Probiotics have been defined as ‘live microorganisms that, when administered in adequate amounts, confer a health benefit to the host’( Reference Fuller 1 ). Probiotics promote crypt cell proliferation, prevent cytokine-induced apoptosis( Reference Laudanno, Vasconcelos and Catalana 2 ), reduce pro-inflammatory cytokine production and regulate the intestinal immune system( Reference Dieleman, Goerres and Arends 3 ). Saccharomyces boulardii is a probiotic thermophilic non-pathogenic yeast( Reference Jespersen 4 ) that is widely used in the treatment of gastrointestinal disorders associated with diarrhoea of varying aetiology( Reference Buts and De Keyser 5 , Reference Buts, Stilmant and Bernasconi 6 ). In a study on irinotecan-induced intestinal mucositis, Sezer et al. ( Reference Sezer, Usta and Cicin 7 ) found that the administration of S. boulardii reduced the severity of intestinal mucositis by significantly decreasing leucocyte migration and inflammation.
Mucositis is a major oncological problem associated with the cytotoxicity of chemotherapy and radiotherapy. According to Duncan & Grant( Reference Duncan and Grant 8 ), the development of intestinal mucositis may be divided into the following stages: the inflammatory stage; the epithelial degradation stage; the ulceration/bacterial stage, followed by the re-establishment of functional epithelia.
The anti-metabolite agent 5-fluorouracil (5-FU) is used in the treatment of a range of cancers, including colorectal and breast cancers( Reference Longley, Harkin and Johnston 9 , Reference Sonis 10 ), but it is known to induce intestinal damage, especially intestinal mucositis( Reference Baerg, Murphy and Anderson 11 ), and intestinal stem cell apoptosis( Reference Keefe, Gibson and Hauer-Jensen 12 ). Using an animal model, Soares et al. ( Reference Soares, Mota and Gomes 13 ) found that 5-FU-induced intestinal mucositis was associated with neutrophil infiltration, increased levels of pro-inflammatory cytokines and a significant delay in gastric emptying. However, there are no previous studies that have evaluated the effect of treatment with S. boulardii on the inflammatory and functional aspects of 5-FU-induced intestinal mucositis, which is the objective of the present study.
Materials and methods
Animals
Male Swiss mice weighing 25–30 g (supplied by the Department of Physiology and Pharmacology, UFC Medical School) were kept in a temperature-controlled room with access to water ad libitum and were fasted for 24 h before all experiments. The study was approved by a local research ethics committee (protocol #34/10), and all procedures involving animals were carried out in accordance with the Guide for the Care and Use of Laboratory Animals of the US Department of Health and Human Services.
Model of intestinal mucositis induced by 5-fluorouracil
Mice were randomly assigned to one of the following three groups comprising eight mice each: saline (control); saline+5-FU (450 mg/kg, single dose, intraperitoneally); 5-FU+S. boulardii (16 × 109 colony-forming units (CFU)/kg) (Merck) for 3 d. Mice were killed 3 d after treatment with S. boulardii. Blood samples were collected, and the jejunum and ileum were removed for analyses of morphology and histopathology and determination of apoptosis, myeloperoxidase (MPO) activity, and non-protein sulfhydryl group, nitrite and cytokine (TNF-α, IL-1β and chemokine (C–X–C motif) ligand 1 (CXCL-1)) concentrations. Diarrhoea occurrence and weight were recorded daily throughout the study in mice. Weight (g) is expressed as the percentage of weight loss compared with the control group.
Leucocyte count
Mice were anaesthetised before the collection of blood samples, which was performed by heart puncture. The total number of leucocytes was determined after dilution of blood samples in Turk's solution using a Neubauer chamber. Results are expressed as leucocytes/ml.
Intestinal morphometry, histopathology and apoptosis assay
Segments of the jejunum (3 cm segment immediately distal to the ligament of Treitz) and distal ileum (6 cm segment adjacent to the ileocaecal valve) were collected, fixed and stained with haematoxylin and eosin for the measurement of villus height and crypt depth. For each sample, ten intact and well-oriented villi and crypts were measured and averaged. Microscopy was double blinded. Mucosal inflammation was assessed using a modification of the histopathological scores described by Macpherson & Pfeiffer( Reference MacPherson and Pfeiffer 14 ) and was graded as follows: 0, normal histological findings; 1, villus blunting, loss of crypt architecture, sparse inflammatory cell infiltration, vacuolisation and oedema; 2, villus blunting with fattened and vacuolated cells, crypt necrosis, intense inflammatory cell infiltration, vacuolisation and oedema; 3, villus blunting with fattened and vacuolated cells, crypt necrosis, intense inflammatory cell infiltration, vacuolisation and oedema. Cell apoptosis in the jejunal and ileal segments was detected by terminal deoxynucleotidyl transferase-mediated dUTP nick-end labelling (TUNEL) assay using the In situ Apoptosis Detection Kit (Millipore) according to the manufacturer's instructions. Results are expressed as the presence or absence of TUNEL-positive cells.
Determination of intestinal myeloperoxidase activity
MPO is primarily found in azurophilic neutrophil granules and has been extensively used as a biochemical marker of infiltration of granulocytes into various tissues, including the gastrointestinal tract. The extent of neutrophil accumulation in the intestinal mucosa was quantified using a MPO activity assay kit( Reference Bradley, Christensen and Rothstein 15 ). Briefly, intestinal tissue (50 mg/ml) was homogenised in hexadecyltrimethylammonium bromide buffer (Sigma). The homogenate was centrifuged at 4500 rpm for 7 min at 4 °C. MPO activity in the resuspended pellet was assayed by measuring the change in absorbance at 450 nm using o-dianisidine dihydrochloride (Sigma) and 1 % H2O2 (Merck). Results are expressed as units/mg tissue. A unit of MPO was defined as the amount of enzyme required to convert 1 μmol/min H2O2 into water at 22 °C.
Determination of sulfhydryl group concentrations
The concentrations of non-protein sulfhydryl groups (mainly reduced glutathione) in intestinal tissues were assessed using an assay for non-protein sulfhydryl content( Reference Sedlak and Lindsay 16 ). Briefly, 100 mg/ml of frozen intestinal tissue were homogenised in 0·02 m-EDTA. Aliquots of 400 μl homogenate were mixed with 320 μl of distilled water and 80 μl of 50 % TCA to precipitate the proteins. The mixture was centrifuged (3000 rpm) for 15 min at 4°C. Aliquots of 400 μl of supernatant were mixed with 800 μl of 0·4 m-Tris buffer (pH 8·9) and 20 μl of 5,5-dithiobis-(2-nitrobenzoic acid) (Fluka), followed by shaking for 3 min. Within 5 min of the addition of 5,5-dithiobis-(2-nitrobenzoic acid), absorbance was read at 412 nm against a blank reagent without the homogenate. Results are expressed as μg/mg tissue.
Determination of nitrite concentrations
The production of NO was determined indirectly by measuring nitrite concentrations based on the Griess reaction( Reference Chen, Swilley and Bell 17 ). Briefly, 100 μl of intestinal tissue homogenate were incubated with 100 μl of Griess reagent (1 % sulphanilamide in 1 % H3PO4-0·1 % N-(1-naphthyl)ethylenediamine dihydrochloride-1 % H3PO4-distilled water, 1:1:1:1) at room temperature for 10 min. Absorbance was measured at 540 nm in a microplate reader. Nitrite concentrations were determined from a standard nitrite curve generated using NaNO2.
Determination of cytokine (TNF-α, IL-1β and chemokine (C–X–C motif) ligand 1) concentrations
The concentrations of cytokines (TNF-α, IL-1β and CXCL-1) in duodenum samples were determined by ELISA using protocols supplied by the manufacturer (R&D Systems). Results are expressed as pg/ml.
Measurement of gastric emptying and intestinal transit
Gastric emptying and intestinal transit were measured using the modified technique of Reynell & Spray( Reference Reynell and Spray 18 ). Mice were fed 300 μl of a test meal consisting of a non-absorbable marker (0·75 mg/ml phenol red in 5 % glucose). After 20 min, mice were killed by cervical dislocation. After laparotomy, the stomach and bowels were exposed and the oesophageal-gastric, gastroduodenal and ileocaecal junctions were immediately isolated by ligatures. The specimens were then moved onto a table and divided into stomach and proximal, medial and distal bowel segments. Each segment was placed in a graduated cylinder, and the total volume was measured by adding 10 ml of 0·1 m-NaOH. The samples were then cut into small pieces and homogenised for 30 s. After 20 min, 1 ml of supernatant was removed and centrifuged for 10 min at 2800 rpm. The proteins in the homogenate were precipitated by adding 20 % TCA and separated by centrifugation for 20 min at 2800 rpm. Then, 150 ml of supernatant were added to 200 ml of 0·5 m-NaOH. The absorbance of the samples was determined by spectrophotometry at a wavelength of 540 nm, and it is expressed as optical density.
Fractional dye retention is expressed as a percentage, according to the following equation:

Intestinal transit was calculated for each bowel segment by dividing the amount of phenol red recovered from a given segment by the amount of phenol red recovered from all the three segments, and it is expressed as a percentage.
Determination of intestinal absorption and permeability
After an overnight fast (6–8 h), mice were administered 2 ml of a solution containing lactulose (200 mg/ml) and mannitol (50 mg/ml) by gavage needle. After 1 h, mice were given access to food and water ad libitum. Urine samples were collected for the next 24 h in a flask with 25 μl of a solution containing chlorhexidine (40 mg/ml). Total urine volume was recorded, and two aliquots were stored at − 20°C. Each urine sample (50 μl) was mixed with 50 μl of a solution containing melibiose (3·6 mm) and diluted in 2·9 ml of twice-distilled and deionised water. After centrifugation and filtration via a Millipore membrane (0·22 μm), 50 μl were employed for sugar determination using HPLC with pulsed amperometric detection as described previously( Reference Lima, Silva and Gifoni 19 ). The urinary recovery of both lactulose and mannitol was calculated as a percentage of the dose ingested. Both these sugars are hydrophilic and have a low affinity for the monosaccharide transport system and, thus, are absorbed passively in a non-mediated fashion and excreted in the urine( Reference Pearson, Eastham and Laker 20 ). Mannitol, a monosaccharide, has a radius of 0·4 nm and is absorbed transcellularly through aqueous pores in the cell membrane, reflecting the total epithelial absorptive surface area. By contrast, lactulose, a disaccharide with a radius of 0·52 nm, is absorbed paracellularly via extrusion zones at villus tips and tight junctions, reflecting the disruption of intestinal barrier function( Reference Deitch 21 ).
Statistical analysis
Results are reported as means with their standard errors for each group. Data were submitted to ANOVA followed by Bonferroni's test (parametric data) or the Kruskal–Wallis test and Dunn's test (non-parametric data). The level of statistical significance was set at P< 0·05.
Results
Effects of Saccharomyces boulardii on intestinal mucositis induced by 5-fluorouracil
Initially, a dose–response analysis was carried out for S. boulardii (1·78 × 109, 5·34 × 109, 16 × 109 and 48 × 109CFU/kg). An increase in weight was observed in the control group throughout the study period. By contrast, the weight of the 5-FU group decreased considerably (9·75 (sem 0·58)%) on day 3 after 5-FU administration compared with that of the control group. Treatment with S. boulardii at doses 1·78 × 109 and 5·34 × 109CFU/kg did not reverse the weight loss in the 5-FU group; however, treatment with S. boulardii at doses 16 × 109 and 48 × 109CFU/kg significantly prevented (P< 0·05) weight loss in the 5-FU group. Thus, we chose to use a submaximal dose (16 × 109CFU/kg) for all the other experiments (Table 1). Stools suggestive of diarrhoea were observed in the ileal segments of the 5-FU group. It was possible to detect the presence of solid faeces in the saline and 5-FU+S. boulardii groups compared with the 5-FU group (Fig. 1).
Table 1 Reduction of weight loss (%) by Saccharomyces boulardii in mice with 5-fluorouracil (5-FU)-induced intestinal mucositis (Mean values with their standard errors)

CFU, colony-forming units.
* Mean value was significantly different from that of the 5-FU group (P< 0·05).
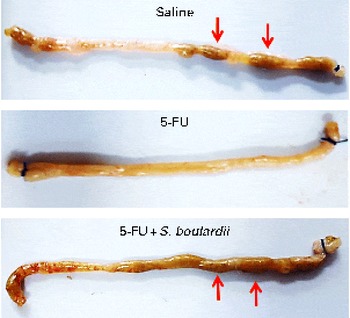
Fig. 1 Prevention of diarrhoea by Saccharomyces boulardii in mice with 5-fluorouracil-induced intestinal mucositis. Stools suggestive of diarrhoea were observed in the ileal segments of the 5-fluorouracil (5-FU) group. The image shows the presence of solid faeces (arrows) in the saline and 5-FU+S. boulardii groups compared with the 5-FU group.
Significant leucopenia was observed on day 3 after 5-FU administration (1675 (sem 138) cells/mm3) compared with the control (5289 (sem 851) cells/mm3). Treatment with S. boulardii did not prevent 5-FU-induced leucopenia (2825 (sem 303) cells/mm3).
Effects of Saccharomyces boulardii on histopathological changes in intestinal mucosa
Compared with the control group, the 5-FU group presented the following histopathological changes in the jejunum and ileum: mucosa with shortened villi with vacuolated cells; crypt necrosis; intense inflammatory cell infiltration; vacuolisation; oedema. Histopathological changes induced by 5-FU were significantly reversed in the 5-FU+S. boulardii group, as demonstrated by the microscopic scores (Table 2). The photomicrographs in Fig. 2 show intense inflammatory cell infiltration, vacuolisation and oedema in the jejunum (Fig. 2(e)) and ileum (Fig. 2(g)) of the 5-FU group compared with the control group (Fig. 2(a) and (c)). Treatment with S. boulardii for 3 d (Fig. 2(i) and (l)) reversed the changes in both segments. Furthermore, 5-FU markedly increased the apoptosis of intestinal crypt cells in the jejunum (Fig. 2(f)) and ileum (Fig. 2(h)) compared with the control (Fig. 2(b) and (d)), which was prevented by treatment with S. boulardii (Fig. 2(j) and (m)).
Table 2 Attenuation of histopathological parameters by Saccharomyces boulardii in mice with 5-fluorouracil (5-FU)-induced intestinal mucositis (Median scores and ranges)
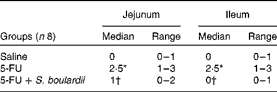
* Median value was significantly different from that of the saline group (P< 0·05).
† Median value was significantly different from that of the 5-FU group (P< 0·05).
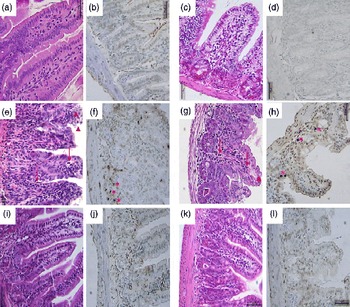
Fig. 2 Attenuation of intestinal damage and apoptosis by Saccharomyces boulardii in mice with 5-fluorouracil-induced intestinal mucositis. Photomicrographs (400 × , scale 50 μm) showing the jejunum and ileum of mice in the (a–d) control, (e–h) 5-fluorouracil (5-FU, 450 mg/kg, single dose, intraperitoneally) and (i–l) 5-FU+S. boulardii (16 × 109 colony-forming units/kg) groups treated for 3 d. (e, g) 5-FU induced shortening of the villi, deepening of the crypts, intense inflammatory cell infiltration (arrows), vacuolisation and oedema (arrowheads). Apoptosis was assessed using transferase-mediated dUTP nick-end labelling (TUNEL) assay and 5-FU was found to increase the number of TUNEL-positive cells in the (f) jejunum (*) and (h) ileum (*). Treatment with S. boulardii reverted these alterations.
5-FU induced a significant decrease in the villus height (Fig. 3(a)), an increase in the crypt depth (Fig. 3(b)) and a decrease in the villus:crypt ratio (Fig. 3(c)) in the jejunum and ileum compared the control. Figure 3(b) shows that treatment with S. boulardii reversed the 5-FU-induced increase in crypt depth in both segments. Similarly, the decrease in the villus:crypt ratio observed in the 5-FU group was significantly reversed by treatment with S. boulardii (Fig. 3(c)).
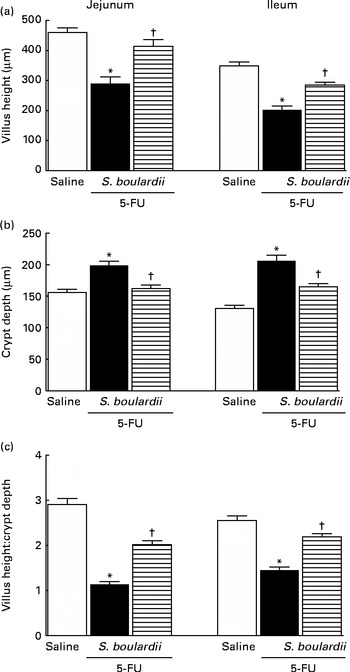
Fig. 3 Reversion of 5-fluorouracil (5-FU)-induced intestinal morphometric changes by Saccharomyces boulardii in mice. Segments of the jejunum and ileum were retrieved to measure (a) villus height, (b) crypt depth and (c) the villus:crypt ratio. Values are means, with their standard errors represented by vertical bars. * Mean value was significantly different from that of the saline group (P< 0·05; ANOVA followed by Bonferroni's test). † Mean value was significantly different from that of the 5-FU group (P< 0·05; ANOVA followed by Bonferroni's test).
Effects of Saccharomyces boulardii on myeloperoxidase activity and on reduced glutathione and nitrite concentrations
Following the administration of 5-FU, we observed a significant increase in neutrophil infiltration in the jejunum (5·87 (sem 1·06) ultrastructural MPO (UMPO)/mg) and ileum (14·09 (sem 2·99) UMPO/mg) compared with the control (jejunum 1·73 (sem 0·36) UMPO/mg and ileum 1·51 (sem 0·17) UMPO/mg). Treatment with S. boulardii reduced neutrophil infiltration in the jejunum (3·08 (sem 0·46) UMPO/mg) and ileum (3·67 (sem 0·85) UMPO/mg) (Fig. 4(a)).
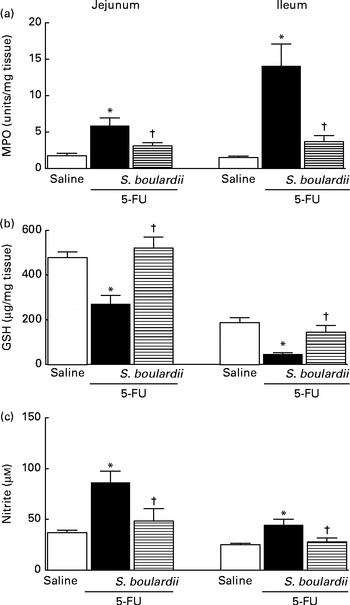
Fig. 4 Attenuation of inflammatory parameters (myeloperoxidase (MPO), reduced glutathione (GSH) and nitrite) by Saccharomyces boulardii in mice with 5-fluorouracil (5-FU)-induced intestinal mucositis. There were increased levels of (a) MPO activity, (b) GSH consumption and (c) nitrite due to exposure to 5-FU. (a–c) Treatment with S. boulardii for 3 d attenuated the inflammatory parameters. Values are means, with their standard errors represented by vertical bars. * Mean value was significantly different from that of the saline group (P< 0·05; ANOVA followed by Bonferroni's test). † Mean value was significantly different from that of the 5-FU group (P< 0·05; ANOVA followed by Bonferroni's test).
In 5-FU-treated mice, we detected significantly reduced glutathione concentrations in the jejunum (270·90 (sem 38·50) μg/mg) and ileum (46·01 (sem 6·30) μg/mg) compared with those in the control mice (jejunum 477·60 (sem 25·20) μg/mg and ileum 186·80 (sem 22·80) μg/mg), an effect that was reversed after treatment with S. boulardii by 52·43 % (521·30 (sem 48·53) μg/g) in the jejunum and by 52·94 % (144·90 (sem 29·83) μg/g) in the ileum (Fig. 4(b)).
Figure 4(c) shows that 5-FU increased nitrite concentrations in the jejunum (86·43 (sem 10·93) μm) and ileum (44·66 (sem 5·46) μm) compared with the control (37·00 (sem 2·39) and 25·08 (sem 1·38) μm, respectively). By contrast, treatment with S. boulardii reduced nitrite concentrations in the jejunum and ileum (40·28 (sem 9·85) and 32·26 (sem 5·23) μm, respectively).
Effects of Saccharomyces boulardii on TNF-α, IL-1β and chemokine (C–X–C motif) ligand 1 production
As shown in Fig. 5(a) and (b), in mice with intestinal mucositis induced by 5-FU, the concentrations of TNF-α and IL-1β increased significantly (P< 0·05) in the jejunum (56·34 and 81·73 %, respectively) and ileum (195·44 and 107·45 %, respectively) compared with those in the control mice. Similarly, CXCL-1 concentrations were increased by 5-fold in the jejunum and 3-fold in the ileum. By contrast, treatment with S. boulardii significantly (P< 0·05) reduced TNF-α and IL-1β concentrations by 48·92 and 32·21 % in the jejunum and by 38·92 and 61·79 % in the ileum, respectively. Treatment with S. boulardii decreased CXCL-1 concentrations by 5-fold in the jejunum (198·9 (sem 151·5) pg/ml) and by 3-fold in the ileum (376·6 (sem 107·1) pg/ml) (Fig. 5(c)).
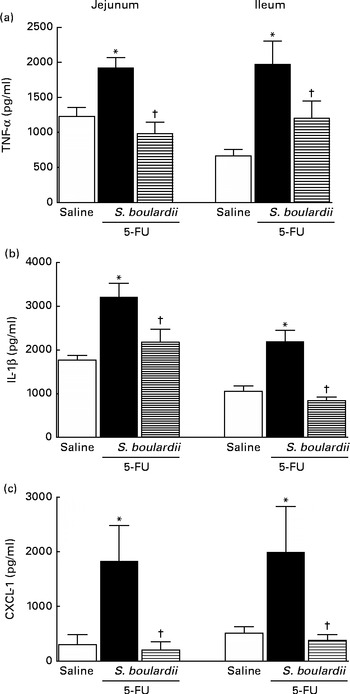
Fig. 5 Reduction of the increased concentrations of TNF-α, IL-1β and chemokine (C–X–C motif) ligand 1 (CXCL-1) by Saccharomyces boulardii in mice with 5-fluorouracil (5-FU)-induced intestinal mucositis. There were increased concentrations of (a) TNF-α, (b) IL-1β and (c) CXCL-1 due to exposure to 5-FU. (a, b and c) Treatment with S. boulardii for 3 d reduced the concentrations of pro-inflammatory cytokines. Values are means, with their standard errors represented by vertical bars. * Mean value was significantly different from that of the saline group (P< 0·05; ANOVA followed by Bonferroni's test). † Mean value was significantly different from that of the 5-FU group (P< 0·05; ANOVA followed by Bonferroni's test).
Effects of Saccharomyces boulardii on gastric emptying and gastrointestinal transit
Gastric retention was significantly (P< 0·05) higher in mice with intestinal mucositis induced by 5-FU (54·91 (sem 3·42) %) than in the control group (25·21 (sem 2·55) %) (Fig. 6(a)). This change was reversed by treatment with S. boulardii (31·38 (sem 2·79) %). Fig. 6(b) shows a significant level of retention in the proximal, medial and distal bowel segments (5-FU v. saline) associated with diarrhoea. Treatment with S. boulardii reversed these changes. We observed that S. boulardii alone had no effect on gastric emptying, but had a mild effect on medial bowel segments. However, the intestinal transit curve of S. boulardii alone had a course similar to that of the intestinal transit curve of saline.
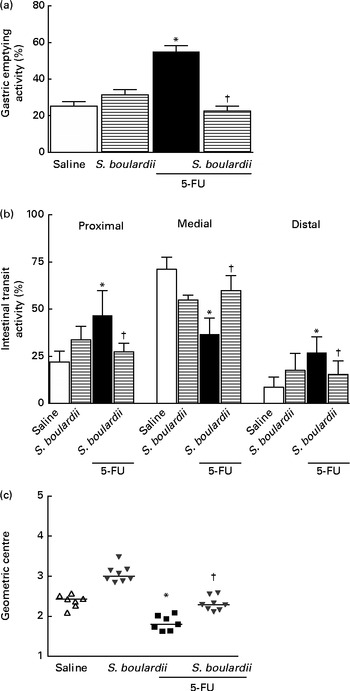
Fig. 6 Reversion of delayed gastric emptying and gastrointestinal transit associated with 5-fluorouracil (5-FU)-induced intestinal mucositis by Saccharomyces boulardii in mice. Significantly (a) delayed gastric emptying and (b) intestinal transit due to exposure to 5-FU and (c) displacement of the geometric centre were observed. (a–c) Treatment with S. boulardii for 3 d attenuated gastrointestinal disorders. Values are means, with their standard errors represented by vertical bars. * Mean value was significantly different from that of the saline group (P< 0·05; ANOVA followed by Bonferroni's test). † Mean value was significantly different from that of the 5-FU group (P< 0·05; ANOVA followed by Bonferroni's test).
Similarly, gastrointestinal transit was significantly slower in the 5-FU group than in the saline group (median geometric centre: 1·83 (sem 0·07) v. 2·32 (sem 0·08); P< 0·05), but was normalised by treatment with S. boulardii (2·31 (sem 0·06)) (Fig. 6(c)).
Effects of Saccharomyces boulardii on intestinal absorption and permeability
In mice treated with 5-FU, the excretion of mannitol was reduced to 4·96 (sem 1·24) % compared with that in the saline-treated mice (9·54 (sem 2·10) %; Fig. 7(a)). This reduction in mannitol absorption following 5-FU administration reflects a substantial decrease in the mucosal absorptive area. Mice treated with 5-FU exhibited an increase in lactulose excretion (6·60 (sem 2·05) %), but this increased excretion was not significantly different from excretion in the saline-treated group (3·65 (sem 0·95) %) (Fig. 7(b)). Additionally, the lactulose:mannitol ratio was significantly (P< 0·05) increased in the 5-FU-treated group than in the control group (1·38 (sem 0·24) v. 0·52 (sem 0·03)), but was normalised by treatment with S. boulardii (0·62 (sem 0·03)) (Fig. 7(c)).
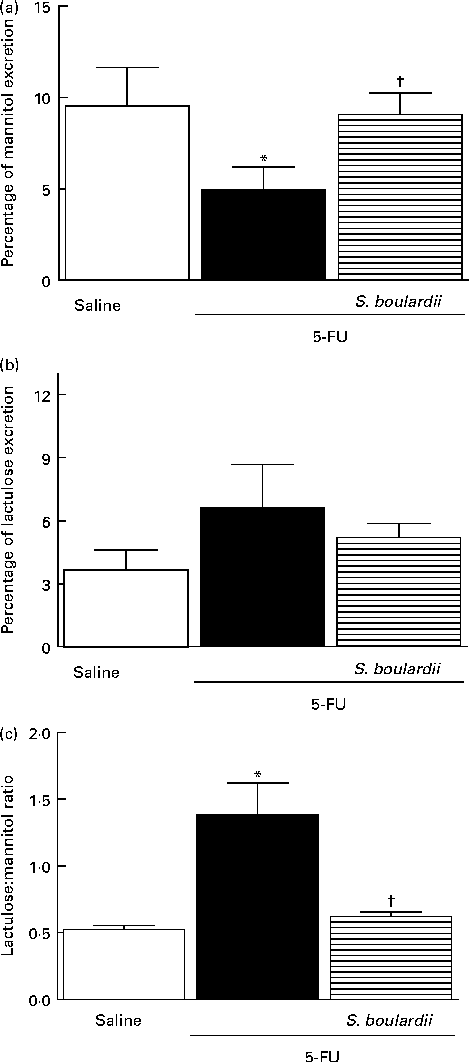
Fig. 7 Induction of the recovery of the integrity of the intestinal barrier in mice treated with 5-fluorouracil (5-FU) by Saccharomyces boulardii. Intestinal absorption and permeability were evaluated by lactulose/mannitol test. After treatment with 5-FU (450 mg/kg, single dose, intraperitoneally) or 5-FU+S. boulardii for 3 d, mice were administered a solution containing lactulose (200 mg/ml) and mannitol (50 mg/ml). (a) There was a significant reduction in the percentage of mannitol excretion induced by 5-FU treatment. (b) 5-FU induced changes, but not significantly, in the intestinal lactulose urinary excretion. (c) There was a marked increase in the lactulose:mannitol ratio induced by 5-FU. (a)–(c) Treatment with S. boulardii for 3 d reversed permeability alterations. Values are means, with their standard errors represented by vertical bars. * Mean value was significantly different from that of the saline group (P< 0·05; ANOVA followed by Bonferroni's test). † Mean value was significantly different from that of the 5-FU group (P< 0·05; ANOVA followed by Bonferroni's test).
Discussion
In the present study, treatment with S. boulardii was able to prevent diarrhoea and weight loss, which are important symptoms of intestinal mucositis induced by 5-FU. In addition, treatment with S. boulardii significantly decreased apoptosis and reversed histopathological changes in murine intestinal mucositis induced by 5-FU, partly due to reductions in inflammatory parameters (concentrations of nitrite, reduced glutathione and cytokines and infiltration of neutrophils). Interestingly, S. boulardii also reversed 5-FU-induced gastrointestinal function alterations (gastric emptying, gastrointestinal transit, and intestinal absorption and permeability). Our findings are in part consistent with those of Sezer et al. ( Reference Sezer, Usta and Cicin 7 ), who observed significantly lower levels of leucocyte migration and inflammation in a model of irinotecan-induced intestinal mucositis treated with S. boulardii. Nevertheless, this study did not investigate the role of S. boulardii in the altered function of the gastrointestinal tract in mucositic intestines.
Epithelial damage and neutrophil infiltration in the mucosa during the inflammatory stage of mucositis have been observed in several other studies( Reference Sonis, Elting and Keefe 22 ). Mucositis induced by anti-neoplastic drugs destroys epithelial cells, subsequently inducing a local inflammatory response( Reference Sonis, Elting and Keefe 22 , Reference Rubenstein, Peterson and Schubert 23 ). Using an ex vivo model, Edens et al. ( Reference Edens, Levi and Jaye 24 ) found that the migration of neutrophils to epithelial cells can induce changes in the permeability of the intestinal epithelium, possibly explaining the finding of dyspepsia in animals with 5-FU-induced intestinal mucositis.
In a hamster model of oral mucositis induced by 5-FU, Lima et al. ( Reference Lima, Brito and Cunha 25 ) observed important macroscopic and microscopic lesions and increased MPO activity, which could be inhibited by treatment with pentoxifylline and thalidomide. Another study using methotrexate revealed a significant increase in villous atrophy in rat intestinal mucosa after treatment( Reference Carneiro-Filho, Oria and Wood Rea 26 ). Intestinal injury may include brush-border hydrolase activity changes, blunted villus height, crypt deepening and increased crypt cell apoptosis with decreased proliferation( Reference Petschow, Carter and Hutton 27 , Reference Orazi, Du and Yang 28 ).
Prisciandaro et al. ( Reference Prisciandaro, Geier and Butler 29 ) reported that probiotic supernatants derived from Escherichia coli Nissle 1917 and Lactobacillus fermentum BR11 lowered the histological scores and MPO activity levels in the jejunum of rats treated with 5-FU. Smith et al. ( Reference Smith, Geier and Yazbeck 30 ) successfully reversed inflammation (as demonstrated by a decrease in MPO activity) using Lactobacillus fermentum BR11, thereby reducing the severity of 5-FU-induced intestinal mucositis in the rat jejunum. Moreover, treatment with S. boulardii has been shown to reverse increased serum nitrate and nitrite concentrations in models of gastrointestinal damage induced by non-steroidal anti-inflammatory drugs( Reference Girard, Coppe and Pansart 31 ).
In a study of colitis in mice induced by Citrobacter rodentium, Wu et al. ( Reference Wu, Vallance and Boyer 32 ) demonstrated that treatment with S. boulardii significantly attenuated weight loss, lowered intestinal crypt hyperplasia and histological damage scores, and reduced MPO activity. Canonici et al. ( Reference Canonici, Siret and Pellegrino 33 ) observed that S. boulardii secretes substances capable of increasing enterocyte migration, regardless of cell proliferation. This increase in migration was associated with the ability of S. boulardii to promote cellular–extracellular matrix interactions.
In the present study, S. boulardii significantly reduced the concentrations of pro-inflammatory cytokines (IL-1β and CXCL-8) in the mouse jejunal and ileal segments that were increased by the administration of 5-FU, in correlation with previous findings regarding gastric inflammation. Melo et al. ( Reference Melo, Brito and Soares 34 ) found TNF-α, IL-1β and CXCL-1 to be important mediators in the pathogenesis of intestinal mucositis induced by irinotecan and concluded that treatment with pentoxifylline and thalidomide had a protective effect on intestinal structures. More recently, Soares et al. ( Reference Soares, Lima-Junior and Mota 35 ) evaluated the role of platelet-activating factor (PAF) in the pathogenesis of intestinal mucositis induced by 5-FU using PAF receptor knockout mice and a PAF receptor antagonist (BN52021). The intestinal mucosa of mice treated with PAF receptor antagonist was shown to be protected against damage caused by exposure to 5-FU.
Fidan et al. ( Reference Fidan, Kalkanci and Yesilyurt 36 ) concluded that the administration of S. boulardii may have a protective effect against diarrhoeal pathogens by reducing the pro-inflammatory response. The authors observed decreased secretion of pro-inflammatory cytokines (IL-1β) in animals treated with S. boulardii, but higher concentrations of anti-inflammatory cytokines (IL-4 and IL-10), suggesting that S. boulardii has a protective effect by reducing the pro-inflammatory response. In addition, Lee et al. ( Reference Lee, Kim and Chi 37 ) observed that treatment of HT-29 cells with TNF-α and IL-1β increased CXCL-8 concentrations in a murine model of trinitrobenzene sulphonic acid-induced colitis, whereas the effect was inhibited by the administration of S. boulardii through a decrease in the concentrations of inflammatory cytokines (CXCL-8, IL-1β, TNF-α and inducible NO synthase). These studies clearly support our findings of anti-inflammatory effects of S. boulardii on 5-FU-induced intestinal mucositis.
Another hypothesis for the beneficial effects of treatment with S boulardii on intestinal mucositis induced by 5-FU was based on decreased apoptosis. We demonstrated that treatment with S. boulardii prevents an increase in apoptosis in intestinal crypt cells in the jejunum and ileum induced by 5-FU. Studies have demonstrated that 5-FU chemotherapy increases the number of apoptotic cells in mouse intestinal crypt( Reference Wu, Han and Wang 38 , Reference Yasuda, Kato and Yamanaka 39 ) and the mechanisms involved in chemotherapy-mediated induction of small-intestinal cell apoptosis are associated with the presence of pro-inflammatory cytokines such as TNF-α and IL-1β( Reference Sonis 40 ).
Moreover, Soares et al. ( Reference Soares, Mota and Gomes 13 ) found 5-FU-induced intestinal mucositis to be associated with delayed gastric emptying/intestinal transit of liquids and with hypercontractility of the deep muscle of the stomach and duodenum in both the inflammatory and post-inflammatory phases. Patients receiving anticancer therapy commonly experience gastrointestinal symptoms, especially dyspepsia, dysphagia and diarrhoea( Reference Riezzo, Clemente and Leo 41 ), which are sometimes referred to as cancer-associated dyspepsia syndrome.
In the present study, S. boulardii reversed 5-FU-induced changes in gastrointestinal function, enhancing intestinal transit and gastric emptying and decreasing retention in the distal bowel segment, which may in part account for the observed attenuation of diarrhoea and weight loss. In parallel, the permeability of the small intestine of 5-FU-treated mice was significantly affected. The lactulose:mannitol excretion rate was significantly reduced by 5-FU treatment. A study has suggested that a reduction in mannitol absorption is related to the reduced surface area of intestinal mucosa and that increased lactulose absorption indicates a leaky gut( Reference Hollander 42 ). This finding is interesting because several authors have shown that the loss of integrity of the intestinal epithelial barrier can lead to bacterial translocation with the development of a systemic inflammatory response( Reference Prisciandaro, Geier and Butler 29 , Reference van Vliet, Harmsen and de Bont 43 , Reference Wardill and Bowen 44 ). Reinforcing our findings, Garcia Vilela et al. ( Reference Garcia Vilela, De Lourdes De Abreu Ferrari and Oswaldo Da Gama Torres 45 ) demonstrated that patients with inflammatory bowel disease present a significant change in mucosal integrity and that treatment with S. boulardii improves intestinal permeability in these patients. Thus, treatment with S. boulardii may be hypothesised to normalise bowel function by reducing inflammation associated with 5-FU-induced intestinal mucositis.
Few studies have evaluated the effects of probiotic bacteria on intestinal motility. Mediation of the relaxation of colonic motility by Bifidobacterium, Lactobacillus and Streptococcus has been suggested by one study( Reference Massi, Ioan and Budriesi 46 ). This mechanism could explain the observed attenuation of diarrhoea due to a reduction in stool frequency and restoration of the microbiota. Czerucka & Rampal( Reference Czerucka and Rampal 47 ) demonstrated that treatment with S. boulardii restored luminal electrolyte transport in cholera toxin-induced diarrhoea in the rabbit jejunum and suggested a mechanism involving cAMP-dependent chloride secretion. In addition, Budriesi et al. ( Reference Budriesi, Ioan and Micucci 48 ) used a mixture containing Castanea sativa and S. boulardii to induce anti-spasmodic and spasmolytic effects in segments of guinea pig intestinal smooth muscle that were contracted by carbachol, histamine, KCl and BaCl2, suggesting that the treatment's effect involved the inhibition of voltage-dependent Ca2+ channels.
According to McFarland( Reference McFarland 49 ), the protective effect of S. boulardii may involve different pathways classified as luminal, trophic or anti-inflammatory. These pathways include mechanisms such as maintenance of the integrity of the intestinal mucosa by preserving mucosal cell adhesion( Reference Rodrigues, Nardi and Bambirra 50 ), neutralisation of bacterial virulence factors( Reference Czerucka, Dahan and Mograbi 51 ) and increased immune response in the intestinal mucosa( Reference Brandao, Castro and Bambirra 52 ).
In conclusion, to our knowledge, this is the first study to evaluate the effect of administration of S. boulardii on the inflammatory and functional aspects of intestinal mucositis induced by 5-FU. Our findings will hopefully contribute to the discovery of novel probiotic-based treatments for gastrointestinal toxicity associated with anticancer therapy.
Acknowledgements
All sources of financial and material support were provided by a grant from CNPq and FUNCAP (Brazil). The funders had no role in study design, data collection and analysis, decision to publish or preparation of the manuscript.
The authors thank Maria S. F. França for excellent technical assistance in the experimental protocols.
The authors' contributions are as follows: P. F. C. J. and P. M. G. S. designed the study, conducted the study and data analysis and wrote the manuscript; A. A. L. conducted the study in vivo and data analysis; L. F. M. M., A. F. N., J. V. G. C., L. M. N. S., C. M. S., W. O. M., M. R. C. and A. X. F. conducted the study and data analysis; R. A. R. wrote the manuscript; M. H. L. P. S. designed the study and wrote the manuscript. All authors discussed, edited and approved the final version.
The authors declare that there are no conflicts of interest.