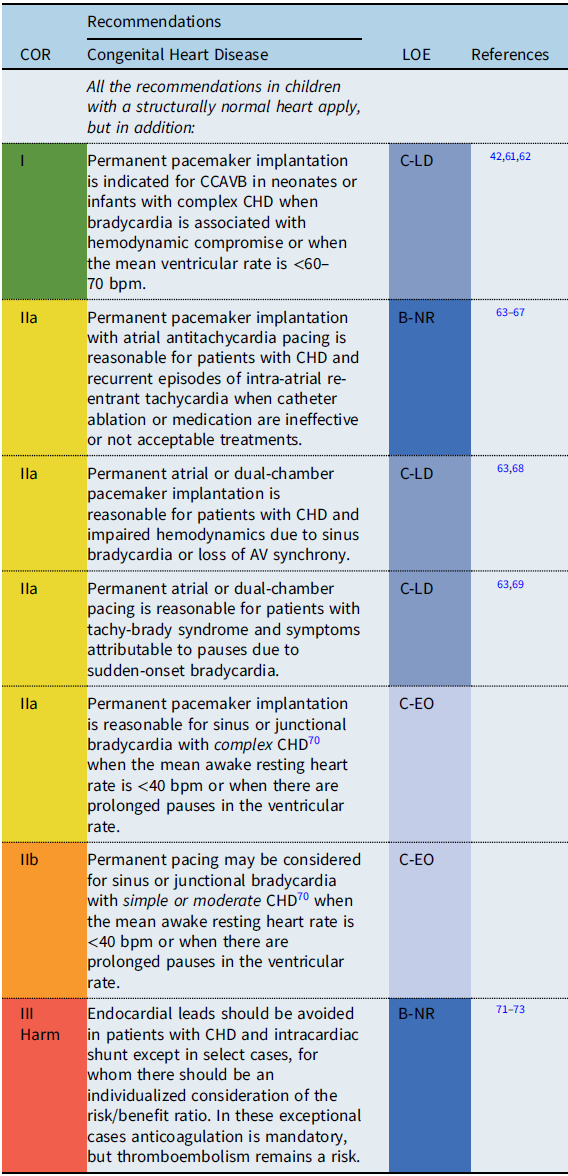
Recommendation-specific supportive text
Patients with CHD often have important structural and functional lesions, Reference Stout, Daniels and Aboulhosn70 which influence both the indications for pacing as well as the type of pacing lead(s) utilized. Reference Glatz, Rhodes and Gayno42 Therefore, pacemaker implantation in these patients should not be viewed as an isolated procedure. The loss of vascular access or direct access to cardiac chambers and/or persistent right-to-left shunting require utilization of epicardial pacing leads (with concomitant sternotomy or thoracotomy), Reference Lau, Gaynor and Fuller74 although novel hybrid approaches to lead placement are being developed. Reference Termosesov, Kulbachinskaya and Polyakava75,Reference Clark, Kumthekar and Mass76
Bradycardia and scar-related tachycardias are common following surgery, and in the absence of high-grade AV block, atrial pacing is preferred to avoid pacing-induced ventricular dysfunction. Reference Tsao, Deal and Backer67,Reference Barber, Batra and Burch68 Permanent pacemaker and/or lead implantation may be considered prophylactically in patients with evidence of conduction disease and heart defects with a known natural progression to advanced heart block (e.g., discordant AV connections, heterotaxy syndrome) at the time of cardiac surgery. Reference Huhta, Maloney and Ritter59,Reference Moore and Aboulhosn60,Reference Cohen, Rhodes and Spray77
Similarly, in single-ventricle patients undergoing Fontan conversion, prophylactic antitachycardia pacemakers have been used. Reference Tsao, Deal and Backer67 There may be a role for pacing in improving the hemodynamic status in patients with plastic bronchitis and protein-losing enteropathy without conventional pacing indications. Reference Rychik, Atz, Celermajer and Deal78
The decisions regarding pacemaker implantation should also consider the complexity of the patient’s anatomy and hemodynamic status, with complex defined as patients with palliative repairs or impaired ventricular function or circulatory physiology. Reference Stout, Daniels and Aboulhosn70
Post cardiac transplantation
Recommendation-specific supportive text

Transient sinus bradycardia is relatively common immediately after transplantation and frequently resolves spontaneously. In rare cases, sinus bradycardia may persist and pacemaker implantation may be needed, but at least a week should be allowed for spontaneous recovery of sinus node function. Early post-transplant AV block has been reported in pediatric patients to be more frequent than in the adult population and may be related to donor age. Reference Kertesz, Towbin and Clunie79,Reference El-Assaad, Al-Kindi and Oliveira80 An analysis of the United Network for Organ Sharing (UNOS) database reported that between 1994 and 2014, 1% of cardiac transplant patients <18 years of age required a pacemaker in the acute post-transplant interval. Factors associated with need for pacemaker implant were biatrial anastomosis, older donor age, and antiarrhythmic drug use. Reference El-Assaad, Al-Kindi and Oliveira80
Late-onset conduction disorders (sinus node or AV node dysfunction) may be related to cardiac allograft vasculopathy or allograft rejection. Patients should be evaluated for the presence or development of transplant coronary artery disease, as late-onset bradycardia may be the first manifestation. Reference Kertesz, Towbin and Clunie79,Reference Cannon, Denfeld and Friedman84 Microvascular angiopathy that may not be seen during conventional angiography may also cause significant ventricular dysfunction and subsequent graft failure with an added risk for conduction abnormalities. Reference Chang, Hruban and Levin85
The role of prophylactic ICD implantation is not well established but may be considered in patients who require pacemakers. Risk factors to consider are coronary artery vasculopathy and left ventricular dysfunction, which may present as ventricular arrhythmias and have been associated with SCD. Reference Daly, Chakravarti and Tresler86,Reference Carboni87
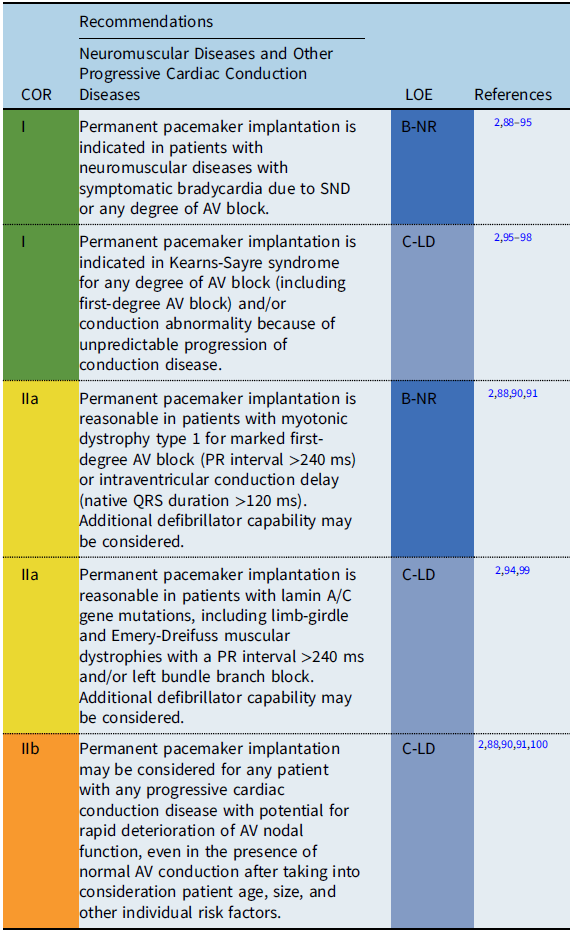
Conditions include Duchenne muscular dystrophy, Becker muscular dystrophy, myotonic dystrophy type 1, Friedreich ataxia, Emery-Dreifuss muscular dystrophy, facioscapulohumeral muscular dystrophy, Barth syndrome, Kearns-Sayre syndrome, lamin A/C mutations, and desmin-related myopathies.
Neuromuscular diseases and other
Progressive cardiac conduction diseases
Recommendation-specific supportive text
Progressive cardiac conduction diseases often involve genetic disorders with progressive deterioration of the conduction system occurring either in isolation or in conjunction with other cardiac and metabolic diseases including neuromuscular and mitochondrial diseases.
The severity and onset of cardiac complications differ among the diseases. Conduction disturbances are commonly observed in myotonic dystrophy type 1 and Emery-Dreifuss muscular dystrophy. Reference Jones, Mortsell and Rajaruthnam81 Variable degrees of conduction abnormalities may occur, ranging from first-degree AV block to complete AV block with unpredictable progression. Laminopathy caused by mutations in the LMNA gene is a wide-spectrum disorder exhibiting peripheral neuropathy, skeletal muscle disorders, progerias, and dilated cardiomyopathy. Cardiac conduction abnormalities, such as sinus bradycardia, AV block, atrial fibrillation, atrial standstill, and ventricular tachycardia (VT), are common and are often observed before the onset of heart failure symptoms. Reference Carboni87,Reference Groh, Groh and Saha92 In a meta-analysis, arrhythmias were observed in 36% of patients before 20 years of age, with heart failure observed in 10% before 30 years of age. Reference Carboni87 A prolonged PR interval >240 ms in adults is reported to be a predictor of progressive AV block and/or ventricular arrhythmias in patients with myotonic dystrophy and in patients with laminopathy. Reference Ha, Tarnopolsky and Bergstra91,Reference Groh, Groh and Saha92,Reference Van Berlo, de Voogt and van der Kooi94,Reference Hasselberg, Edvardsen, Petri and Berge99
Among the mitochondrial diseases, patients with Kearns-Sayre syndrome, characterized by progressive external ophthalmoplegia and myopathy with an onset before the age of 20 years, are known to carry a high risk for AV block and SCD. Reference Feingold, Mahle and Auerbach88–Reference Ha, Tarnopolsky and Bergstra91 Currently, an HRS expert consensus statement on the evaluation and management of arrhythmic risk in neuromuscular disorders is under development. Therefore, the above recommendations may be subject to modification as newer data become available.
Neurocardiogenic syncope

Recommendation-specific supportive text
In the vast majority of cases, neurocardiogenic syncope is a limited disease and pacemaker implantation is not required. In some patients, however, recurrent syncopal events may significantly impair quality of life and may result in traumatic injury, particularly when the dominant feature of reflex syncope is cardioinhibitory. Reference Kolterer, Gebauer, Janousek, Dähnert, Riede and Paech101–Reference Brignole, Menozzi and Moya104,Reference Bestawros, Darbar, Arain, Abou-Khalil, Plummer, Dupont and Rah108 Therefore, in a highly select group of patients who fail more conservative treatment options, pacemaker therapy may be useful by preventing profound bradycardia or prolonged asystole. Because the efficacy of pacing depends on the clinical setting, a clear relationship between symptoms and bradycardia should be established prior to pacemaker implantation. Bradycardia or asystole should be observed during episodes of clinical syncope, ideally on more than one occasion. Reference Paech, Wagner, Mensch and Antonin Gebauer105 Event monitors and ICMs have been effective for documenting this relationship.
In pallid breath-holding spells, studies of predominantly infants and toddlers have demonstrated either complete resolution or a significant reduction in the number of syncopal events in 86% patients with pacing. Reference Kolterer, Gebauer, Janousek, Dähnert, Riede and Paech101,Reference McLeod, Wilson, Hewitt, Norrie and Stephenson102 Single-chamber pacing with hysteresis appears as effective as dual-chamber pacing with rate drop response for the prevention of syncope and seizures. Pacemaker settings may be optimized to prevent sustained bradycardia by programming a relatively fast pacing rate at the time of the vasovagal reflex to augment cardiac output.
Attributed to vagal storm in the setting of epilepsy, ictal-induced bradyarrhythmia or asystole can impair both cerebral perfusion and cortical function and contribute to transient loss of consciousness and injury. Reference Sutton, de Jong and Stewart106,Reference Benditt, van Dijk and Thijs107 While conventional antiepileptic medications and epilepsy surgery are the mainstay treatments for ictal-induced bradycardia, pacemaker implantation may be reasonable as an adjunct for reducing the severity of symptoms.
Cardiac channelopathies
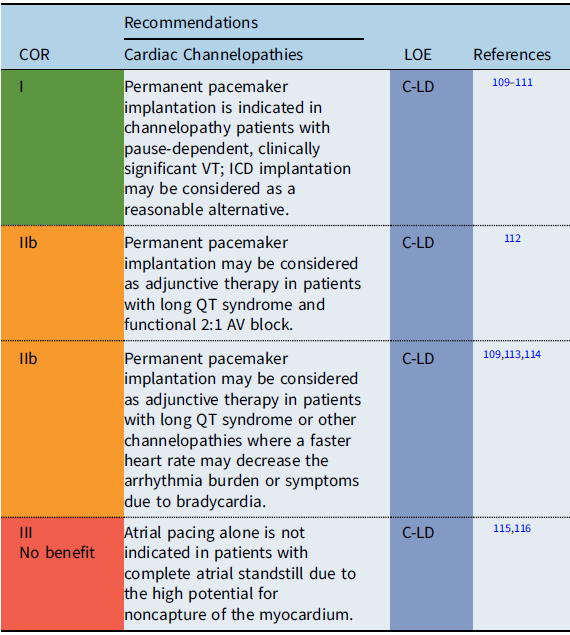
Recommendation-specific supportive text
The utility of pacing as adjunctive therapy in the various channelopathies is not well defined. Most data are based on observational reports of pacing in the context of long QT syndrome (LQTS). In certain high-risk patients with LQTS, permanent pacemaker implantation may provide a benefit to decrease bradycardia-related or pause-related initiation of ventricular tachyarrhythmias or so-called short-long-short episodes. Reference Moss, Liu and Gottlieb109–Reference Viskin, Fish and Zeltser111 In infants with prolonged QT-related functional 2:1 AV block, one observational study reported that pacing in combination with other therapies resulted in favorable outcomes with no mortality. Reference Aziz, Tanel and Zelster112 Additionally, in some patients with LQTS, atrial pacing faster than the intrinsic rate has been shown to shorten the QT interval and reduce the rate of recurrent syncopal events in high-risk LQTS patients. Reference Moss, Liu and Gottlieb109,Reference Kowlgi, Giudicessi and Brake114 When SND and/or AV block are present in the setting of a channelopathy or as the result of antiarrhythmic medications needed for treatment of a channelopathy, the indications for permanent pacing detailed in the respective section on SND and/or AV block apply. In the setting of atrial standstill secondary to a channelopathy or laminopathy, single-chamber atrial pacemaker placement alone is not recommended due to the high probability of atrial noncapture. Reference Bellmann, Roser and Muntean115,Reference Ishikawa, Tsuji and Makita116
Inflammation/infection

Recommendation-specific supportive text
Systemic infections may cause myocardial inflammation or infiltration presenting with bradycardia or complete AV block. Known causes are Lyme disease (Borrelia burgdorferi), Chagas disease in individuals from Trypanosoma cruzi–endemic areas in Central and South America, and rarely from diphtheria myocarditis. Other etiologies include infectious mononucleosis (Epstein-Barr virus), bacterial endocarditis, viral myocarditis with perivalvular abscess, rheumatic fever, and sarcoidosis.
In symptomatic AV block associated with Lyme disease, approximately 40% of patients may require temporary pacing, although AV block is typically reversible with antibiotic therapy. Reference McAlister, Klementowicz and Andrews117,Reference Forrester and Mead118 Chronic Chagas disease can present with different degrees of conduction defects. Advanced heart block in Chagas is permanent, and pacemaker implantation is indicated. Reference Nunes, Beaton and Acquatella119,Reference Bocchi, Bestetti and Scanavacca120 An ICD should be considered in Chagas cardiomyopathy in the presence of significant left ventricular dysfunction or ventricular arrhythmias. Reference Bocchi, Bestetti and Scanavacca120 More recently, there have been reports of transient AV conduction abnormalities associated with the COVID-19–related multisystem inflammatory syndrome in children (MIS-C) with ventricular dysfunction. Reference Dionne, Mah and Son121 Medical-directed therapy for the underlying condition should be maximized (including antibiotic therapy, steroids, intravenous immunoglobulins), and if tolerated, a waiting period of up to several months is warranted prior to pacemaker implantation to provide sufficient opportunity for spontaneous recovery of AV conduction.
Recovery of AV conduction in patients with complete heart block due to acute myocarditis has been reported to occur in 67% of young patients within 7 days of the onset of AV block. Reference Batra, Epstein and Silka122 Late monitoring for possible recurrence of symptoms or unrecognized recurrences of AV block or other arrhythmias is advised in these patients.
Implantable cardioverter defibrillators
Introduction
The process of CIED guideline development has evolved over the past few decades, with initial recommendations based on observational clinical experience and refined based on controlled clinical studies and advances in device technology. Although the development of pediatric CIED recommendations has been limited by the lack of RCTs and small patient numbers, pacemaker recommendations have been established based on clearly defined diagnoses and five decades of clinical experience. Conversely, pediatric recommendations for ICD implantation have been primarily based on adult data and, with some modifications, applied to younger patients. Adult ICD guidelines are based on a specific diagnosis as the defined cause or presumed risk factor for a sudden cardiac event, such as ischemia, cardiomyopathy, or genetic cardiovascular disease. Reference Epstein, Dimarco and Ellenbogen6,Reference Tracy, Epstein and Darbar7,Reference Al-Khatib, Stevenson and Ackerman12,Reference Priori, Blomström-Lundqvist and Mazzanti13 In contrast, recent studies of pediatric SCA survivors have continued to demonstrate that in approximately 50% of cases, the cause of the event remains undefined despite an extensive and systematic evaluation. Reference Cunningham, Roston and Franciosi123,Reference Rucinski, Winbo and Marcondes124 Furthermore, in young patients with diagnoses such as catecholaminergic polymorphic ventricular tachycardia (CPVT) or Brugada syndrome, SCA is often the presenting symptom of the disease. Reference Silka, Kobayashi and Hill125,Reference van der Werf, Lieve and Bos126 Therefore, while development of pediatric ICD recommendations based on specific cardiovascular diagnoses would be intuitively preferable, the following discussion of ICD indications will begin with general considerations for the young patient with an unexplained SCA, followed by a more nuanced series of recommendations for ICD implantation when a specific cause of SCA or defined risk factor has been identified. Furthermore, there remain extensive “gaps” in current ICD recommendations, irrespective of age, for many of the diseases associated with SCD in pediatrics. Reference Cohen, Etheridge, Shah, Rhodes and Kaltman127,Reference Minier, Probst and Berthome128 The recommendations that follow are largely based on limited clinical data or expert opinion and consensus and require the application of case-specific clinical judgment and a shared-decision approach.
General recommendations for implantable cardioverter defibrillator therapy

Recommendation-specific supportive text
ICD guidelines specific to pediatrics must consider the unique aspects of device implantation and follow-up in children as well as the pathogenesis of the disease, which may evolve over time. A pediatric cardiologist should be involved in the decision to implant an ICD in pediatric patients, and the procedure should be performed by a cardiologist or cardiothoracic surgeon with special training and/or experience in CIED implantation in the pediatric age-group. ICD implantation should be a shared decision between the patient, family, and physician considering specific pediatric characteristics including age, size of the patient, need for an epicardial device, religious/cultural beliefs, and patient quality of life. This includes the physical as well as the psychological impact of an ICD on the patient’s well-being. Reference Sears, Hazelton and St Amant137 In addition, all ICD recommendations are based on the premise that meaningful survival of >1 year is expected; meaningful survival means that a patient has a reasonable quality of life and functional status. Reference Shen, Sheldon and Benditt11 It is further recommended that the indications for an individual patient’s ICD be reconsidered at each reintervention with respect to current guidelines, especially after a period of nonuse, as discontinuation of device therapy may be considered in select cases. Reference Kini, Soufi and Deo138
ICD indications for cardiac channelopathies
Long QT syndrome

Recommendation-specific supportive text
Congenital LQTS refers to genetically heterogeneous disorders characterized by the phenotypes of QTc prolongation on the ECG and risk of potentially life-threatening cardiac arrhythmias. Both phenotypic and genotypic characteristics are used to guide risk stratification of patients with LQTS and consideration for ICD. Reference Mazzanti, Maragna and Vacanti153 Phenotypic risk factors include the onset of symptoms at age <10 years, prior SCA, or recurrent syncope. Reference Bos, Bos and Johnson143–Reference Biton, Rosero and Moss146,Reference Mazzanti, Maragna and Vacanti153 Additional high risk factors include a QTc ≥ 550 ms regardless of genotype, QTc ≥ 500 ms with LQT1 genotype, females with LQT2 genotype, and males with LQT3 genotype. Reference Wedekind, Burde and Zumhagen141,Reference Giudicessi and Ackerman150
Patients with rare conditions such as the Jervell and Lange-Nielson syndrome, Timothy syndrome, or calmodulinopathies may be at highest risk for SCA or SCD. Reference Giudicessi and Ackerman150–Reference Crotti, Spazzolini and Tester152 Infants presenting with bradycardia, functional 2:1 AV block, or cardiac arrest are also at significant risk. Reference Moore, Gallotti and Shannon155
Nonselective beta-blockers are considered first-line therapy and can significantly decrease subsequent cardiac events in patients, especially in those with KCNQ1 mutations. Reference Priori, Wilde and Horie14,Reference Vincent, Schwartz and Denjoy140 In addition, beta-blockers and cardiac sympathetic denervation without ICD may be appropriate alternatives in carefully selected patients. Reference Priori, Wilde and Horie14,Reference Schwartz, Priori and Cerrone142,Reference Bos, Bos and Johnson143
In highest-risk patients, observational studies support effectiveness of the ICD in preventing SCD, with consideration of left cardiac sympathetic denervation to reduce the frequency of ICD shocks. Reference Schwartz, Spazzolini and Priori139,Reference Schwartz, Priori and Cerrone142,Reference Bos, Bos and Johnson143 However, implantation of an ICD in asymptomatic low-risk patient with LQTS for a positive family history of LQTS-related SCD is not clearly supported by published data, and individual decision-making is important. Reference Priori, Wilde and Horie14
Catecholaminergic polymorphic ventricular tachycardia
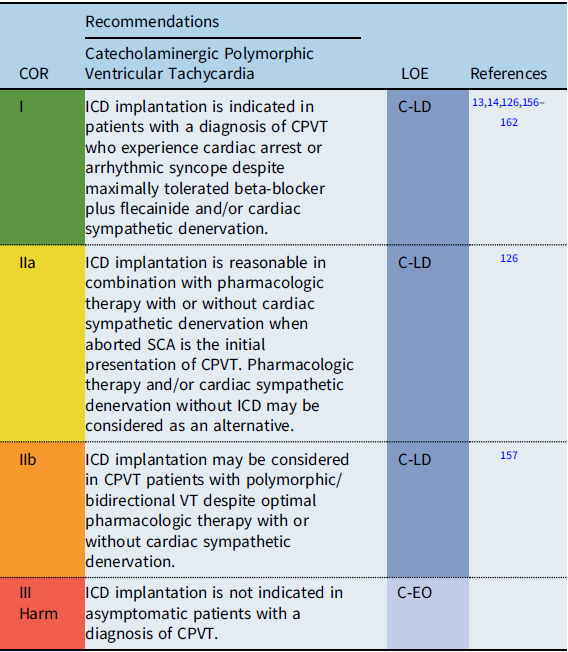
Recommendation-specific supportive text
CPVT is characterized by exertion-related polymorphic or bidirectional VT and is associated with syncope and SCA. SCA/SCD is reported in 3–13% of CPVT patients. Reference Priori, Napolitano and Memmi158 High risk factors include male sex, previous history of cardiac arrest, multiple genetic variants, and younger age at diagnosis. Reference Priori, Napolitano and Memmi158,Reference Roston, Haji-Ghassemi and LaPage159 Continued complex ventricular ectopy on exercise testing despite optimal medical therapy is also associated with worse outcome. Reference Hayashi, Denjoy and Extramiana160 Studies evaluating CPVT patients with >2 genetic variants suggest that these patients may also be at higher risk for SCA. Reference Roston, Haji-Ghassemi and LaPage159
Treatment with nonselective beta-blockers is associated with a reduction in adverse cardiac events. Reference Priori, Blomström-Lundqvist and Mazzanti13,Reference Priori, Wilde and Horie14,Reference Priori, Napolitano and Memmi158 The addition of flecainide to refractory patients in addition to maximally tolerated beta-blocker may suppress ventricular ectopy by as much as 85%. Reference Kannankeril, Moore and Cerrone161
In general, ICD implantation should be reserved for CPVT patients with prior SCA or with arrhythmogenic syncope on combination medical therapy and/or cardiac sympathetic denervation. Reference Priori, Blomström-Lundqvist and Mazzanti13,Reference Priori, Wilde and Horie14,Reference van der Werf, Lieve and Bos126,Reference Miyake, Webster and Czosek157 Inappropriate shocks are reported in 20–30% of CPVT patients with ICDs. Reference Miyake, Webster and Czosek157,Reference Olde Nordkamp, Postema and Knops163,Reference Roses-Noguer, Jarman and Clague164 Device programming in patients with CPVT should be optimized to deliver therapy for ventricular fibrillation (VF) and to minimize inappropriate shocks and the risk of potentially fatal electrical storms. Reference Miyake, Webster and Czosek157,Reference Roses-Noguer, Jarman and Clague164
Cardiac sympathetic denervation is recommended in patients who continue to have syncope or significant arrhythmias despite optimal medical therapy, are intolerant of medical therapy, or experience recurrent ICD shocks. Reference De Ferrari, Dusi and Spazzolini162 In selected patients with aborted SCA as the initial presentation of CPVT, pharmacologic therapy and/or cardiac sympathetic denervation without ICD may be considered as a possible alternative. Reference Priori, Wilde and Horie14,Reference van der Werf, Lieve and Bos126
Brugada syndrome

Recommendation-specific supportive text
Brugada syndrome (BrS) is an inherited arrhythmogenic disorder characterized by a coved-type ST-segment elevation in the right precordial ECG leads and an increased risk of SCD. Reference Al-Khatib, Stevenson and Ackerman12–Reference Priori, Wilde and Horie14,Reference Gonzalez Corcia, de Asmundis and Chierchia165 The phenotypic expression of the disease spans from patients who are completely asymptomatic to those who experience a lethal arrhythmia. Reference Gonzalez Corcia, de Asmundis and Chierchia165,Reference Gonzalez Corcia, Sieira and Sarkozy166 The syndrome presents typically in the fourth to fifth decade, but in rare cases may have an early onset during childhood. Reference Moore, Gallotti and Shannon155 Pediatric cases are rare but can express as a rapidly progressive form and lead to life-threatening arrhythmias. Reference Minier, Probst and Berthome128,Reference Gonzalez Corcia, Sieira and Sarkozy166–Reference Michowitz, Milman and Andorin169
The placement of an ICD remains the only therapy with proven efficacy for the management of ventricular arrhythmias and prevention of SCD in patients with BrS. Reference Gonzalez Corcia and Sieira170 Adult recommendations for risk stratification including ventricular stimulation have been established but have not been validated in pediatrics. Reference Al-Khatib, Stevenson and Ackerman12–Reference Priori, Wilde and Horie14 Findings associated with high risk of ventricular arrhythmias and SCD in children include, in order of relevance: the presence of symptoms (SCD or arrhythmogenic syncope), spontaneous coved-type ST elevation (type I pattern) ECG, atrial arrhythmias and/or SND, and conduction abnormalities (AV block or intraventricular conduction delay). Reference Gonzalez Corcia, de Asmundis and Chierchia165 Although attempts have been made to create a noninvasive risk stratification scoring system, Reference Gonzalez Corcia, Sieira and Pappaert167 such recommendations are based on small cohorts. Patients with a type I ECG pattern and a history of syncope or SCD have a class I indication for an ICD implantation. Reference Hayashi, Denjoy and Extramiana160 In this study, 9 of 35 (26%) BrS patients with an ICD implanted at age <20 years received an appropriate therapy during a median follow-up of 7.3 years. Reference Hayashi, Denjoy and Extramiana160 Conversely, implantation of an ICD is not indicated in asymptomatic patients in the absence of risk factors. Large multicentric studies are necessary to further characterize risk factors and support primary prevention indications for BrS in pediatric patients.
ICD indications for cardiomyopathies
Hypertrophic cardiomyopathy
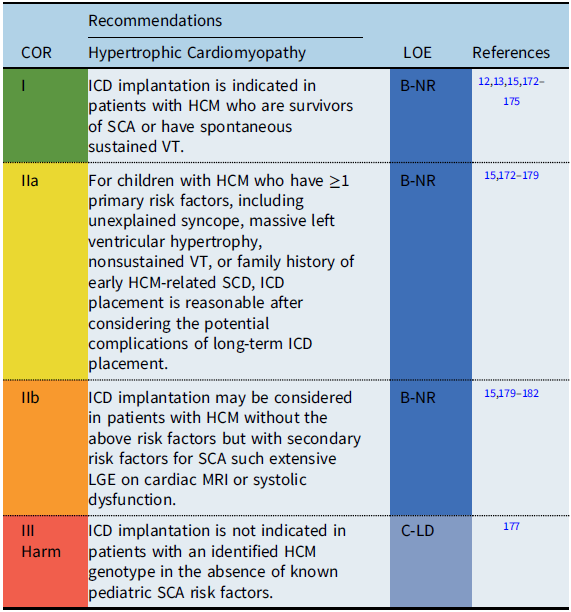
Recommendation-specific supportive text
Hypertrophic cardiomyopathy (HCM) is a genetic cardiovascular condition manifested by pathologic left ventricular hypertrophy in the absence of loading conditions. The phenotypic expression of HCM is variable, resulting in a diverse clinical course and highly variable long-term prognosis. Estimates for SCD rates in childhood HCM vary widely, with recent epidemiologic studies that have reported rates of between 1 and 7.2% per year. Reference Maron, Rowin and Casey172,Reference Miron, Lafreniere-Roula and Steve Fan174 While ICDs have improved the outcomes for patients with HCM resuscitated from SCA, the accurate identification of risk factors for SCD to guide primary prevention ICD implantation remains a challenge, particularly given the potential progression of the disease process over time. Reference Maron, Rowin and Casey172–Reference Norrish, Cantarutti and Pissaridou175 A multicenter pediatric HCM registry study reported the 5-year risk of SCA was 9%. Reference Miron, Lafreniere-Roula and Steve Fan174 Primary and secondary prevention ICDs were implanted in 18 and 4% of the cohort, respectively. Only 2.5% of the patients with a primary prevention ICD received an appropriate discharge at 5 years’ follow-up, highlighting the major gaps in knowledge for accurate prediction of SCD risk in pediatric HCM patients. Reference Miron, Lafreniere-Roula and Steve Fan174
Previously published clinical practice guidelines define high risk for SCD in HCM by the presence of ≥1 clinical risk factors based on primarily adult data. Reference Epstein, Dimarco and Ellenbogen6,Reference Tracy, Epstein and Darbar7,Reference Priori, Wilde and Horie14 Recent studies, however, suggest that the significance of the various risk factors may differ in children compared to adults. Reference Miron, Lafreniere-Roula and Steve Fan174–Reference Norrish, Ding and Field177 Conventional risk factors include survival from an SCA, spontaneous sustained VT, unexplained syncope, nonsustained VT, family history of early HCM-related SCD, and massive left ventricular hypertrophy. Reference Priori, Wilde and Horie14,Reference Maron, Spirito and Ackerman173 While a left ventricular wall thickness ≥30 mm is considered a risk factor in adults, left ventricular hypertrophy is determined relative to age and body size and therefore should be converted to a z score when evaluating this as a risk factor in smaller children. Reference Miron, Lafreniere-Roula and Steve Fan174,Reference Norrish, Ding and Field177 A multicenter pediatric study showed that a left ventricular posterior wall thickness z score ≥5 was associated with VT/VF or SCA, while a meta-analysis of pediatric studies reported a maximum left ventricular wall thickness ≥30 mm or a z score ≥6 associated with an increased risk of SCD. Reference Norrish, Cantarutti and Pissaridou175,Reference Balaji, DiLorenzo and Fish176
Other secondary risk factors for SCD, such as late gadolinium enhancement (LGE) on cardiac magnetic resonance imaging (MRI), have been investigated, but the predictive value of LGE for SCD in children is still unclear. Reference Briasoulis, Mallikethi-Reddy and Palla179,Reference Prinz, Schwarz and Ilic180 The evolving role of genetic testing for specific “malignant” sarcomere mutations remains debated and requires further investigation before inclusion as specific risk factors for SCD in pediatric patients with HCM. Reference Vermeer, Clur and Blom181,Reference Maron, Maron and Semsarian183
Restrictive cardiomyopathy
There are limited data regarding the use of ICDs in patients with restrictive cardiomyopathy. Reference Muchtar, Blauwet and Gertz184,Reference Walsh, Grenier and Jeffries185 The underlying cause of the restrictive cardiomyopathy is most commonly due to abnormalities in the sarcomeric genes, resulting in overlap with the HCM phenotype as well as risk for both tachyarrhythmias and conduction block. Reference Walsh, Grenier and Jeffries185 Given the overlap with HCM, ICD recommendations for patients with restrictive cardiomyopathy are included under the HCM and general guidelines. However, these patients do require unique consideration as, in comparison to those with HCM, patients with purely restrictive cardiomyopathy may not display the typical risk factors such as thickening of the intraventricular septum but do appear to be at higher risk for SCD, SCA, and cardiac transplant. Reference Webber, Lipshultz and Sleeper186,Reference Wittekind, Ryan and Gao187 Given this, ICD implantation may be appropriate in patients with a restrictive cardiomyopathy who present with heart failure or unexplained syncope when transplant is not an immediate option. Reference Zangwill, Naftel and L’Ecuyer188
Arrhythmogenic cardiomyopathies

Recommendation-specific supportive text
Arrhythmogenic cardiomyopathy (ACM) encompasses a spectrum of disorders of the myocardium with the distinguishing feature of presentation with sustained arrhythmias. Reference Towbin, McKenna and Abrams16 It includes, but is not limited to, genetic disorders such as arrhythmogenic right/left ventricular cardiomyopathy, lamin A/C, filamin-C, phospholamban, and cardiac amyloidosis. Reference Towbin, McKenna and Abrams16 Under this definition, infectious processes such as myocarditis and Chagas disease and inflammatory disorders such as sarcoidosis may also be classified. Most of these entities are infrequent before puberty and often overlap with other cardiomyopathies in presentation, particularly dilated cardiomyopathy. Reference Towbin, McKenna and Abrams16
The diagnosis of ACM requires a high degree of suspicion. The initial evaluation should include clinical history, physical examination, detailed family history, 12-lead ECG, echocardiography, ambulatory electrocardiography monitoring, exercise testing, and cardiac MRI. Additional testing includes signal-averaged ECG and genetic testing. Reference Towbin, McKenna and Abrams16,Reference DeWitt, Chandler and Hylind189
The most frequent form of ACM in the pediatric age-group is arrhythmogenic right ventricular cardiomyopathy (ARVC). Reference DeWitt, Chandler and Hylind189 ARVC is characterized by predominant right ventricular involvement with fibro-fatty replacement of the myocardium resulting in conduction abnormalities and ventricular arrhythmias. Biventricular disease is associated with younger age of onset. Reference Mazzanti, Ng and Faragli190,Reference Te Riele, James and Sawant191 ARVC is either de novo or inherited in an autosomal dominant pattern involving variances in desmosomal genes or desmosome-associated proteins. Reference Towbin, McKenna and Abrams16,Reference Ortiz-Genga, Cuenca and Dal Ferro193 Syncope is reported in 16–40% of ARVC patients at the time of diagnosis, is frequently exercise related, and has been associated with high arrhythmic risk. Reference Towbin, McKenna and Abrams16,Reference Te Riele, James and Sawant191 In adult ARVC cohorts, risk factors for SCD include syncope presumed due to ventricular arrhythmia, sustained or nonsustained VT, and severe right ventricular and/or left ventricular systolic dysfunction. Reference Al-Khatib, Stevenson and Ackerman12,Reference Towbin, McKenna and Abrams16 Due to the relatively low prevalence of manifest ARVC in the young, there is a paucity of data regarding risk stratification for SCD in pediatric patients with ARVC.
Overall, SCD affects 2–15% of young patients with ACM. Reference DeWitt, Chandler and Hylind189,Reference Te Riele, James and Sawant191 Patients presenting with SCD and/or sustained ventricular arrhythmias have a class I indication for an ICD implantation. Reference Al-Khatib, Stevenson and Ackerman12,Reference Towbin, McKenna and Abrams16 The limited available data on risk stratification in the young hamper the indication for a primary prevention ICD in this population. However, ICD implantation is reasonable in patients with ACM with hemodynamically tolerated sustained VT, syncope presumed due to ventricular arrhythmia, or an LVEF ≤ 35%. Candidacy and timing of cardiac transplantation and whether a wearable external defibrillator is a reasonable alternative should be taken into consideration on an individual basis for those patients with advanced heart failure. Reference Kusumoto, Calkins and Boehmer5
Nonischemic dilated cardiomyopathy

Recommendation-specific supportive text
The incidence of SCD in pediatric patients with idiopathic/NIDCM is only 1–5%, which is significantly less than that in adult patients. Reference Bharucha, Lee and Daubeney195,Reference Pahl, Sleeper and Canter196 Although studies have shown some ICD survival benefit for secondary prevention in pediatric dilated cardiomyopathy, the low incidence of SCD has made it quite difficult to establish risk factors to guide recommendations for primary prevention ICD implantation. Reference Bharucha, Lee and Daubeney195 In contrast to some studies of adult patients with NIDCM and LVEF ≤ 35%, Reference Bardy, Lee and Mark198 there is no clear evidence that ICDs implanted for primary prevention improve survival for pediatric patients with NICDM. Reference El-Assaad, Al-Kindi and Oliveira199,Reference Rhee, Canter and Basile200 However, primary prevention ICDs may be considered for patients with syncope or severe impairment of left ventricular function despite optimal medical therapy (beta-blockers and afterload reduction) and after careful consideration of device-related complication risks, candidacy and timing of cardiac transplantation, and whether a wearable external defibrillator is a reasonable alternative. Reference Kusumoto, Calkins and Boehmer5,Reference Kirk, Dipchand and Rosenthal17,Reference Dubin, Berul and Bevilacqua194,Reference Middlekauff, Stevenson, Stevenson and Saxon197
The phenotype of NIDCM may overlap with other types of pediatric cardiomyopathies resulting in variable risks of SCD. For example, the Sudden Death in Childhood Cardiomyopathy study showed that the risk of SCD varied according to cardiomyopathy phenotype. Reference Bharucha, Lee and Daubeney195 The cumulative incidence of SCD at 15 years was 5% for idiopathic dilated cardiomyopathy compared to 23% for left ventricular noncompaction. Myocardial dysfunction and/or a history of clinically significant arrhythmias are strongly associated with mortality in left ventricular noncompaction. Reference Jefferies, Wilkinson and Sleeper201,Reference Brescia202 Therefore, factors that may influence the decision regarding implantation of a primary prevention ICD include the underlying etiology of the NIDCM, the cardiomyopathy phenotype, the degree of ventricular dysfunction, and the presence of cardiac arrhythmias. Reference van Waning, Caliskan and Hoedemaekers203
ICD indications for congenital heart disease

Recommendation-specific supportive text
The association between CHD and arrhythmias has been well established. First demonstrated in repaired Tetralogy of Fallot, multiple studies since have identified risk factors for VT or SCD including residual cardiac defects, alterations in hemodynamics, and scars from prior interventions/surgeries. Reference Khairy, Harris and Landzberg204–Reference Nieminen, Jokinen and Sairanen206 Correction of residual abnormalities or ablation of arrhythmogenic substrate may improve ventricular function and reduce symptoms. However, this may be inadequate to prevent the risk of subsequent VT or SCA in all but a select group of patients. Reference Miyazaki, Sakaguchi and Ohuchi207,Reference Zeppenfeld, Schalij and Bartelings208 ICD placement may therefore be appropriate in patients with, or at high risk of, potentially life-threatening arrhythmias. Reference Khairy, Van Hare and Balaji9,Reference Al-Khatib, Stevenson and Ackerman12,Reference Priori, Blomström-Lundqvist and Mazzanti13
While ICDs are commonly placed for both primary and secondary prevention in patients with CHD, those with CHD appear to have an increased risk of inappropriate shocks compared to those with ICDs and without CHD. Reference Berul, Van Hare and Kertesz130,Reference Von Bergen, Atkins and Dick131,Reference Jordan, Freedenberg and Wang211–Reference Krause, Müller and Wilberg213 Appropriate ICD shock rates of 3–6% per year have been shown with an increased frequency of appropriate shocks for secondary prevention indications. Reference Khairy, Harris and Landzberg204 Antitachycardia pacing has been shown to be effective in VT termination and reducing ICD shocks. Reference Kalra, Radbill and Johns214 Patients with CHD receiving an ICD have an increased rate of complications as high as 26–45%, as well a high rate of inappropriate shocks. Reference Berul, Van Hare and Kertesz130,Reference Von Bergen, Atkins and Dick131,Reference Dechert, Bradley and Serwer212,Reference Krause, Müller and Wilberg213 The role of programmed stimulation and presence and degree of ventricular dysfunction as risk factors for SCD in CHD and thus primary prevention ICDs continues to be debated. Reference Sandhu, Ruckdeschel and Sauer215–Reference Khairy, Landzberg and Gatzoulis217 ICD implantation can be especially challenging in patients with CHD due to anatomic complexity, intracardiac shunts, or limited vascular access. This may require nonstandard approaches such as epicardial leads, nontransvenous defibrillation coils or a subcutaneous ICD. Reference Radbill, Triedman and Berul218,Reference von Alvensleben Johannes, Dechert and Bradley David219
Insertable cardiac monitors
Syncope and palpitations are common symptoms in children and adolescents. ICMs (also referred to as implantable loop recorders) are subcutaneously implanted devices that provide long-term rhythm surveillance and documentation during a patient’s symptomatic event. Rhythm tracings during events are either patient-triggered recordings or stored automatically by predefined criteria. Long-term ECG monitoring using an ICM is recommended in symptomatic cases when the personal history, physical examination, and noninvasive investigations have been inconclusive, especially due to the low frequency of clinical events and/or limited feasibility of a complete diagnostic protocol. Reference Steinberg, Varma and Cygankiewicz220–Reference Babikar, Hynes and Ward224 A remote monitoring program with immediate wireless data transfer capability and daily diagnostic data availability has overcome the prior problem of limited device storage capacity and has facilitated early diagnosis. ICMs, along with Holter monitoring, external loop recorders, and remote at-home telemetry, are reported to provide a diagnostic yield of 43–50% at 2 years and 80% at 4 years. Reference Moya, Sutton and Ammirati221–Reference Placidi, Drago and Milioni226
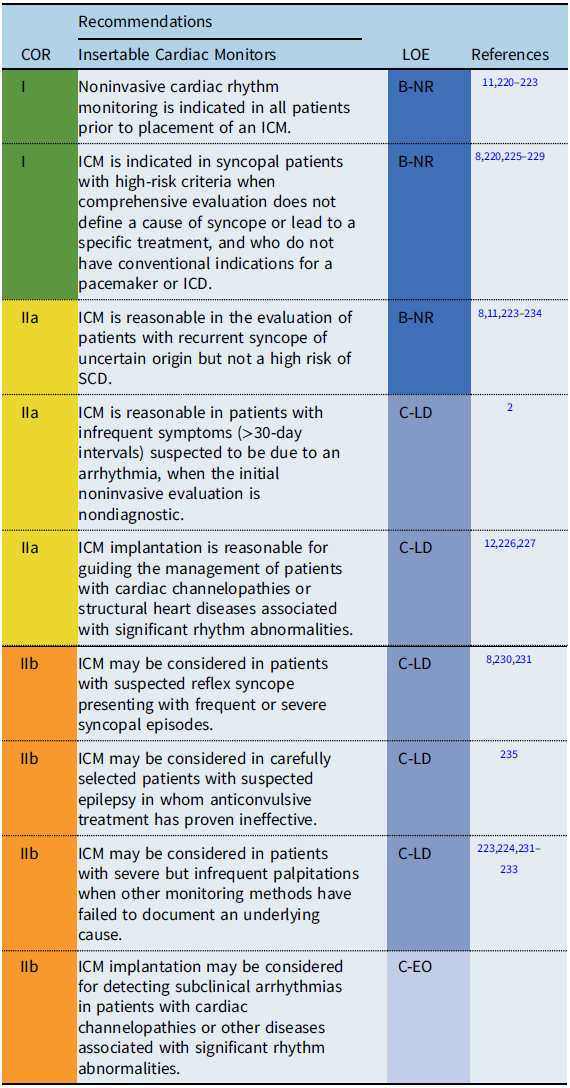
Recommendation-specific supportive text
Several observational studies have demonstrated a benefit of ICM in establishing a diagnosis for recurrent symptoms of unclear etiology when other monitoring methods have failed to document an underlying cause.
-
Syncope: Cardiac or undefined syncope may be present in up to 8% of syncopal events in children and adolescents. Reference Hernández-Madrid, Paul and Abrams8 In adults, monitoring with an ICM has been shown to be more cost-effective for establishing a diagnosis than other methods of rhythm monitoring and should be the method of choice when arrhythmogenic syncope is suspected but not proven. Reference Pradhan, Robinson and Shivapour223,Reference Placidi, Drago and Milioni226
-
Palpitations: ICM implantation should be considered on an individual basis, taking into account each patient’s underlying cardiac condition, the severity of symptoms, and age- and development-related monitoring limitations. Reference Frangini, Cecchin and Jordao233,Reference Kenny, Chakrabarti and Ranasinghe234
-
Bradyarrhythmias: ICM may be useful in the monitoring of bradyarrhythmias and their correlation with clinical symptoms. ICM may also be useful for patients at risk for intermittent or progressive AV block including patients with neuromuscular diseases, progressive cardiac conduction diseases, and Kearns-Sayre syndrome. Reference Ha, Tarnopolsky and Bergstra91,Reference Di Mambro, Tamborrino and Silvetti98
-
Other conditions: ICM may be useful for occult arrhythmia detection in asymptomatic children with potentially lethal cardiac diseases (e.g., inherited primary arrhythmia syndromes, cardiomyopathies) and may identify events that warrant changes in patient management. Reference Placidi, Drago and Milioni226,Reference Rossano, Bloemers and Sreeram230–Reference Al Dhahri, Potts and Chiu232 Furthermore, monitoring with an ICM may provide psychological reassurance for parents of children at risk for malignant arrhythmias. Reference Frangini, Cecchin and Jordao233
CIED lead management
Lead management remains a vitally important issue in children, both with and without CHD. Updated consensus statements regarding lead management and extraction were put forth in 2017 Reference Kusumoto, Schoenfeld and Wilkoff18 and 2018. Reference Bongiorni, Burri and Deharo236 The following recommendations are complementary to these existing guidelines with a nuanced perspective focusing on pediatrics and patients with CHD.
The definitions used related to lead management in this document are similar to those explained in the 2017 statement. Reference Kusumoto, Schoenfeld and Wilkoff18 The general category of “lead removal” includes “lead explant” that is performed using a simple traction technique and “lead extraction” that refers to removal of a lead that has been implanted for >1 year or requiring the assistance of specialized equipment regardless of implant duration. Reference Kusumoto, Schoenfeld and Wilkoff18 The most common indications for transvenous lead extraction in children remain lead failure (76%) and venous occlusion. Reference Fu, Huang and Zhong237–Reference McCanta, Kong and Carboni243 Pediatric patients are more likely to outlive the functionality of their leads, amplifying the importance of lead durability, longevity of venous access, and long-term risks of lead dysfunction. Coupled with studies in children indicating that older lead age is an independent predictor of need for advanced extraction techniques and added complexity, greater emphasis should be given to the potential risks of lead abandonment in this population. Reference Cecchin, Atallah and Walsh240,Reference Mah, Prakash and Porras241
Available extraction tools in children are similar to those in the adult population, as there are no special tools designed specifically for children or patients with CHD. These include locking stylets, telescoping sheaths, femoral snares, and mechanical, laser, or radiofrequency-powered sheaths. Reference McCanta, Kong and Carboni243,Reference Moak, Freedenberg and Ramwell244 Extractors should be appropriately trained, and the entire team must have working knowledge of these tools and techniques. Additionally, expertise in pediatrics, CHD, and surgically corrected anatomy is mandatory, as the methods and potential complications may be specific to both size and anatomy. Unusual lead position and foreign material such as prosthetic valves, conduits, and baffles may necessitate adjustments in approach. Reference Fender, Killu and Cannon242 Younger patients are also more likely to require the use of femoral extraction tools. Reference El-Chami, Sayegh and Patel245 Lastly, the presence of epicardial leads may require surgical access as a component of the procedure. Reference Mah, Prakash and Porras241
The environment for lead extractions in the pediatric population warrants careful patient-centered assessment for optimal preparedness (Table S1 in Supplemental Appendix 3). As in adults, major complications are relatively rare, but significant potential for life-threatening events exists. Reference Bongiorni, Burri and Deharo236,Reference Riley, Petersen, Ferguson and Bashir238,Reference Atallah, Erickson and Cecchin239 The contribution of complex CHD to the likelihood of successful extraction has varied, ranging from 74 to 94% for complete removal. The rates of major complications, however, have been found to be consistent between 3 and 4%. Reference Atallah, Erickson and Cecchin239,Reference Cecchin, Atallah and Walsh240,Reference Fender, Killu and Cannon242 Specific complications may be more prevalent based on anatomy and size, such as increased subpulmonary AV valve regurgitation in transposition of the great arteries, or increased risk of tricuspid or pulmonary valve involvement related to excess lead slack left for growth in smaller children. Reference McCanta, Kong and Carboni243–Reference Webster, Margossian, Alexander, Cecchin, Triedman, Walsh and Berul248 Additionally, although patient age and size have not been shown to predict venous occlusion, more vigorous fibrous adhesions have been implicated in younger patients. Reference Bar-Cohen, Berul and Alexander249
Due to the complexities and potential for serious events in this population, lead extractions should only be performed in centers with an institutional commitment to the development and maintenance of a collaborative team. This includes a need for appropriate facilities, necessary equipment, trained personnel, and the ability to manage all complications. A multidisciplinary team familiar with nuances related to CHD is vital to maximizing procedural safety and efficacy (Table S1 in Supplemental Appendix 3). In particular, it is essential that the cardiac surgeon and surgical team be readily available to immediately provide open-chest surgical repair. Based on congenital anatomy and previous surgeries, emergent surgical approach via thoracotomy (versus sternotomy) may be preferred in certain scenarios, and focused pre-procedure imaging and planning is critical.
It must be recognized that several gaps in knowledge persist in relationship to lead management in children and patients with CHD. Reference Joy, Kumar and Poole250 This includes limited data in the very young, as well as the impact of multiple extractions over a lifetime on vascular integrity and valvular function. There also continues to be lack of clarity regarding prophylactic lead extractions at the time of generator change, Reference Sohal, Williams and Akhtar251 and long-term prospective studies on abandonment versus extraction in the young do not exist.

*Based on adult lead management guidelines. Reference Kusumoto, Schoenfeld and Wilkoff18,Reference Bongiorni, Burri and Deharo236
Recommendation-specific supportive text
The most common indications for lead removal are infection, venous occlusion, advisory or recall as a result of potential lead malfunction, or mechanical lead failure. Reference Kusumoto, Schoenfeld and Wilkoff18,Reference Joy, Kumar and Poole250–Reference Escudero, Mah and Miyake256 Lead management involves the assessment of risks and benefits of whether or not to remove the lead based on the individual clinical condition of the patient as well as lead characteristics. Reference Kusumoto, Schoenfeld and Wilkoff18,Reference Bongiorni, Burri and Deharo236,Reference Viganego, O’Donoghue and Eldadah253
Upper extremity venous thrombosis and venous stenosis are not absolute indications for lead removal. However, instances in which a thrombosis causes significant symptoms (e.g., superior vena cava syndrome, ongoing thromboembolic events), or in which stenosis/occlusion impedes upgrade of an existing device, are generally considered appropriate circumstances to remove an existing lead. Reference Bongiorni, Burri and Deharo236–Reference Riley, Petersen, Ferguson and Bashir238
Infections, which can result in CIED device and lead removal, can generally be grouped into major categories: isolated pocket infection, CIED-associated endocarditis, bacteremia without an alternative source (particularly Staphylococcus aureus), or bacteremia that persists or recurs despite appropriate antimicrobial therapy. Reference Kusumoto, Schoenfeld and Wilkoff18,Reference Bongiorni, Burri and Deharo236 These situations are associated with challenging management decisions and often require CIED device and lead removal when the infection is more than superficial cellulitis. Reference Viganego, O’Donoghue and Eldadah253,Reference Baddour, Epstein and Erickson254
Advisory/recall: The decision to remove an apparently normally functioning lead or leads in response to a manufacturer’s or regulatory body’s recall or warning is complex and should be performed in close consultation with an electrophysiologist with consideration for the patient’s overall clinical status. Reference Janson, Patel and Bonney255,Reference Escudero, Mah and Miyake256
Recommendations for CIED follow-up and ancillary testing

Recommendation-specific supportive text
Cardiovascular implantable electronic devices (CIEDs) that are currently amenable to remote interrogation and monitoring (RIM) include pacemakers, ICDs, and ICMs. The benefits of routine RIM are extensively validated and maximize the opportunity for prolongation of battery life as well as early detection and intervention of CIED malfunctions, arrhythmic issues, and adverse events. Reference Dechert, Sewer and Bradley268–Reference Hummel, Leipold and Amorosi272 Remote evaluation of CIEDs began with transtelephonic monitoring (TTM), an analog-based technology that delivered limited data on pacemaker function via transmission over a telephone landline. RIM technologies, which are now incorporated in all CIEDs, are recommended over TTM because of the additional diagnostic data they provide, but TTM is still in use with older devices that do not have RIM capability. At present, there are no established guidelines for CIED follow-up in the pediatric population with resultant variability in monitoring of pediatric CIEDs. Reference Dechert, Sewer and Bradley273,Reference Boyer, Silka and Bar-Cohen274
Several device, lead, and pocket complications can be seen within the first few days to weeks after CIED implantation, and an in-person evaluation (IPE) is useful in the early post-implant phase. Although specific patient care guidelines for IPE and RIM for children have not been established, the Centers for Medicare & Medicaid Services has established reimbursement guidelines for IPE and RIM for patients with pacemakers.
In addition to monitoring the CIED itself, it is equally important to evaluate the impact of CIED-related consequences on the patient with ancillary testing. Ancillary testing may consist of but is not limited to 12-lead ECG, echocardiogram, ambulatory rhythm monitoring, chest X-ray, and exercise stress testing. The annual IPE should include evaluation of the patient’s underlying rhythm. In patients who have >40% paced ventricular rhythm, it is reasonable to assess systemic ventricular function by echocardiogram every 1–3 years for early recognition of pacemaker-induced cardiomyopathy or lead-related valve regurgitation. Reference Dasgupta, Madani and Figueroa259–Reference Tantengco, Thomas and Karpawich263 Ambulatory rhythm monitoring and/or exercise stress testing may be useful in patients with arrhythmia concerns or symptoms related to activity and to assist with device optimization. Reference Gonzalez Corcia, Remy and Marchandise264–Reference Diemberger, Gardini and Martignani267,Reference Kadish, Buxton and Kennedy275–Reference Sampio, Craveiro and Darrieux277 It is reasonable to consider lead surveillance with chest X-ray in the acute post-implant period and to consider repeating every 1–3 years according to growth. Reference Mah, Prakash and Porras241,Reference Berul, Villafane and Atkins247
Special considerations
CIEDs and magnetic resonance imaging

Recommendation-specific supportive text
The 2017 MRI and Radiation Exposure in Patients with CIEDs Consensus Statement provides comprehensive recommendations for individuals with both conditional and nonconditional transvenous devices. Reference Indik, Gimbel and Abe19 With MRI, there is potential risk for heating of the lead, increase in pacing thresholds, sudden battery depletion, and inappropriate sensing/pacing. The consensus statement also provides guidance for CIED programming and evaluation pre-, during, and post-MRI along with a protocol of testing and patient-specific considerations. However, these recommendations are not specific for patients with abandoned or epicardial CIED leads and make no specific recommendations for MRI in these cases. Reference Nazarian, Hansford and Rahsepar283,Reference Padmanabhan, Kella and Mehta284
Regarding epicardial lead considerations, younger patients and those with CHD have a greater likelihood of requiring epicardial leads. Additionally, as there are no MRI conditional epicardial leads, even when used with a conditional device, the system is considered nonconditional. The 2017 recommendations suggest a possible contraindication to MRI, and in the pediatric section no recommendations regarding epicardial leads are made. However, when attached to a device, the limited data show only a small increase in risk for substantial alterations of the pacing threshold or changes in sensing after MRI. Reference Bireley, Kovach and Morton279–Reference Gakenheimer-Smith, Etheridge and Niu281,Reference Rahsepar, Zimmerman and Hansford285,Reference Balmer, Gass and Dave286
Regarding abandoned leads, in vitro data suggest that epicardial leads are more likely to generate heat than transvenous leads; however, small studies evaluating MRIs in patients with both epicardial and transvenous abandoned leads suggest that it can be done safely in the majority of cases. Reference Schaller, Brunker and Riley282,Reference Nazarian, Hansford and Rahsepar283,Reference Langman, Goldberg and Finn287–Reference Higgins, Gard and Sheldon289 Even so, these studies do not imply lack of an effect on the myocardium underlying the abandoned lead. In summary, the data on MRI use in epicardial or abandoned leads are inadequate to provide specific recommendations or an absolute contraindication.
Acknowledging the sparsity of data, but also appreciating the importance of MRI for diagnosis, prognosis, and surgical planning, individualized consideration of the risk/benefit ratio of MRI in young patients must be made on a “case-by-case basis.” Reference Indik, Gimbel and Abe19
CIEDs and sports participation
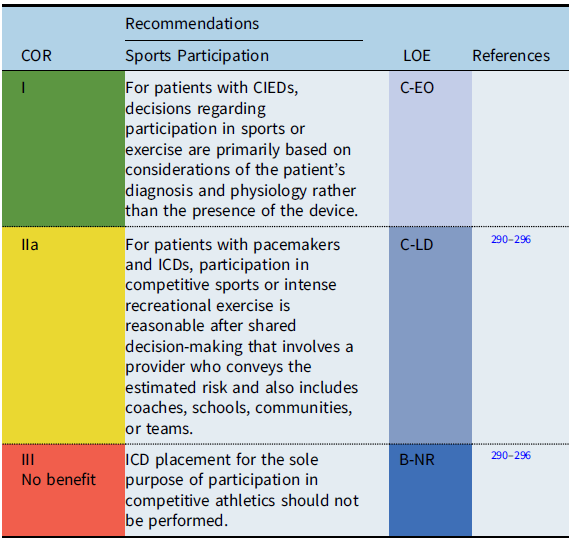
Recommendation-specific supportive text
The safety of sports participation for patients with CIEDs remained fundamentally unstudied until the past decade. Despite a dearth of research, initial published guidelines recommended against strenuous competitive sports participation (greater than class Ia) for patients with pacemakers or ICDs. Reference Zipes, Link and Ackerman295–Reference Mitchell, Haskell and Snell298 Subsequent to publication of guidelines in 2005, evidence emerged suggesting that risks of sports participation for athletes with CIEDs may be lower than hypothesized. Reference Saarel, Pilcher and Etheridge290–Reference Saarel, Law and Berul293
Surveys from HRS (2006) and PACES (2013) suggested that many patients with pacemakers and ICDs had participated in sports without adverse events. Reference Saarel, Pilcher and Etheridge290,Reference Mitchell, Haskell and Snell298 Thus, an international ICD Sports Registry was initiated and reported in 2013–2018. Reference Lampert, Olshansky and Heidbuchel292,Reference Saarel, Law and Berul293 The registry consisted of 129 patients <21 years of age including varsity high school and college athletes. While shocks occurred during sports, there were no deaths, no resuscitated arrests, and no arrhythmia-related injuries during sports. In addition, the rate of lead malfunction was similar to previously reported rates in unselected populations. Reference Lampert, Olshansky and Heidbuchel292 The conclusion was made that despite the potential for exercise to be arrhythmogenic, some young patients with ICDs can participate in sports without injury or failure to terminate the arrhythmia.
When questions arise about sports participation in youth with CIEDs, it is now standard practice to counsel patients and families about the risks, including potential for increased rate of ventricular tachyarrhythmias and damage to the pacemaker or ICD system. Counseling is patient specific; the underlying cardiac disease, type of device, indication for implant, position of leads and pulse generators, underlying heart rhythm, patient age, and type of athletic activity are considered when estimating risk. Reference Mitchell, Haskell and Snell298,Reference Lampert, Cannom and Olshansky299 Shared decision-making processes that include the patient, family, coach, school, team, and other community members should be utilized to determine the best course of pursuit for individuals with CIEDs and sporting endeavors.
CIEDs in low- and middle-income countries
A quote often used by doctors dealing with cardiac rhythm problems in resource limited settings (or indeed any branch of medicine) is the Italian proverb “Il meglio è l’inimico del bene,” which translates to “better is the enemy of good.” Low- and middle-income countries (LMIC) are defined as those designated by the World Bank based on per capita income. 300 They represent a heterogenous community including countries where the primary deterrent to the use of implantable devices is the cost of the device (India and most countries in Asia and Southern Africa) and those in which the deterrent is both the cost and the availability (sub-Saharan Africa). Reference Bonny, Mgantcha and Jeilan301 These problems have been alleviated to a small extent by philanthropic measures initiated by the Western world as well as universal health care policies announced by various governments in recent years. Pediatric cardiologists in these countries circumvent these problems by using two primary strategies:
-
1. Patient-specific strategy. Most centers in LMIC tailor the indications of the device to an individual patient instead of following standard guidelines. This is based on available evidence and is not anecdotal, as is widely believed. In postoperative heart block, it has been shown that 95% of AV conduction recovery happens by the 10th postoperative day. Reference Weindling, Saul and Gamble50,Reference Romer, Tabbutt and Etheridge51 Children with intermittent AV conduction on telemetry as well as an accelerated junctional rhythm have been shown to have a much higher recovery rate. Reference Murray, Smith and Flack302,Reference Paech, Dahnert and Kostelka303 Hence, many centers prefer to wait till the 10th postoperative day before placement of a permanent pacemaker. In children (and young adults) who have intermittent AV conduction and those with a reasonably fast narrow complex escape rhythm, centers may choose to wait even longer for recovery of AV conduction so as to avoid the use of a permanent pacemaker. Late recovery of surgically induced AV block has also been reported. Reference Paech, Dahnert and Kostelka303,Reference Batra, Wells and Hinoki304 Occasionally patients have been discharged home before return of AV conduction, and spontaneous recovery was documented on follow-up. Reference Bruckheimer, Berul and Kopf305 In patients with corrected CHD and normal ventricular function, a single-chamber pacemaker is used in most centers, while a dual-chamber pacemaker is reserved for children with palliated hearts and more than mild ventricular systolic dysfunction.
-
2. The use of explanted devices. Devices explanted from deceased patients with a battery life of >50% of a new device have been used in patients from a resource-limited setting. Reference Khairy, Lupien and Nava306 A hypothetical increased risk of infection from an explanted device has been a major deterrent for this approach. However, a recent meta-analysis of 18 studies involving 2270 patients in whom a reused pacemaker was placed revealed no significant increase in the risk of surgical site infection compared to a new device and a small increase in the risk of device malfunction. Reference Baman, Meier and Romero307 Even this small risk was shown to be predominantly technical and did not endanger the life of the patient. Standard guidelines on device reuse in India have been published. Reference Kapoor, Vora, Nataraj and Mishra308
While most centers have used such inventive strategies to implant a device in children, follow-up interrogation of the device is often challenging. Most pediatric cardiac centers in LMIC are located in a few urban centers with a very large referral area. Frequent travel for device interrogation is often impossible for families because of the costs involved as well as the loss of livelihood. There is no published literature on the gravity of this problem, as most centers lack the resources to follow patients meticulously. Although remote monitoring is ideally suited for these patients, the added cost of the device makes it less attractive. The recent launch of mobile-based remote monitoring pacemakers using Bluetooth technology has immense potential in LMIC if such devices can be priced affordably. Reference Roberts and El Refai309
Shared decision-making

Recommendation-specific supportive text
Shared decision-making is a process whereby patients, families, and providers exchange information and dialogue about medical diagnostic and treatment options. Reference Elwyn, Frosch and Thomson310 The goal is for patients and their families to reach evidence-informed and value-congruent medical decisions collaboratively with their clinicians. This modern model for health care decision-making has superseded paternalism, a previous model whereby providers made medical decisions on behalf of their patients using the ethical principal of beneficence. A shared decision-making approach, combining the ethical principles of professional beneficence and patient autonomy, has been shown to improve patient outcomes. Reference Greenfield and Kaplan311,Reference Legare, Adekpedjou and Stacey312
The use of shared decision-making should occur prior to all CIED implantation procedures. Clinicians must estimate and clearly describe the potential benefits and risks for the patient and their family. Some decisions will be relatively straightforward; for example, the decision to implant a permanent pacemaker to treat postoperative surgical complete heart block in a patient who is pacemaker dependent will be largely uncontestable. However, other treatment decisions, such as implantation of an ICD for primary prevention of SCD, are more complex and nuanced and include choice of ICD system, device location, and personalized estimation of risk of life-threatening arrhythmia for the particular patient over time.
Finally, the shared decision-making process is also important and applicable to post-implant diagnostic and treatment decisions for our patients with CIEDs including genetic testing, MRI, sports participation, pregnancy, cardiac surgery, and device reprogramming, removal, or revision.
Knowledge gaps and future research
There have been no RCTs involving CIEDs in children. Therefore, the recommendations put forth in this guideline are based on data from observational studies in children, clinical trials in adults, and expert opinion. Clinical trials, especially RCTs, remain challenging in pediatric populations because of low overall event rates in specific diseases and variations in disease progression from birth to adulthood. Reference Khairy, Dore and Poirier313
Critical knowledge gaps exist is several areas. Reference Goette, Auricchio and Boriani314 One example is the use of ICDs for the primary prevention of SCD. With reduction in size and the development of novel lead configurations, ICD use in pediatrics has increased dramatically while the age at implant has decreased significantly. Reference Berul, Van Hare and Kertesz130,Reference Burns, Evans and Kaltman315 However, the accurate identification of patients at increased risk remains perplexing.
Several other important knowledge gaps include but are not limited to the optimal timing of pacemaker implantation after postoperative AV block, contemporary outcomes of patients with isolated CCAVB who do not undergo pacing, risk factors for pacemaker-induced cardiomyopathy, optimal age and body size for transvenous lead implantation, and safety of MRI with abandoned or epicardial leads.
With continuing technological innovations, future research is needed to develop pediatric-specific criteria for application of these new technologies. These include subcutaneous ICDs, leadless pacemakers, and conduction system pacing. Reference von Alvensleben Johannes, Dechert and Bradley David219,Reference Breatnach, Dunne and Al-Alawi316,Reference Lyon, Dandamudi and Kean317 Multicenter prospective registries as well as high-quality retrospective data are necessary to provide real-world evidence for new and existing CIED technologies. Future research should be conducted in collaboration with PACES, other relevant scientific societies, the U.S. Food and Drug Administration, and industry partners for development of pediatric “appropriate” CIEDs and device algorithms to specifically benefit young patients and improve their long-term outcomes.
Supplementary data
Supplementary data (Appendices 3 and 4) associated with this article can be found in the online version at https://doi.org/10.1017/S1047951121003413.
Appendix A1
Author relationships with industry
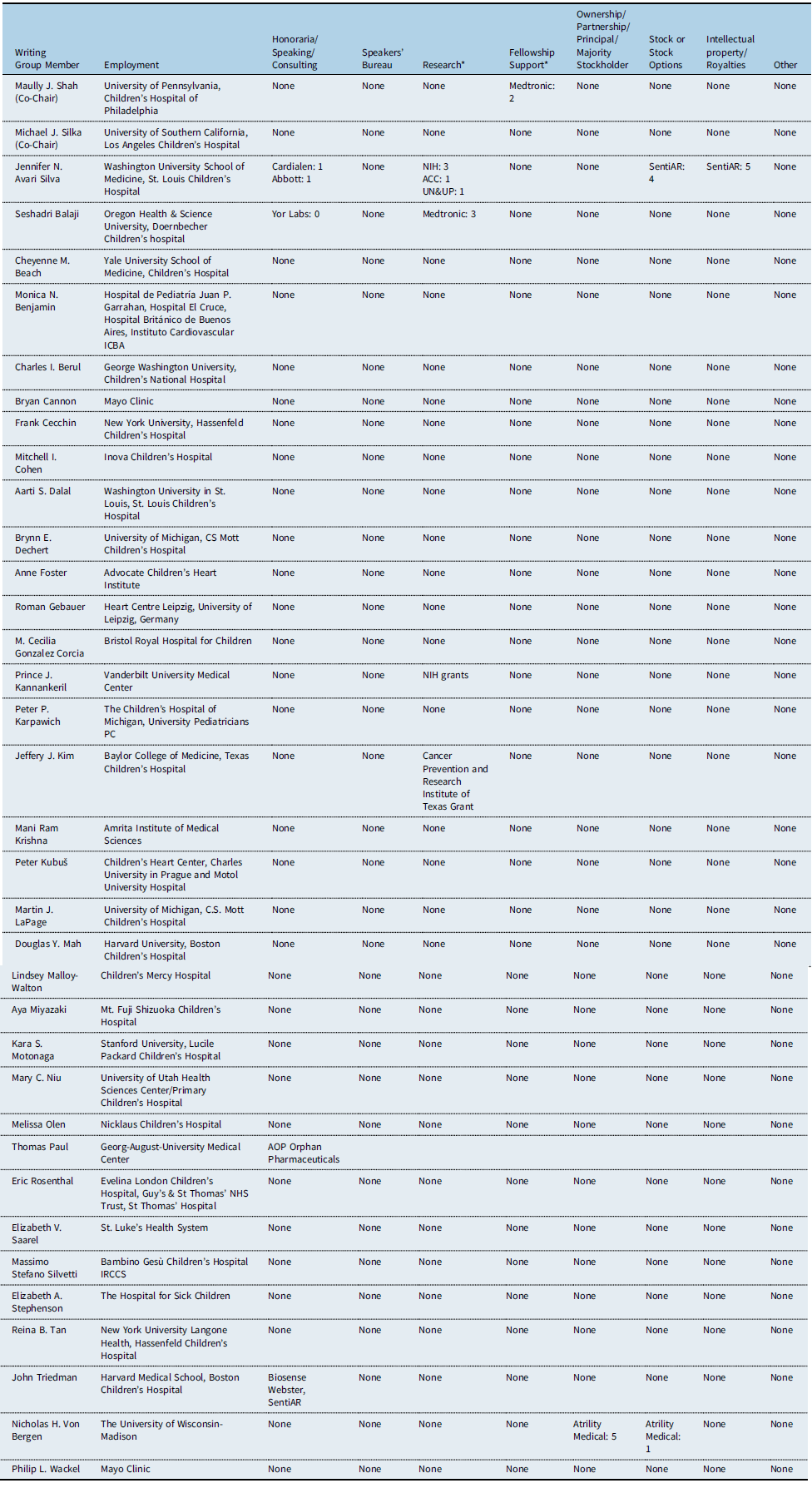
Number value: 0 = $0; 1 = ≤$10,000; 2 = >$10,000 to ≤$25,000; 3 = >$25,000 to ≤$50,000; 4 = >$50,000 to ≤$100,000; 5 = >$100,000.
*Research and fellowship support are classed as programmatic support. Sources of programmatic support are disclosed but are not regarded as a relevant relationship with industry for writing group members or reviewers.
Appendix A2
Reviewer relationships with industry

Number value: 0 = $0; 1 = ≤$10,000; 2 = >$10,000 to ≤$25,000; 3 = >$25,000 to ≤$50,000; 4 = >$50,000 to ≤$100,000; 5 = >$100,000.
*Research and fellowship support are classed as programmatic support. Sources of programmatic support are disclosed but are not regarded as a relevant relationship with industry for writing group members or reviewers.