Vitamin D status is assessed by the concentration of serum 25-hydroxyvitamin D (25(OH)D), a circulating vitamin D metabolite( Reference Holick 1 ). Humans obtain vitamin D from exposure to sunlight and through vitamin D intake, with sunlight being the primary source( Reference Holick 1 ). Vitamin D facilitates intestinal Ca absorption( Reference Bouillon, Van Cromphaut and Carmeliet 2 , Reference Christakos 3 ), and severe deficiency is detrimental to childhood bone health( Reference Wharton and Bishop 4 ). In addition, vitamin D status in childhood has been inversely associated with cardiometabolic risk markers such as glucose, insulin and blood pressure( Reference Dolinsky, Armstrong and Mangarelli 5 ). These associations have been suggestively explained by a potential favourable role of active vitamin D, 1,25-dihydroxyvitamin D (1,25(OH)2D), in cardiometabolic mechanisms such as blood pressure regulation through the renin–angiotensin system( Reference Li 6 ), pancreatic β-cell function and protection( Reference Wolden-Kirk, Overbergh and Christesen 7 , Reference Wolden-Kirk, Rondas and Bugliani 8 ), and low-grade inflammation suppression( Reference Querfeld 9 , Reference Marcotorchino, Gouranton and Romier 10 ), as well as by an inverse association with parathyroid hormone (PTH), an apparent predictor of inflammation, dyslipidaemia and cardiovascular calcifications in obese children and adolescents( Reference Alemzadeh and Kichler 11 , Reference Gilardini, Pasqualinotto and Di Matteo 12 ).
Excess body weight and fat mass in childhood increase the risk of the metabolic syndrome (MetS), a cluster of elevated risk factors including insulin resistance, high blood pressure, deranged lipid profile and abdominal obesity, that has been associated with CVD risk in adults( Reference Zimmet, Alberti and Kaufman 13 , Reference Nadeau, Maahs and Daniels 14 ). BMI is consistently inversely associated with vitamin D status in adults( Reference Saneei, Salehi-Abargouei and Esmaillzadeh 15 ). This association has also been demonstrated in overweight and obese children( Reference Pacifico, Anania and Osborn 16 – Reference Alemzadeh, Kichler and Babar 18 ), and in American and Spanish children of primarily normal weight( Reference Olson, Maalouf and Oden 19 – Reference Codoner-Franch, Tavarez-Alonso and Simo-Jorda 22 ), although not all studies agree( Reference Aypak, Turedi and Yuce 23 , Reference Sacheck, Goodman and Chui 24 ). Relations between vitamin D status and cardiometabolic risk markers may be mediated or confounded by fat mass, which in turn may be associated with vitamin D status through sequestering of vitamin D in adipose tissue( Reference Earthman, Beckman and Masodkar 25 ) or by reverse causality – that is, through poorer diets and less sun exposure in overweight subjects leading to lower vitamin D status. Therefore, adjustment for the potential confounding effects of fat mass is important when evaluating associations between vitamin D status and cardiometabolic health. Most studies in children have used BMI, which does not convey information on body composition, and more sophisticated measurements of body fat are called for in observational studies( Reference Earthman, Beckman and Masodkar 25 ). Moreover, as physical activity, especially of moderate-to-vigorous physical activity (MVPA), has been associated both with cardiometabolic risk markers( Reference Ekelund, Luan and Sherar 26 ) and with increased sun exposure and vitamin D status in children( Reference Ha, Cho and Lee 27 ), it should be considered a potential confounder.
The aim of this cross-sectional study was to (1) investigate associations between vitamin D status and cardiometabolic risk markers, and (2) investigate whether these associations were independent of objectively measured fat mass index (FMI) and physical activity, in a large cohort of Danish 8–11-year-old school children.
Methods
Study design and participants
The present study used cross-sectional baseline data from the Optimal well-being, development and health for Danish children through a healthy New Nordic Diet (OPUS) School Meal Study, a large cluster-randomised controlled cross-over trial including third- and fourth-grade children from nine schools in Denmark. The primary aim of the OPUS School Meal Study was to evaluate the impact of school meals based on the New Nordic Diet on a MetS score and cognitive concentration performance, as previously described( Reference Damsgaard, Dalskov and Petersen 28 ). Briefly, initial contact was established with thirty-nine schools whereof nine schools, located in eight separate urban and rural municipalities, were included. Inclusion criteria for the schools were (a) location in the eastern part of Denmark; (b) a total of four or more classes at third and fourth grade; (c) suitable kitchen facilities available for food production during school days; and (d) a high motivation for participation as determined by the study team. Written information about the study was sent to the families of all 1021 third- and fourth-grade children at the schools. To recruit families of various socioeconomic and ethnic backgrounds, we held several information meetings at each school; sent out several reminders; and had translators present at meetings when relevant. Exclusion criteria for the children were (a) diseases or conditions that might obstruct the measurements or put a child at risk by eating the intervention school meals, or (b) concomitant participation in other studies that involved radiation or blood sampling. Written informed consent for participation was obtained from the custody holders of 834 children (82 %). The study was conducted in accordance with the Declaration of Helsinki, and the study protocol was approved by the Committees on Biomedical Research Ethics for the Capital Region of Denmark (H-1-2010-124) and registered with clinicaltrials.gov (NCT 01577277).
Questionnaires
Information on demographic and socioeconomic characteristics was collected by an in-depth 2 h personal interview with the child and parents. On the basis of the highest education level attained in the household, parental education level was categorised as lower secondary education (≤10 years of schooling), upper secondary education, vocational education, short higher education, bachelor’s degree or equivalent or ≥master’s degree (≥17 years of schooling). Children were categorised as immigrants/descendants if all grandparents and ≥1 parent were born outside Denmark. This classification was inspired by the definitions of immigrants and descendants used by Statistics Denmark( 29 ), although not identical as citizenship was not available in the present study. Pubertal status was self-evaluated by the child based on breast development in girls and pubic hair in boys (Tanner stages)( Reference Morris and Udry 30 ). Registrations were dichotomised into a variable indicating entered puberty (yes/no).
Recordings of dietary intake and physical activity
During the weeks before the clinical examination, children, aided by their parents, recorded their daily intake of food and beverages for 7 consecutive days using a Web-based Dietary Assessment Software for Children. The software was developed specifically for children aged 8–11 years and validated in the OPUS School Meal Pilot Study( Reference Biltoft-Jensen, Trolle and Christensen 31 , Reference Biltoft-Jensen, Hjorth and Trolle 32 ). Intake data were processed as previously described( Reference Biltoft-Jensen, Trolle and Christensen 31 ). Energy intake (EI) relative to estimated BMR was evaluated following the equations by Henry( Reference Henry 33 ), and energy under-reporters (EI/BMR≤1·05) and over-reporters (EI/BMR≥2·28)( Reference Black 34 ) were excluded from the dietary variables, as were children who registered their diet for <4 d. Intake of dietary supplements was registered and a dichotomous variable was formed, indicating whether the child had ingested any kind of vitamin D-containing dietary supplements, including multivitamins, during the dietary registration week. To measure physical activity, the children were asked to wear an accelerometer (ActiGraph GT3X+ Tri-Axis Accelerometer Monitor) in an elastic belt tightly fastened at the right hip for the same 7 d and eight nights as the dietary recordings, and to remove it only during water activities – that is, showering or swimming. Analyses of the physical activity data were described in detail previously( Reference Hjorth, Chaput and Michaelsen 35 ). We used time spent with MVPA, defined as ≥2296 vertical counts/min( Reference Trost, Loprinzi and Moore 36 ), as the measure of physical activity, as this has shown stronger associations with cardiometabolic risk markers( Reference Ekelund, Luan and Sherar 26 ) than total physical activity (counts/min) in children.
Clinical measurements and blood sampling
Clinical measurements and blood sampling were conducted from 30 August to 28 November 2011 during morning hours at each school, as previously described( Reference Damsgaard, Dalskov and Laursen 37 ). Children had been fasting since midnight, had voided and were wearing only light clothing. Height was measured to the nearest one decimal using a transportable stadiometer (CMS Weighing Equipment Ltd). Height was derived as the mean of three consecutive measurements with the child in standing position and holding his head in the Frankfurter plane and body weight was measured using a digital scale (BWB 800 S; Tanita). BMI was calculated as body weight (kg) divided by height (m2), and sex-adjusted and age-adjusted Z-scores for BMI were calculated using the WHO AnthroPlus software( 38 ). Children were categorised as underweight, normal weight, overweight and obese on the basis of age- and sex-specific cut-offs defined to pass through BMI of 18·5, 25 and 30 kg/m2 at the age of 18 years, as described by Cole et al. ( Reference Cole, Flegal and Nicholls 39 , Reference Cole, Bellizzi and Flegal 40 ). Waist circumference was measured in centimetres to the nearest millimetre using a non-elastic measuring tape at the level of the umbilicus, and the mean of three consecutive measurements was used. Total body fat mass was determined by dual-energy X-ray absorptiometry (DXA) scanning (Lunar Prodigy ProTM; GM Healthcare) with EnCoreTM software. FMI was calculated as total fat mass divided by height squared. In addition, the investigator recorded the apparent ethnicity of the child by applying the terminology of the DXA software – that is, Caucasian, Asian, African, Latin or Other. Children from Turkey, the Middle East, Pakistan and India were recorded as Caucasians. Because of small categories, a dichotomised variable indicating whether or not the child was Caucasian (yes/no) was used.
Blood pressure and heart rate were measured by an automated device (UA-787 Plusl; A&D Medical) after 10 min of rest using two different cuff sizes (18–22 or 22–32 cm). A second device (ProBP 3400 Sure BP; Welch Allyn Inc.) was used for children with arm circumferences <18 cm. Measurements were performed three times whereof the mean of the last two measurements was used. Thereafter, fasting blood was sampled from the forearm by venepuncture. Local anaesthetic patches were offered to the children to reduce discomfort of blood sampling.
Blood analyses
Whole-blood glucose concentrations were analysed immediately after sampling (Glucose 201; Hemocue Danmark). Twenty-four glucose samples were removed from the analyses because they were obtained from non-fasting children. Heparinised blood and blood with EDTA were centrifuged at 2500 g for 10 min at room temperature, and plasma was stored at −80°C for batch analysis of total, LDL and HDL-cholesterol, TAG, IL-6 and adiponectin. Blood collected in serum tubes with gel was centrifuged after 30 min of coagulation at room temperature, and it was stored at −80°C for later batch analysis of insulin, 25(OH)D and intact PTH. For each analysis, all samples were ran on the same device with the same reagent lot, all samples from each child were analysed on the same day and all samples from one school were analysed in one assay. Cholesterols and TAG were quantified on the Vitros 5·1 FS analyser (Ortho-Clinical Diagnostics, Johnson & Johnson). LDL-cholesterol was calculated using Friedewalds’s equation( Reference Friedewald, Levy and Fredrickson 41 ). Plasma high-sensitivity IL-6 and adiponectin were measured in duplicate by ELISA (R&D Systems Europe Ltd), and serum insulin was quantified on ADVIA Centaur XP (Siemens Healthcare). Homoeostasis model of assessment of insulin resistance (HOMA-IR) was calculated as plasma glucose (mmol/l)×serum insulin (mIU/l)/22·5( Reference Cutfield, Jefferies and Jackson 42 ), with insulin concentrations converted from pmol/l to mIU/l by dividing by 6·945. Twenty-three insulin and HOMA-IR samples were removed from the analyses because they were obtained from non-fasting children.
Vitamin D status was measured as serum 25(OH)D concentrations (including both D2 and D3) by an automated chemiluminescent immunoassay on DiaSorin LIAISON (DiaSorin AB) at the Department of Clinical Biochemistry, Aalborg University Hospital, Aalborg, Denmark, a laboratory partaking in the vitamin D external quality assessment scheme (DEQAS)( Reference Carter, Berry and Gunter 43 ). During the serum 25(OH)D analysis period (May to September 2012), the DEQAS quality control showed that our analysis had a negative bias of up to 30·6 % compared with the all laboratory trimmed means (ALTM) and a bias of 2·3–14·5 % compared with the means of laboratories using the same method as we used (method mean). Accordingly, the results of the laboratory are always within 2 sd but mostly within 1 sd of ALTM with a tendency to be slightly lower. Before analysis, serum was thawed at room temperature for 30 min before being centrifuged at 4000 rpm for 3 min. Concentrations are presented in nmol/l (1 nmol/l=0·4006 ng/ml), and lowest detection limit was 10 nmol/l. Vitamin D deficiency and insufficiency was defined as serum 25(OH)D concentrations <25 and ≤50 nmol/l, as recommended by the European Society for Paediatric Gastroenterology, Hepatology and Nutrition( Reference Braegger, Campoy and Colomb 44 ).
Serum intact PTH concentrations were determined by a chemiluminescent immunoassay on ADVIA Centaur XP (Siemens Healthcare). Lowest detection limit was 0·265 pmol/l. One sample had PTH concentration below the detection limit of 0·265 pmol/l; this sample was defined as half of the detection limit (0·133 pmol/l), and one doubtful PTH value of 109 pmol/l (25(OH)D at 89·1 nmol/l) was removed from the data set as extreme outlier.
EPA+DHA in whole blood, a biomarker of fish and n-3 long-chain PUFA intake, was measured by high-throughput GC within 3 months after blood sampling, as previously described( Reference Damsgaard, Eidner and Stark 45 ). The content of individual fatty acids and fatty acid classes are given in weight% of the total whole-blood fatty acids.
The inter- and intra-assay CV for the blood analyses were as follows: 1·4 and 1·2 % (total cholesterol); 2·0 and 1·2 % (HDL-cholesterol); 1·5 and 0·8 % (TAG); 6·7 and 2·9 % (IL-6); 11 and 3·8 % (adiponectin); 2·5 and 3·1 % (insulin); 5·4 and 7·6 % (25(OH)D); 7·4 and 7·9 % (PTH); 4·5 and 1·3 % (EPA); and 6·4 and 2·4 % (DHA). The inter-assay variation for glucose was 4·0 %.
Metabolic syndrome score and clinically high values
A continuous MetS score was calculated by combining the Z-scores derived from separate analyses of the five outcomes: mean arterial pressure, plasma HDL-cholesterol, plasma TAG, HOMA-IR and waist circumference. This was based on the International Diabetes Federation (IDF) definition of the MetS for children ≥10 years of age( Reference Zimmet, Alberti and Kaufman 13 ), although we included HOMA-IR rather than blood glucose because the latter is highly stable in non-diabetic children, even among obese adolescents with MetS features( Reference Gobel, Jensen and Frokiaer 46 ). In addition, the cut-offs recommended by the IDF for diagnosing the MetS in ≥10-year-olds were used in the present study to identify clinically aberrant values of the individual MetS markers( Reference Zimmet, Alberti and Kaufman 13 ). These were ≥130 and ≥85 for systolic and diastolic blood pressure, respectively; ≥1·7 and <1·03 mmol/l for TAG and HDL-cholesterol, respectively; and ≥90th percentile for waist circumference, using a recently published Danish reference material for waist circumference in children( Reference Wohlfahrt-Veje, Tinggaard and Winther 47 ). No widely used cut-offs for HOMA-IR have been defined in children. The cut-off of 2·5 used in adults has been applied, but as it has been argued that it should be closer to 3 in growing children of this age( Reference Tresaco, Bueno and Pineda 48 ) the cut-off for HOMA-IR was set at >2·8 in the present study. For total and LDL-cholesterol, values ≥200 mg/dl (≥5·2 mmol/l) and ≥130 mg/dl (≥3·4 mmol/l) were defined as elevated, as recommended by the American Academy of Pediatrics( 49 ).
Statistical analyses
The statistical analyses were carried out using STATA (version 12.1; StataCorp). Assumptions underlying statistical models were assessed graphically using residual and normal probability plots, and outcomes were logarithm-transformed as appropriate. Significance was established at P<0·05. Descriptive characteristics of the study sample are presented as means and standard deviations, medians (25th–75th percentile) or proportions (%), and sex differences in continuous variables were tested using the two-sample t test or Wilcoxon–Mann–Whitney test (non-normally distributed variables), and with the χ 2 test or Fisher’s exact test for categorical variables.
Linear mixed-effects models were used to evaluate associations between serum 25(OH)D and the cardiometabolic markers, and in all models school, grade, class and sibling were included as random effects to account for the dependency caused by the nested design. Sex, age, height, ethnicity, entered puberty (yes/no) and parental education were included as fixed effects, as well as EPA+DHA in whole blood, which has previously been associated with cardiometabolic health in the current study population( Reference Damsgaard, Eidner and Stark 45 ). In addition, an interaction term between sex and puberty was included in the models, but it was removed if nonsignificant. In addition, all associations including blood pressure were adjusted for the blood pressure device applied.
Additional adjustment for FMI (or BMI-for-age Z-scores), MVPA, as well as PTH, as potential confounders or mediators were performed subsequently in those models that had initially shown significant associations between 25(OH)D and cardiometabolic markers, by including one covariate at a time. High colinearity meant that waist circumference and the MetS score were not adjusted for FMI or BMI Z-scores. All estimated regression coefficients are presented as outcome response per 10 nmol/l increase in serum 25(OH)D.
Finally, we explored whether serum 25(OH)D was associated with elevated cardiometabolic values, using logistic regression analysis. These models included the same fixed effects as the linear regression models described above. In case of convergence problems, reduced logistic regression models were fitted including only class as random effect or no random effects.
Results
Characteristics of the study population
Of the 834 children originally enroled in the OPUS School Meal Study, eleven children withdrew before the first blood sampling, seven were ill or on holiday on the day of sampling and thirty-four children failed to deliver a blood sample or had a sample with insufficient blood volume for 25(OH)D analysis. Accordingly, 782 children (93·8 %) were included in the present study. There were 47·7 girls and 52·3 % boys and most children (94·7 %) were of Caucasian ethnicity, whereas 2·6, 1·8, 0·8 and 0·1 % were of Asian, Afro-American, Latin-American and other ethnicities, respectively. As will be published elsewhere (Rikke A. Petersen, unpublished results), the serum 25(OH)D concentrations ranged from 15 to 132 nmol/l and concentrations ≤50 nmol/l were observed in 28.4 % of the children, whereas 2·4 % had concentrations <25 nmol/l. About half of the children had taken vitamin D-containing supplements during the dietary registration week. Boys were older, spent more time on MVPA, had higher intakes of energy, protein, vitamin D and Ca and higher serum 25(OH)D than girls, whereas more girls had entered puberty (Table 1). In addition, girls had higher FMI and higher values of most cardiometabolic markers compared with boys (Table 2).
Table 1 Characteristics of the study population (Mean values and standard deviations; median values and 25th, 75th percentiles; percentages)
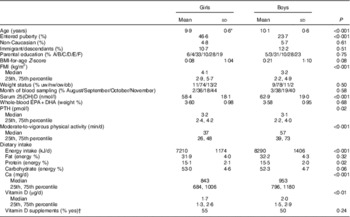
25(OH)D, 25-hydroxy vitamin D; A, ≤lower secondary education; B, upper secondary education; C, vocational education; D, short higher education; E, bachelor’s degree or equivalent; F, ≥master’s degree; nw, normal weight; ob, obese; ow, overweight; PTH, parathyroid hormone; uw, underweight.
* n 756–782; however n 727 for PTH and n 681 for dietary intake.
† Registered intake of vitamin D-containing dietary supplements ≥1 d during the dietary recording week.
Table 2 Cardiometabolic risk markers in the study population (Mean values and standard deviations; median values and 25th, 75th percentiles)
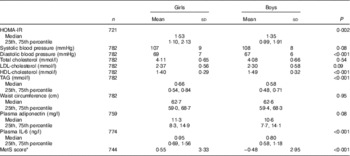
HOMA-IR, homoeostasis model of assessment-insulin resistance; MetS: metabolic syndrome.
* Based on mean arterial pressure, HDL-cholesterol, TAG, HOMA-IR and waist circumference (see the ‘Methods’ section).
Associations between vitamin D status and BMI, fat mass index, physical activity and parathyroid hormone
Serum 25(OH)D was negatively associated with BMI Z-scores (P=0·03) and FMI (P=0·001) and positively associated with MVPA (1·21 min/d, 95 % CI 0·29, 2·12/10 nmol/l increase) (P=0·010). However, the association with BMI Z-scores became nonsignificant when the model was adjusted for parental education (−0·03, 95 % CI −0·07, −0·001, P=0·14). In contrast, the negative association between serum 25(OH)D and FMI (−0·09 kg/m2, 95 % CI −0·16, −0·02, P=0·007), as well as the positive association with MVPA (1·29 min/d (95 % CI 0·36, 2·23, P=0·007), remained after adjustment for parental education. Moreover, serum 25(OH)D was negatively associated with PTH, corresponding to 0·23 pmol/l (95 % CI 0·16, 0·29, P<0·001) lower PTH per 10 nmol/l increase in 25(OH)D.
Associations between vitamin D status and cardiometabolic risk markers
Serum 25(OH)D was negatively associated with diastolic blood pressure, total and LDL-cholesterol, TAG and the MetS score, but not with HOMA-IR, systolic blood pressure, HDL-cholesterol or waist circumference (Table 3). Adjustment for FMI did not change any of the associations, whereas the association between serum 25(OH)D and diastolic blood pressure became borderline significant after adjustment for MVPA. Adjustment for BMI Z-scores instead of FMI or for PTH did not change the results (data not shown).
Table 3 Associations between serum 25-hydroxy vitamin D (25(OH)D) and cardiometabolic risk markers (β Coefficients and 95 % confidence intervals)
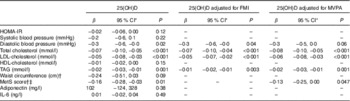
FMI, fat mass index; HOMA-IR, homoeostasis model of assessment-insulin resistance; MetS, metabolic syndrome; MVPA, moderate-to-vigorous physical activity (min/d).
* Mixed linear models, all adjusted for sex, age, height, ethnicity, whole-blood EPA+DHA, entered puberty (yes/no) and parental education. Regression coefficients are presented as cardiometabolic outcome response per 10 nmol/l increase in serum 25(OH)D.
† Because of high risk of colinearity, waist circumference and the MetS score were not adjusted for FMI.
‡ Based on mean arterial pressure, plasma HDL-cholesterol, plasma TAG, HOMA-IR and waist circumference.
Only fifteen children (1·9 %) had plasma TAG ≥1·7 mmol/l. The same number had high blood pressure defined as ≥130/85 mmHg, whereas thirty-seven children (4·7 %) had HDL-cholesterol values <1·03 mmol/l. A total of sixty-five children (9·0 %) had HOMA-IR >2·8 and 265 children (33·9 %) had a waist circumference ≥90th percentile( Reference Wohlfahrt-Veje, Tinggaard and Winther 47 ). Elevated total and LDL-cholesterol (≥5·2 and ≥3·4 mmol/l, respectively) were found in fifty (6·4 %) and thirty-nine (5·0 %) children, respectively. Higher serum 25(OH)D was associated with reduced odds for high total cholesterol – that is, OR 0·80 (95 % CI 0·66, 0·97) (P=0·03) per 10 nmol/l increase in serum 25(OH)D. This was not seen for LDL-cholesterol (P=0·93) or any of the other cardiometabolic markers (P>0·12).
Discussion
This study showed inverse associations between serum vitamin D status and a number of cardiometabolic risk markers in a sample of primarily normal weight, healthy children with low prevalence of vitamin D deficiency. To our surprise, the associations with plasma cholesterols, TAG and the continuous MetS score were independent of the potential confounding effects of fat mass and physical activity. The negative association with the MetS score was likely driven by TAG and to some degree by waist circumference, as total and LDL-cholesterol were not included in the score.
Some previous studies among child populations of fairly comparable ethnicity, age and body size have also demonstrated inverse associations between vitamin D status and total cholesterol( Reference Kumar, Muntner and Kaskel 20 , Reference Delvin, Lambert and Levy 50 ), TAG( Reference Delvin, Lambert and Levy 50 , Reference Rodriguez-Rodriguez, Ortega and Gonzalez-Rodriguez 51 ), diastolic blood pressure( Reference Kumar, Muntner and Kaskel 20 , Reference Williams, Fraser and Sayers 52 ) and waist circumference( Reference Pacifico, Anania and Osborn 16 ), whereas four studies in Canadian, Spanish, American and British children, respectively, found no associations with LDL-cholesterol( Reference Sacheck, Goodman and Chui 24 , Reference Delvin, Lambert and Levy 50 – Reference Williams, Fraser and Sayers 52 ). A study in 149 Spanish children reported an inverse association with TAG, but not with total cholesterol, after adjustment for age, sex, BMI and physical activity( Reference Rodriguez-Rodriguez, Ortega and Gonzalez-Rodriguez 51 ). Whereas associations with HDL-cholesterol were not found in the present study, positive associations between serum 25(OH)D and HDL-cholesterol were reported in large samples of American( Reference Kumar, Muntner and Kaskel 20 ) and British children( Reference Williams, Fraser and Sayers 52 ). In the British study, the association with HDL-cholesterol, but not that with blood pressure, remained after adjustment for waist circumference, socioeconomic position and other potential confounders( Reference Williams, Fraser and Sayers 52 ). None of the studies investigating associations with plasma lipid profile adjusted for puberty, which may be an important confounder.
In the present study, vitamin D status was positively associated with BMI and FMI. However, only the association with FMI remained after adjustment for parental education. This indicates that socioeconomic differences may indeed be confounding the association with BMI, probably through an effect on behaviours related to both vitamin D exposure and being overweight. However, in line with the persisting association with FMI in the present study, a randomised controlled trial in African-American children reported that the serum 25(OH)D response to vitamin D supplementation was consistently inversely correlated with baseline total body fat mass( Reference Dong, Stallmann-Jorgensen and Pollock 53 ). Similarly, an American vitamin D supplementation trial in Caucasian adolescents found increments in 25(OH)D concentrations to be lower in obese compared with non-obese adolescents( Reference Aguirre Castaneda, Nader and Weaver 54 ), indicating that vitamin D sequestering in adipose tissue may indeed have a role.
We showed that associations between vitamin D status and blood pressure and plasma lipids were independent of fat mass and BMI, indicating potential independent mechanisms. It has been demonstrated that the active metabolite of vitamin D, 1,25(OH)2D, can upregulate lipoprotein lipase in vitro, thereby potentially playing a beneficial role in lipoprotein metabolism( Reference Querfeld, Hoffmann and Klaus 55 ). However, causality cannot be inferred from the present study, and to our knowledge no randomised controlled trials have investigated the effect of vitamin D supplementation on plasma lipids in children. A non-randomised study supplementing seventy Argentine Indian school children with 5000 IU (125 µg/d) vitamin D for 8 weeks showed that HDL-cholesterol measured after 1 year was increased compared with a group of twenty unsupplemented children( Reference Hirschler, Maccallini and Sanchez 56 ). In contrast with the present study, the study population had high prevalence of vitamin D deficiency – that is, 20 % of the children had serum 25(OH)D <25 nmol/l. With regard to blood pressure, there is some evidence, especially from vitamin D receptor knockout mice, that vitamin D can reduce blood pressure through inhibition of the renin–angiotensin system( Reference Li 6 ). Other suggested mechanisms include regulation of vascular tone by 1,25(OH)D by facilitating Ca influx to the muscle cells and direct effects on vascular endothelium and smooth muscle cells( Reference Vaidya and Forman 57 ) – for example, through regulation of transcription of endothelial nitric oxide synthase, which has been demonstrated in vitro( Reference Talmor, Golan and Benchetrit 58 ). However, the association between vitamin D status and blood pressure was somewhat confounded by physical activity in the present study. This indicates that behaviours related to both vitamin D exposure and blood pressure rather than biological mechanisms may be responsible for the association. To our knowledge, only the single-blinded randomised controlled trial in forty-nine African-American adolescents has investigated the effects of vitamin D on blood pressure. The participants, who had a mean serum 25(OH)D of 33·4 nmol/l at baseline, were randomised to 2000 IU/d (50 µg/d) or 400 IU/d (10 µg/d) (control) vitamin D for 16 weeks from February to May. Although carotid-femoral pulse-wave velocity increased in the control group and decreased in the high-dose vitamin D group, no changes or differences were seen in systolic or diastolic blood pressure( Reference Dong, Stallmann-Jorgensen and Pollock 53 ). Meta-analyses of randomised trials in adults show no or little effect of vitamin D on blood pressure. Witham et al. ( Reference Witham, Nadir and Struthers 59 ) included eleven randomised controlled vitamin D trials of mainly older subjects and found a small reduction in diastolic blood pressure (−3·1 mmHg, 95 % CI −5·5, −0·6) among the eight studies in hypertensive subjects only. However, as most studies were relatively small (less than sixty subjects) and significant heterogeneity was present for the subgroup analyses, the reliability of these findings is unclear. This area calls for further investigation, especially in children.
MVPA was also positively associated with vitamin D status in the present study, something that has previously been observed in Korean school children of similar age( Reference Ha, Cho and Lee 27 ). In the present study, this level of physical activity could be hypothesised to be a proxy for sun exposure because of the outdoor nature of much MVPA during the period from August to November where data were collected. Indeed, positive associations have been observed between outdoor engagement and physical activity in the OPUS School Meal Study( Reference Hjorth, Chaput and Michaelsen 35 ) and among other children of this age( Reference Nielsen, Taylor and Williams 60 , Reference Nilsson, Andersen and Ommundsen 61 ). Physical activity could also be a proxy for a particular lifestyle and an eating pattern with higher intake of fish (the main food source of vitamin D in Danes), as has been demonstrated in previous studies among children( Reference Morin, Turcotte and Perreault 62 , Reference Ottevaere, Huybrechts and Beghin 63 ). In addition, we have recently shown that n-3 long-chain PUFA in blood, a biomarker of fish intake, was cross-sectionally associated with cardiometabolic risk markers in the OPUS School Meal Study( Reference Damsgaard, Eidner and Stark 45 ). However, the presented results were adjusted for this biomarker. Finally, although we found a strong inverse association between PTH and vitamin D status in the present study, the observed associations between vitamin D status and the cardiometabolic markers did not appear to be mediated by PTH.
To our knowledge, this is the first study of its kind among children from the Nordic countries. Vitamin D status was measured solely during autumn and early winter months, a time of year when vitamin D status is expected to be close to annual peak in Danish children. Still, about every fourth child had serum 25(OH)D status ≤50 nmol/l. A limitation to autumn 25(OH)D measurements is that they are likely influenced by recent sun behaviour including sunny vacations abroad, which were not recorded in the study. However, a recent study in Danish girls showed that the 25(OH)D concentrations attained during summer were strong predictors of vitamin D status the following winter( Reference Andersen, Brot and Jakobsen 64 ). The explorative nature of the study requested multiple testing and the results must naturally be interpreted with this in mind. In addition, although we were able to adjust for important potential confounders such as pubertal status, ethnicity and the biomarker of fish intake, there is always a risk of residual confounding in cross-sectional studies such as the present one. However, the robust findings throughout the adjustments and the consistently low P values for the plasma lipids do not suggest random findings. It is a great strength that the study included DXA scans and 7 d accelerometry measurements, as these provided validated and objective measurements of body fat and physical activity. The observed associations were of small magnitude, and although higher serum 25(OH)D was associated with reduced odds for high total cholesterol values this was not the case for HDL-cholesterol or TAG. Therefore, the immediate clinical relevance is uncertain. However, plasma lipid levels and blood pressure have been shown to track from childhood to adulthood( Reference Juhola, Magnussen and Viikari 65 ) and children with the MetS in childhood are more likely to have the MetS as adults( Reference Morrison, Friedman and Wang 66 ). This indicates that from a population perspective slightly lower levels of the cardiometabolic risk markers may give long-term benefit, if sustained over years. In line with this, higher serum 25(OH)D seemed to be associated with more beneficial HDL-cholesterol, TAG and MetS scores over the entire range of values in the present study, rather than having a specific impact on the proportion of aberrant values. There is no uniformly used set of cut-offs for cardiometabolic risk markers in children, and the various cut-offs for aberrant values should be used and interpreted with caution. The very high proportion of children with waist circumference above the 90th percentile in the present study, despite the use of a recently published Danish reference material( Reference Wohlfahrt-Veje, Tinggaard and Winther 47 ), is hard to explain, but it may relate to the fact that we measured waist circumference at the level of the umbilicus, whereas the reference study measured parallel to the floor at the midpoint between the ribs and the top of the iliac crest.
In conclusion, vitamin D status was negatively associated with a number of cardiometabolic markers in Danish school children with low prevalence of vitamin D deficiency, and apart from blood pressure the associations were independent of body fat and physical activity. The potential underlying cause–effect relationship and possible long-term health implications should be investigated further in randomised controlled trials.
Acknowledgements
The authors thank the participating children, their families, the school managements, teachers and other staff, as well as the entire study team, for their contribution to the study.
The Optimal well-being, development and health for Danish children through a healthy New Nordic Diet (OPUS) study was supported by the Nordea Foundation (grant no: 02-2010-0389). The funder had no role in the design and analysis of the study or in the writing of this article.
R. A. P., C. M., K. F. M., A. A., I. T., L. B. S., S. M. D. and C. T. D. designed the research; R. A., M. F. H., L. B. S., S. M. D., C. T. D. and R. A. P. conducted the research; R. A. P. and C. R. analysed the data; H. K. provided acquisition of the data; R. A. P. and C. T. D. wrote the paper; and R. A. P. and C. T. D. had primary responsibility for the final content.
R. A. P., S. M. D., L. B. S., M. F. H., R. A., I. T., H. K., C. R., K. F. M., C. M. and C. T. D. declare no conflicts of interest that could be perceived as prejudicing the impartiality of the research reported. A. A. is an ad hoc consultant for clients of Gerson Lehrman Group (USA) and consultant/member of advisory/scientific boards for Global Dairy Platform (USA), Kraft Foods (USA), Dutch Beer Knowledge Institute (The Netherlands), McCain Foods Limited (USA), McDonald’s (USA) and Jenny Craig (USA) and receives honoraria as speaker and research funding from a range of Danish and international companies.