Introduction
Tephritidae is a speciose family of Diptera, with a great number of species characterized as serious agricultural pests (Bickel et al., Reference Bickel, Pape and Meier2009). In particular, the genera of Anastrepha, Bactrocera, Ceratitis, Dacus, Rhagoletis, and Zeugodacus include some of the most destructive fruit flies that cause severe economic losses due to crop damaging of commercial fruit and vegetable and restrictions to global trade (White and Elson-Harris, Reference White and Elson-Harris1992; De Meyer et al., Reference De Meyer, Delatte, Mwatawala, Quilici, Vayssieres and Virgilio2015b). From the genus Ceratitis, the Mediterranean fruit fly, Ceratitis capitata, is the best studied species used as a model pest organism, because of its almost global distribution and its enormous economic impact (Malacrida et al., Reference Malacrida, Gomulski, Bonizzoni, Bertin, Gasperi and Guglielmino2007). In the recent years, attention has been also drawn to the Ceratitis species of the African FARQ complex. Until 2016, the complex was known as FAR species complex and was considered to consist of three closely related species, C. fasciventris, C. anonae, and C. rosa (Virgilio et al., Reference Virgilio, Backeljau, Barr and De Meyer2008). However, accumulating evidence from studies on molecular genetics (Virgilio et al., Reference Virgilio, Delatte, Quilici, Backeljau and De Meyer2013), morphometrics (Van Cann et al., Reference Van Cann, Virgilio, Jordaens and De Meyer2015), developmental physiology (Tanga et al., Reference Tanga, Manrakhan, Daneel, Mohamed, Fathiya and Ekesi2015), behavior and sexual compatibility (De Meyer et al., Reference De Meyer, Delatte, Ekesi, Jordaens, Kalinová, Manrakhan, Mwatawala, Steck, Van Cann, Vaničhová, Břizová and Virgilio2015a), chemoecology (Vaníčková et al., Reference Vaníčková, Břízová, Mendonça, Pompeiano and Do Nascimento2015), and environmental preferences (Mwatawala et al., Reference Mwatawala, Virgilio, Joseph and De Meyer2015) lead to the conclusion that C. rosa was, in fact, consisting of two entities, one of which has been described as a new species within the complex, C. quilicii (De Meyer et al., Reference De Meyer, Mwatawala, Copeland and Virgilio2016). Microsatellite analysis indicated the existence of two genotypic groups in C. fasciventris, as well, referred to as types F1 and F2 (Virgilio et al., Reference Virgilio, Delatte, Quilici, Backeljau and De Meyer2013). This was confirmed also by morphological data, however, in the absence of integrative evidence, C. fasciventris is still considered as one species (De Meyer et al., Reference De Meyer, Delatte, Ekesi, Jordaens, Kalinová, Manrakhan, Mwatawala, Steck, Van Cann, Vaničhová, Břizová and Virgilio2015a).
The four members of the FARQ complex are highly polyphagous attacking plants from more than 25 different families and are considered a major threat to the agricultural production and economy of many countries of the African continent, as well as species of quarantine significance (White and Elson-Harris, Reference White and Elson-Harris1992; Smith et al., Reference Smith, McNamara, Scott and Holderness1997; De Meyer et al., Reference De Meyer, Copeland, Lux, Mansell, Wharton, White and Zenz2002). C. fasciventris and C. anonae are distributed mainly through Western and Central Africa, found sympatrically in several regions, while C. rosa and C. quilicii present overlapping distribution in Eastern and Southern Africa (De Meyer et al., Reference De Meyer, Copeland, Lux, Mansell, Wharton, White and Zenz2002, Reference De Meyer, Mwatawala, Copeland and Virgilio2016; Copeland et al., Reference Copeland, Wharton, Luke, De Meyer, Lux, Zenz, Machera and Okumu2006). It has been reported that C. rosa occupies mainly lower altitude areas, while C. quilicii predominates in cooler highland regions (Mwatawala et al., Reference Mwatawala, Virgilio, Joseph and De Meyer2015), probably reflecting differences in the developmental and survival rates of the two species in respect to climate variation (Tanga et al., Reference Tanga, Manrakhan, Daneel, Mohamed, Fathiya and Ekesi2015). It should be noted that C. quilicii is the only one of the two sibling species found in the southernmost parts of Africa where the climate is more temperate (De Meyer et al., Reference De Meyer, Delatte, Ekesi, Jordaens, Kalinová, Manrakhan, Mwatawala, Steck, Van Cann, Vaničhová, Břizová and Virgilio2015a). However, because of the recent separation into different species, the specific distribution patterns for C. rosa and C. quilicii may need reevaluation. Among the FARQ pests, C. rosa and C. quilicii are the most aggressive ones causing significant destruction to a large variety of crops and presenting high expansion potential. Already, C. quilicii has been introduced in the Islands of Mauritius and La Réunion (White et al., Reference White, De Meyer and Stonehouse2000; De Meyer et al., Reference De Meyer, Mwatawala, Copeland and Virgilio2016) and a great concern has arisen about their possible expansion to more temperate climates outside Africa, since they can survive in a wide temperature and altitude range (Duyck and Quilici, Reference Duyck and Quilici2002; Copeland et al., Reference Copeland, Wharton, Luke, De Meyer, Lux, Zenz, Machera and Okumu2006; Geurts et al., Reference Geurts, Mwatawala and De Meyer2012; de Villiers et al., Reference de Villiers, Hattingh and Kriticos2013; Tanga et al., Reference Tanga, Khamis, Tonnang, Rwomushana, Mosomtai, Mohamed and Ekesi2018).
The species of the FARQ complex are extremely similar in morphology; males are hardly identified by subtle differences in the setal ornamentation and pigmentation of mid femur and tibia, while females are practically indistinguishable (De Meyer et al., Reference De Meyer, Delatte, Ekesi, Jordaens, Kalinová, Manrakhan, Mwatawala, Steck, Van Cann, Vaničhová, Břizová and Virgilio2015a, Reference De Meyer, Mwatawala, Copeland and Virgilio2016). Species delimitation and phylogenetic relationships among the four taxa are not fully resolved. Several approaches have been undertaken toward this direction including morphometrics (Van Cann et al., Reference Van Cann, Virgilio, Jordaens and De Meyer2015), interspecies hybridization and estimation of developmental stability (Erbout et al., Reference Erbout, De Meyer and Lens2008), biochemical characterization of pheromones and cuticular hydrocarbons (Vaníčková et al., Reference Vaníčková, Virgilio, Tomčala, Břízová, Ekesi, Hoskovec, Kalinová, Do Nascimento and De Meyer2014, Reference Vaníčková, Břízová, Mendonça, Pompeiano and Do Nascimento2015; Břízová et al., Reference Břízová, Vaníčková, Faťarová, Ekesi, Hoskovec and Kalinová2015), and molecular/genetic data of nuclear and mitochondrial sequences (Douglas and Haymer, Reference Douglas and Haymer2001; Barr and McPheron, Reference Barr and McPheron2006; Barr et al., Reference Barr, Copeland, De Meyer, Masiga, Kibogo, Billah, Osir, Wharton and McPheron2006, Reference Barr, Islam, De Meyer and McPheron2012; Virgilio et al., Reference Virgilio, Backeljau, Barr and De Meyer2008, Reference Virgilio, Jordaens, Breman, Backeljau and De Meyer2012), with the analysis of a specific microsatellite set conferring better resolution among populations of the complex (Delatte et al., Reference Delatte, Virgilio, Simiand, Quilici and De Meyer2013; Virgilio et al., Reference Virgilio, Delatte, Quilici, Backeljau and De Meyer2013, Reference Virgilio, Daneel, Manrakhan, Delatte, Meganck and De Meyer2019). Recently, a phylogenomic study based on genome-wide SNP analysis provided consistent resolution and better insights into the phylogenetic relationships of the FARQ members (Zhang et al., Reference Zhang, De Meyer, Virgilio, Feng, Badji and Li2021). A good understanding of the evolutionary relationships and the development of accurate, simple, and fast diagnostic tools for the sibling species of the FARQ complex is of great importance for the implementation of quarantine measures, as well as for biological control applications, including the sterile insect technique (SIT), against these pests.
The number and structure of chromosomes are fundamental genetic characteristics of species, while chromosome rearrangements are considered to play a major role in speciation. In Diptera, the occurrence of polytene nuclei in several juvenile tissues has greatly facilitated the study of chromosomes due to their enormous size and consistent banding pattern (Zhimulev and Koryakov, Reference Zhimulev and Koryakov2009). Numerous cytogenetic studies in Drosophila but also in mosquitoes have explored the evolutionary changes of chromosome structure among related species and, together with modern genomic data, substantiated that chromosome rearrangements and especially paracentric inversions promote speciation, mainly through suppressing recombination and, thus, preserving sets of co-adapted alleles, and suggested that they could be used as phylogenetic markers (Sturtevant and Dobzhansky, Reference Sturtevant and Dobzhansky1936; Coluzzi et al., Reference Coluzzi, Sabatini, Petrarca and Di Deco1979; Krimbas and Powell, Reference Krimbas and Powell1992; Noor et al., Reference Noor, Grams, Bertucci and Reiland2001; Rieseberg, Reference Rieseberg2001; Kirkpatrick and Barton, Reference Kirkpatrick and Barton2006; Kulathinal et al., Reference Kulathinal, Stevison and Noor2009; Faria and Navarro, Reference Faria and Navarro2010; McGaugh and Noor, Reference McGaugh and Noor2012; Lee et al., Reference Lee, Collier, Sanford, Marsden, Fofana, Cornel and Lanzaro2013). In Tephritidae, as well, differences in the size and structure of mitotic sex chromosomes have been descripted as diagnostic characters among closely related species (Hunwattanakul and Baimai, Reference Hunwattanakul and Baimai1994; Baimai et al., Reference Baimai, Trinachartvanit, Tigvattananont, Grote, Poramarcom and Kijchalao1995, Reference Baimai, Phinchonsgsakuldit, Sumrandee and Tigvattananont2000; Baimai, Reference Baimai1998; Goday et al., Reference Goday, Selivon, Perondini, Greciano and Ruiz2006; Cáceres et al., Reference Cáceres, Segura, Vera, Wornoayporn, Cladera, Teal, Sapountzis, Bourtzis, Zacharopoulou and Robinson2009; Hernández-Ortiz et al., Reference Hernández-Ortiz, Bartolucci, Morales-Valles, Frías and Selivon2012; Giardini et al., Reference Giardini, Milla, Lanzavecchia, Nieves and Cladera2015). Furthermore, comparative analyses of polytene chromosomes have identified specific rearrangements that could distinguish between genera, subgenera, or species (Augustinos et al., Reference Augustinos, Drosopoulou, Gariou-Papalexiou, Asimakis, Cáceres, Tsiamis, Bourtzis, Mavragani-Tsipidou and Zacharopoulou2015; Zacharopoulou et al., Reference Zacharopoulou, Augustinos, Drosopoulou, Tsoumani, Gariou-Papalexiou, Franz, Mathiopoulos, Bourtzis and Mavragani-Tsipidou2017; Gouvi et al., Reference Gouvi, Gariou-Papalexiou, Augustinos, Drosopoulou, Tsiamis, Bourtzis and Zacharopoulou2022). Cytogenetic information on tephritid pests has also been proved valuable for the development and characterization of genetic sexing strains essential for the implementation of certain control methods, such as SIT (Augustinos et al., Reference Augustinos, Drosopoulou, Gariou-Papalexiou, Asimakis, Cáceres, Tsiamis, Bourtzis, Mavragani-Tsipidou and Zacharopoulou2015; Zacharopoulou et al., Reference Zacharopoulou, Augustinos, Drosopoulou, Tsoumani, Gariou-Papalexiou, Franz, Mathiopoulos, Bourtzis and Mavragani-Tsipidou2017; Gouvi et al., Reference Gouvi, Gariou-Papalexiou, Augustinos, Drosopoulou, Tsiamis, Bourtzis and Zacharopoulou2022). Even so, taking into consideration that speciation is a complex procedure driven by variable factors one can understand that chromosome structure and cytogenetics could only be one of multiple tools for species delimitation. Especially in cases of recent or ongoing speciation, pools of independent data in the context of ‘integrative taxonomy’ (Schutze et al., Reference Schutze, Virgilio, Norrbom and Clarke2017a, Reference Schutze, Bourtzis, Cameron, Clarke, de Meyer, Hee, Hendrichs, Krosch and Mwatawala2017b) and modern genome-wide analyses (Zhang et al., Reference Zhang, De Meyer, Virgilio, Feng, Badji and Li2021) are necessary for clearer perception.
In this study, we describe the mitotic and polytene chromosome of C. rosa and C. quilicii and we conduct a comparative polytene chromosome analysis among the above species and C. fasciventris F2 by observation of polytene nuclei of each species as well as of F1 hybrids between them. Furthermore, we localized the hsp70 gene on the polytene chromosomes of the above species, since rearrangements which include the chromosome region where the hsp70 locus resides on the 3L polytene arm seem to be common among several tephritid species (Drosopoulou et al., Reference Drosopoulou, Pantelidou, Gariou-Papalexiou, Augustinos, Chartomatsidou, Kyritsis, Bourtzis, Mavragani-Tsipidou and Zacharopoulou2017; Zacharopoulou et al., Reference Zacharopoulou, Augustinos, Drosopoulou, Tsoumani, Gariou-Papalexiou, Franz, Mathiopoulos, Bourtzis and Mavragani-Tsipidou2017), some of them closely related (Gouvi et al., Reference Gouvi, Gariou-Papalexiou, Augustinos, Drosopoulou, Tsiamis, Bourtzis and Zacharopoulou2022). Our aim is to reveal possibly existing chromosome rearrangements that could be informative toward the better understanding of the phylogenetic relationships of the species and could be used as discriminating characters for species identification within the FARQ complex.
Materials and methods
Insects from five colonies maintained at the Insect Pest Control Laboratory (IPCL), Seibersdorf, Austria were used in the present study. The above colonies were established from insects originating from confirmed colonies of Ceratitis fasciventris F2 (hereafter C. fasciventris), C. rosa and C. quilicii maintained at ICIPE, Kenya and of C. rosa and C. quilicii maintained at CRI, South Africa. The colonies were reared under controlled temperature, humidity, and light conditions, as previously described (Drosopoulou et al., Reference Drosopoulou, Pantelidou, Gariou-Papalexiou, Augustinos, Chartomatsidou, Kyritsis, Bourtzis, Mavragani-Tsipidou and Zacharopoulou2017).
Mitotic chromosome preparations were spread from nerve ganglia of third instar larvae following the air-drying technique described in Mavragani-Tsipidou et al. (Reference Mavragani-Tsipidou, Zacharopoulou, Drosopoulou, Augustinos, Bourtzis, Marec and Sharakhov2014). Brain tissue was dissected in physiological solution, treated with hypotonic solution (1% sodium citrate) for about 15 min and fixed in fresh fixation solution (methanol/acetic acid 3:1) for 3 min. Samples were macerated in a small drop of 60% acetic acid, dripped onto a clean slide and placed on a hotplate (40–45 °C). After air-drying, preparations were stained in Giemsa solution (5% Giemsa in 10 mM phosphate buffer, pH 6.8) and observed with 100× magnification objective lens, using a phase contrast microscope (Leica DMR). Well spread nuclei were photographed using a CCD camera (ProgResCFcool; JENOPTIK Jena Optical Systems, Jena, Germany). About ten chromosome preparations from individual larvae from each strain and at least ten well spread nuclei per preparation were analyzed.
Polytene chromosome preparations for banding pattern analysis were made from salivary glands of third-instar larvae as described in Mavragani-Tsipidou et al. (Reference Mavragani-Tsipidou, Zacharopoulou, Drosopoulou, Augustinos, Bourtzis, Marec and Sharakhov2014). Salivary glands were dissected in 45% acetic acid, transferred to 3N HCL for 1 min, and fixed in fixation solution (3 parts glacial acetic acid: 2 parts water: 1 part lactic acid) for about 5 min. Staining was performed in lacto-acetic-orcein for 5–7 min. After excess stain was removed, the glands were squashed in lacto-acetic acid. About 50 chromosome slides from each strain were prepared and well spread nuclei and/or isolated chromosomes were observed at 63× and 100× objectives in a phase contrast microscope (Leica DMR) and photographed using a CCD camera (ProgResCFcool; JENOPTIK Jena Optical Systems).
Polytene chromosome preparations for in situ hybridization were made following the procedure described by Mavragani-Tsipidou et al. (Reference Mavragani-Tsipidou, Zacharopoulou, Drosopoulou, Augustinos, Bourtzis, Marec and Sharakhov2014). A genomic fragment of the hsp70 gene of Ceratitis capitata (Papadimitriou et al., Reference Papadimitriou, Kritikou, Mavroidis, Zacharopoulou and Mintzas1998) was used as probe. Labeling of the probe and detection of the signal was performed using the ‘DIG-DNA Labeling and Detection kit’ purchased by ROCHE, Mannheim, Germany and following the protocol described in Mavragani-Tsipidou et al. (Reference Mavragani-Tsipidou, Zacharopoulou, Drosopoulou, Augustinos, Bourtzis, Marec and Sharakhov2014). Hybridization was performed at 65 °C. Five preparations and at least ten well spread nuclei per preparation were observed at 100× magnification with a Leica DMR phase contrast microscope equipped with a CCD camera (ProgResCFcool, JENOPTIK Jena Optical Systems).
Results and discussion
Mitotic chromosomes
The karyotypes of C. rosa and C. quilicii (2n = 12) appear identical to each other consisting of five pairs of autosomes and one pair of heteromorphic sex chromosomes (XX/XY) (fig. 1). The largest metacentric (chromosome 2), as well as the only submetacentric (chromosome 3) autosome pair can be easily identified (fig. 1). The remaining three autosomes (namely 4, 5, and 6) being all metacentric of similar size cannot be easily distinguished by our analysis. The two sex chromosomes differ significantly in size: the X chromosome is submetacentric of medium size, while Y is a small metacentric chromosome (fig. 1b, d). The karyotypes of C. rosa and C. quilicii, presented after Giemsa staining, are in agreement with the C. rosa karyotype described by Willhoeft and Franz (Reference Willhoeft and Franz1996). They also appear very similar to the mitotic karyotype of the closely related member of the FARQ complex, C. fasciventris (Drosopoulou et al., Reference Drosopoulou, Pantelidou, Gariou-Papalexiou, Augustinos, Chartomatsidou, Kyritsis, Bourtzis, Mavragani-Tsipidou and Zacharopoulou2017), in which the X chromosome seems to be of slightly smaller size (relatively to the autosomes). Similarly, the main difference of all FARQ karyotypes to C. capitata is the considerably shorter X and Y chromosomes. Such variation in the size of the sex chromosomes, reflecting differences of the amount of heterochromatin, can be commonly observed among very closely related, e.g. within a complex (Baimai et al., Reference Baimai, Trinachartvanit, Tigvattananont, Grote, Poramarcom and Kijchalao1995, Reference Baimai, Phinchonsgsakuldit, Sumrandee and Tigvattananont2000; Selivon et al., Reference Selivon, Perondini and Morgante2005a; Cáceres et al., Reference Cáceres, Segura, Vera, Wornoayporn, Cladera, Teal, Sapountzis, Bourtzis, Zacharopoulou and Robinson2009) or a bit more distantly related, e.g. within a genus, (Hunwattanakul and Baimai, Reference Hunwattanakul and Baimai1994; Frias, Reference Frias2004; Selivon et al., Reference Selivon, Perondini and Rocha2005b; Zacharopoulou et al., Reference Zacharopoulou, Augustinos, Drosopoulou, Tsoumani, Gariou-Papalexiou, Franz, Mathiopoulos, Bourtzis and Mavragani-Tsipidou2017) species of tephritids.
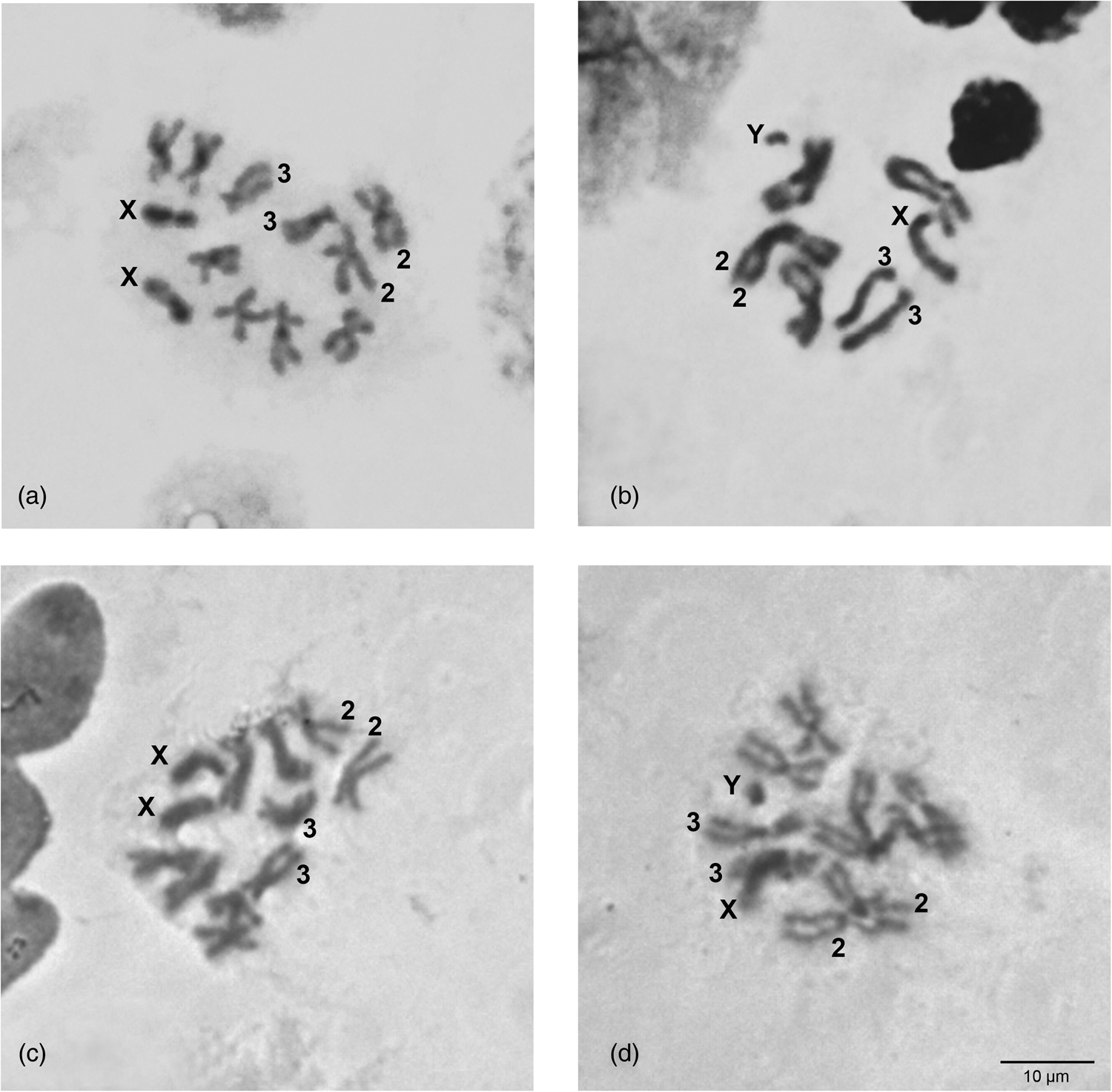
Figure 1. Mitotic karyotypes of C. rosa (a and b) and C. quilicii (c and d). (a, c) Female; (b, d) male. The sex chromosomes, X and Y, as well as the autosomes 2 and 3 are shown.
Polytene chromosomes
The salivary gland polytene nuclei of two C. rosa colonies and two C. quilicii colonies have been studied. Analysis showed that the polytene complement of the above species consists of ten long polytene arms with distinct banding pattern, corresponding to the five autosomes, while a dispersed heterochromatic network represents the under-replicated sex chromosomes (Supplementary figs 1 and 2), similarly to other Tephritidae species (Zacharopoulou et al., Reference Zacharopoulou, Augustinos, Drosopoulou, Tsoumani, Gariou-Papalexiou, Franz, Mathiopoulos, Bourtzis and Mavragani-Tsipidou2017; Gouvi et al., Reference Gouvi, Gariou-Papalexiou, Augustinos, Drosopoulou, Tsiamis, Bourtzis and Zacharopoulou2022). Although no typical chromocenter was observed, the centromeric region of different chromosomes could be found loosely connected (Supplementary fig. 1a). Chromosomes were numbered from 2 to 6, chromosome arms were designated as L or R (Supplementary figs 1 and 2) based on the similarities to C. fasciventris polytene chromosome maps (Drosopoulou et al., Reference Drosopoulou, Pantelidou, Gariou-Papalexiou, Augustinos, Chartomatsidou, Kyritsis, Bourtzis, Mavragani-Tsipidou and Zacharopoulou2017) and following the numbering system proposed for the polytene chromosomes of the medfly, the first tephritid species analyzed cytogenetically (Zacharopoulou et al., Reference Zacharopoulou, Augustinos, Drosopoulou, Tsoumani, Gariou-Papalexiou, Franz, Mathiopoulos, Bourtzis and Mavragani-Tsipidou2017).
Detailed comparison of the polytene chromosome banding pattern failed to reveal differences either among the analyzed strains of C. rosa and C. quilicii nor between each analyzed strain and C. fasciventris (Supplementary figs 3 and 4). Aiming to confirm the identical banding pattern of the analyzed species, the polytene chromosomes of F1 hybrids between C. rosa and C. quilicii, as well as between C. rosa and C. fasciventris and C. quilicii and C. fasciventris were also examined. The analysis of the polytene nuclei of the hybrids did not reveal any chromosome rearrangements between the parental strains. Synapsis of the homologous chromosomes was almost perfect in the hybrids between C. rosa and C. quilicii (fig. 2), while in the hybrids with C. fasciventris minor polymorphic asynapses were observed (Supplementary figs 5 and 6). Asynapses were mainly located at or close to the telomeric and the centromeric regions of the polytene arms and their extent was limited although it could vary among different nuclei (figs 3 and 4). The number and frequency of minor asynaptic sites were higher in the hybrids between C. rosa and C. fasciventris compared to the ones between C. quilicii and C. fasciventris. The most evident asynapses were the ones at the tips of chromosome arms 2L, 2R, 3L, 4R, and 6R (figs 3 and 4).
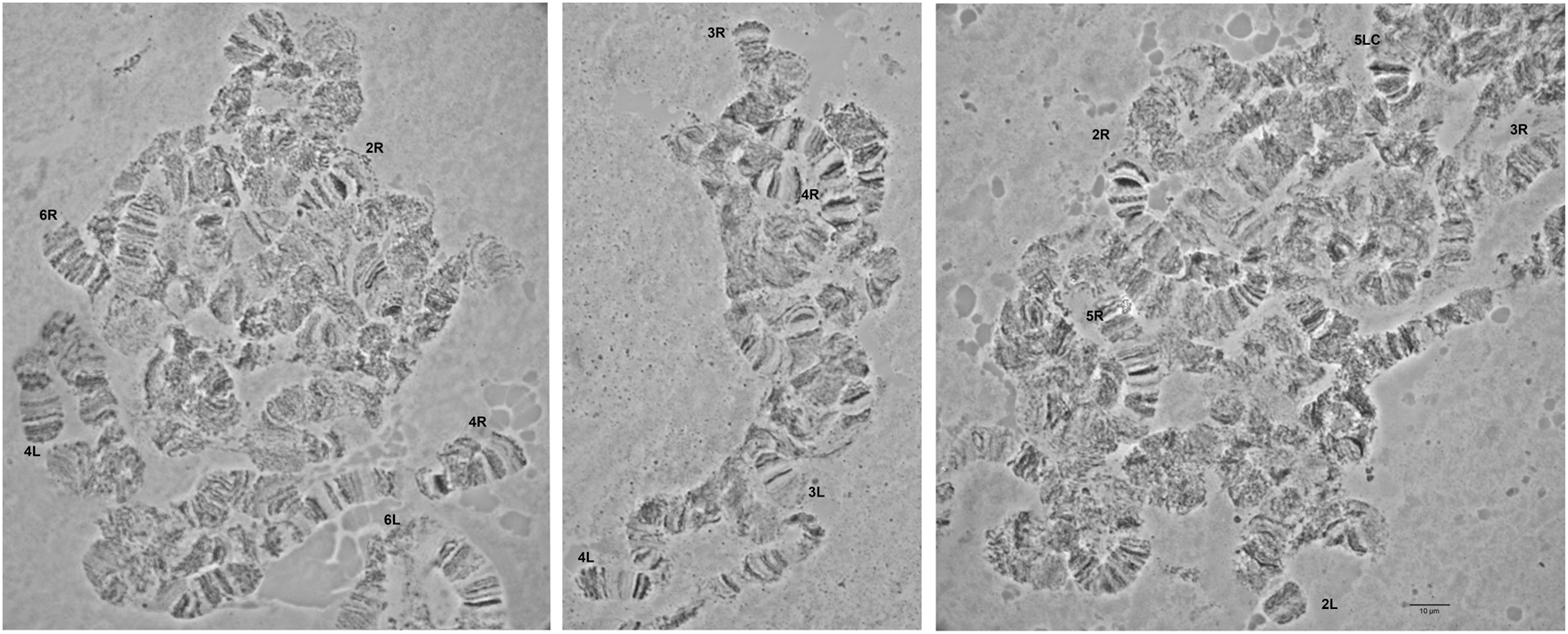
Figure 2. Polytene nuclei of F1 hybrids between C. rosa and C. quilicii. The telomeres of the polytene elements are indicated. 5LC indicates the 5L centromere. No asynapses are observed.
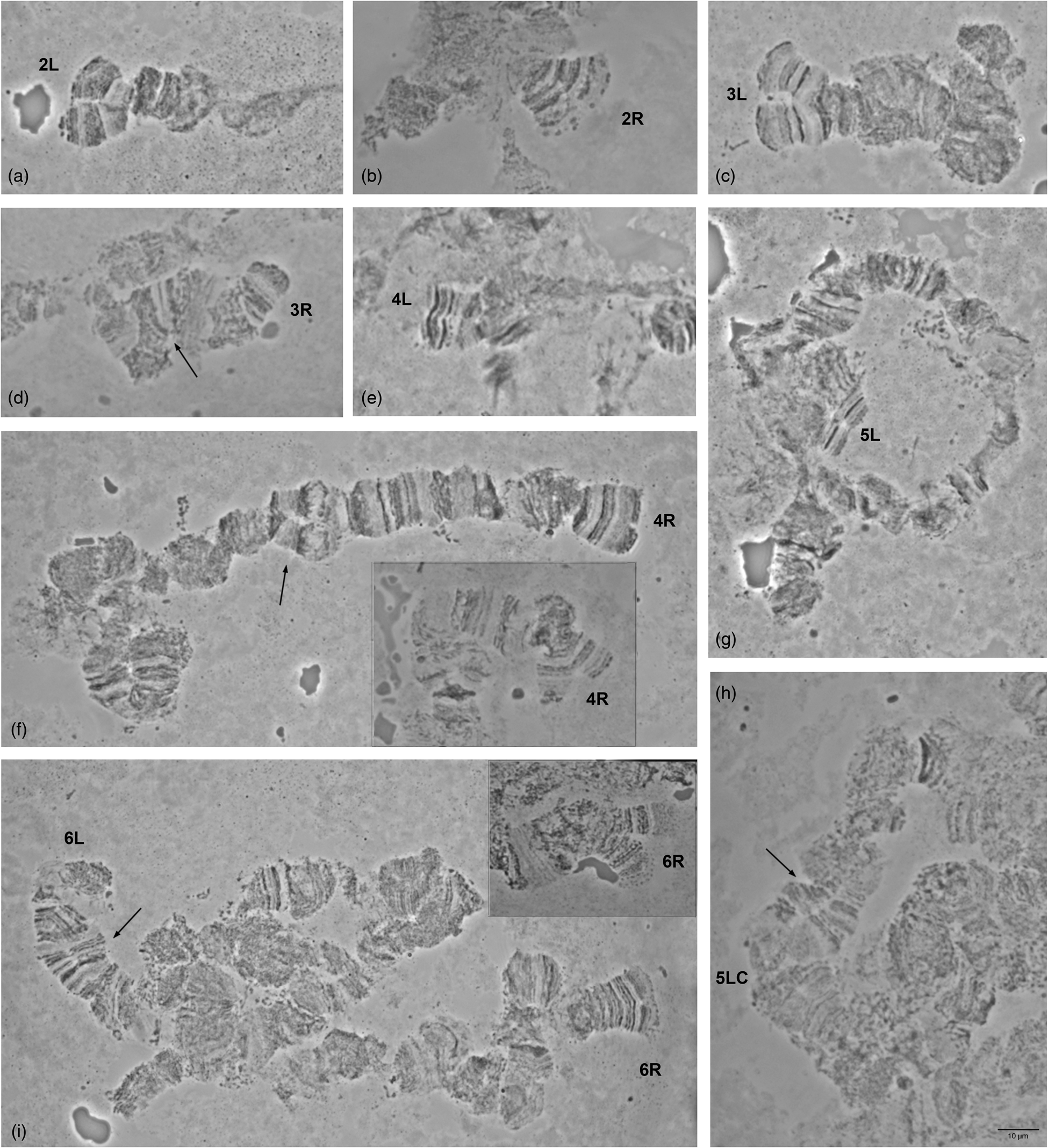
Figure 3. Asynapses frequently observed in the nuclei of F1 hybrids between C. rosa and C. fasciventris. The asynaptic telomeres of the polytene elements are indicated. Variable extent of asynapsis observed for 4R and 6R telomeres is presented in (f) and (i), respectively. Arrows indicate asynapses in the inner parts of the polytene elements. 5LC indicates the 5L centromere.
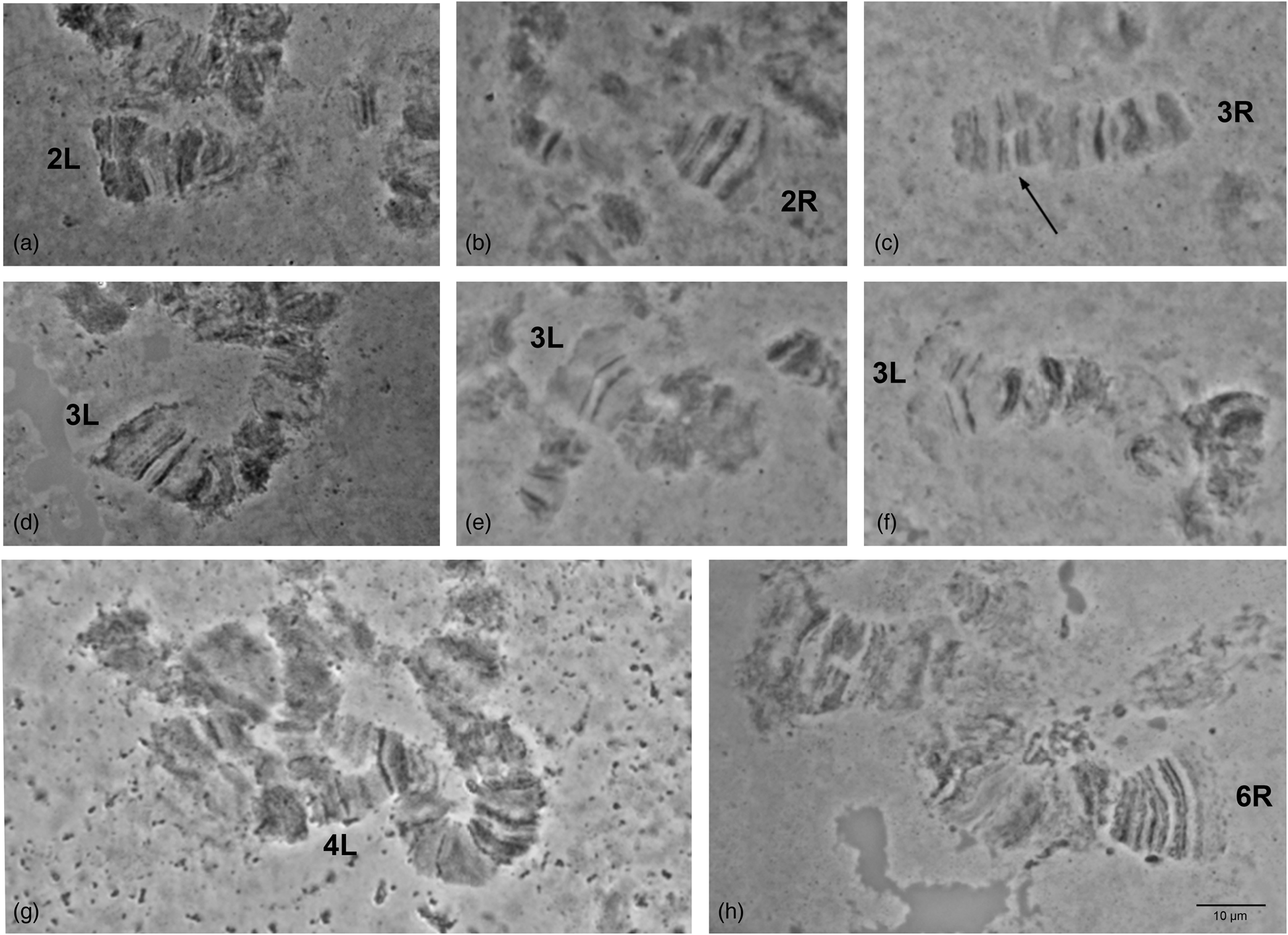
Figure 4. Asynapses frequently observed in the nuclei of F1 hybrids between C. quilicii and C. fasciventris. The asynaptic telomeres of the polytene elements are indicated. Variable extent of asynapsis observed for 3L telomere is presented in (d–f). Arrows indicate asynapses in the inner parts of the polytene elements.
The above observations indicate that the chromosomes of C. rosa and C. quilicii, at least at the banding pattern level, can be considered as homosequential to each other and to C. fasciventris and the available polytene chromosome maps of C. fasciventris (Drosopoulou et al., Reference Drosopoulou, Pantelidou, Gariou-Papalexiou, Augustinos, Chartomatsidou, Kyritsis, Bourtzis, Mavragani-Tsipidou and Zacharopoulou2017) is suggested to be used as reference map for the three FARQ species.
The lack of detectable differences in the mitotic and polytene chromosomes of the three FARQ species indicates that they are very close genetically. This is also supported by previous molecular genetic studies, including analysis of nuclear and mitochondrial fragments, DNA barcoding and analysis of complete mitogenomes, that couldn't resolve phylogeny or provide robust discriminating tools for the members of the complex (Virgilio et al., Reference Virgilio, Backeljau, Barr and De Meyer2008, Reference Virgilio, Jordaens, Breman, Backeljau and De Meyer2012; Barr et al., Reference Barr, Islam, De Meyer and McPheron2012; Drosopoulou et al., Reference Drosopoulou, Pantelidou, Gariou-Papalexiou, Augustinos, Chartomatsidou, Kyritsis, Bourtzis, Mavragani-Tsipidou and Zacharopoulou2017, Reference Drosopoulou, Damaskou, Markou, Ekesi, Khamis, Manrakhan, Augustinos, Tsiamis and Bourtzis2021). The limitations of the above approaches seem to be overcome only by genome-wide sequencing data succeeding to provide a robust phylogenetic inference within the complex (Zhang et al., Reference Zhang, De Meyer, Virgilio, Feng, Badji and Li2021). Absence of chromosomal rearrangements has also been observed between the two members of the B. dorsalis complex, namely B. dorsalis and B. carambolae (Augustinos et al., Reference Augustinos, Drosopoulou, Gariou-Papalexiou, Asimakis, Cáceres, Tsiamis, Bourtzis, Mavragani-Tsipidou and Zacharopoulou2015), however, within other species complexes of Tephritidae chromosome differences have been used as differentiating characters and revealed incipient speciation (Selivon et al., Reference Selivon, Perondini and Rocha2005b, Reference Selivon, Perondini and Morgante2005a; Goday et al., Reference Goday, Selivon, Perondini, Greciano and Ruiz2006; Cáceres et al., Reference Cáceres, Segura, Vera, Wornoayporn, Cladera, Teal, Sapountzis, Bourtzis, Zacharopoulou and Robinson2009; Hernández-Ortiz et al., Reference Hernández-Ortiz, Bartolucci, Morales-Valles, Frías and Selivon2012).
Chromosome localization of the hsp70 gene
The hsp70 gene has been localized on the polytene chromosome of C. rosa and C. quilicii. A unique hybridization signal has been identified on the same chromosomal position (3L polytene chromosome arm, region 27) in all strains tested (fig. 5). The localization site of the hsp70 gene on C. rosa and C. quilicii is identical to the one observed in C. fasciventris (Drosopoulou et al., Reference Drosopoulou, Pantelidou, Gariou-Papalexiou, Augustinos, Chartomatsidou, Kyritsis, Bourtzis, Mavragani-Tsipidou and Zacharopoulou2017) (fig. 5), supporting the homosequentiality of the chromosomes of the three members of the FARQ complex. Nevertheless, it is acknowledged that the localization of a much greater number of probes is required to draw conclusions about genomic synteny among the studied species.

Figure 5. In situ hybridization of the hsp70 gene probe on the salivary gland polytene chromosomes of C. rosa and C. quilicii. Arrows indicate the hybridization signals. The telomere of the 3L polytene arm is indicated. Numbered divisions are shown, separated by lines. The reference map of the 3L arm and the hybridization locus of the hsp70 gene of C. fasciventris (Drosopoulou et al., Reference Drosopoulou, Pantelidou, Gariou-Papalexiou, Augustinos, Chartomatsidou, Kyritsis, Bourtzis, Mavragani-Tsipidou and Zacharopoulou2017) are presented on the top.
In comparison to C. capitata, the site of the hsp 70 gene is different in the FARQ complex species indicating intrachromosomal rearrangements (Drosopoulou et al., Reference Drosopoulou, Pantelidou, Gariou-Papalexiou, Augustinos, Chartomatsidou, Kyritsis, Bourtzis, Mavragani-Tsipidou and Zacharopoulou2017) that have differentiated the structure of the 3L chromosome arm of the above species. The presence of rearrangements, such as translocations and inversions, in the 3L polytene arm has also been revealed by previous comparative analyses among species of several Tephritidae genera (Zacharopoulou et al., Reference Zacharopoulou, Augustinos, Drosopoulou, Tsoumani, Gariou-Papalexiou, Franz, Mathiopoulos, Bourtzis and Mavragani-Tsipidou2017; Gouvi et al., Reference Gouvi, Gariou-Papalexiou, Augustinos, Drosopoulou, Tsiamis, Bourtzis and Zacharopoulou2022), supporting the role of chromosome rearrangements in speciation (Noor et al., Reference Noor, Grams, Bertucci and Reiland2001; Rieseberg, Reference Rieseberg2001; Kirkpatrick and Barton, Reference Kirkpatrick and Barton2006; Faria and Navarro, Reference Faria and Navarro2010; McGaugh and Noor, Reference McGaugh and Noor2012; Lee et al., Reference Lee, Collier, Sanford, Marsden, Fofana, Cornel and Lanzaro2013) and their potential informativeness for phylogenetic inference among related tephritid species (Mavragani-Tsipidou et al., Reference Mavragani-Tsipidou, Zacharopoulou, Drosopoulou, Augustinos, Bourtzis, Marec and Sharakhov2014; Zacharopoulou et al., Reference Zacharopoulou, Augustinos, Drosopoulou, Tsoumani, Gariou-Papalexiou, Franz, Mathiopoulos, Bourtzis and Mavragani-Tsipidou2017; Gouvi et al., Reference Gouvi, Gariou-Papalexiou, Augustinos, Drosopoulou, Tsiamis, Bourtzis and Zacharopoulou2022).
Conclusions
Our comparative mitotic and polytene chromosome analysis of the colonized material of C. rosa and C. quilicii from two different African locations (Kenya and South Africa) and of C. fasciventris from Kenya did not unravel any detectable fixed chromosome rearrangements among the three members of the FARQ complex. The above emphasizes the need for multidisciplinary modern approaches when addressing sensitive issues of species designation within complexes of important insect pests, as only by the accumulation and evaluation of data coming from different aspects of the insect biology we can be led toward a more solid phylogenetic resolution and reliable species identification.
Supplementary material
The supplementary material for this article can be found at https://doi.org/10.1017/S0007485323000214.
Acknowledgements
We thank Drs Sunday Ekesi, Fathiya Khamis, and Aruna Manrakhan for providing the biological material from Kenya and South Africa for the establishment of the insect colonies used in the present study.
Author contributions
E. D., A. A., K. B., and A. Z. designed the study. G. G., E. D., A. G.-P., and A. Z. performed the experiments. G. G., A. G.-P., and A. Z. took the pictures. G. G., E.D., A. G.-P., A. A., and A. Z. interpreted and analyzed the data. A. G.-P. prepared the figures. E. D. wrote the original draft manuscript. A. A. and K. B. critically revised the manuscript. All authors reviewed and approved the final version of the manuscript.
Financial support
The present study has been funded by the Insect Pest Control Subprogram of the Joint FAO/IAEA Centre of Nuclear Techniques in Food and Agriculture through a research contract entitled ‘Cytogenetic analysis of members of Tephritidae complex species and cytogenetic characterization of Genetic Sexing Strains’ (contract number: 20674).
Competing interest
None.