Until recently, the effect of vitamin D has been primarily associated with the regulation of Ca and phosphate balance of the body. However, vitamin D shows a broader range of activities since it affects both the skeletal and immune, cardiovascular, and endocrine systems(Reference Pludowski, Holick and Grant1). Nevertheless, the range and exact effect of vitamin D action are still debatable. Currently, vitamin D deficiency is associated with many chronic ailments related to oxidative stress, inflammation and ageing(Reference Bhattarai, Shrestha and Rokka2). A significant part of these disorders is civilisation diseases, such as obesity or diabetes(Reference Turer, Lin and Flores3,Reference Lips, Eekhoff and Schoor4) . The occurrences of vitamin D deficiency and civilisation diseases are strongly correlated with each other because both problems originate from a common source. The reason for their existence is the presently preferred – but far from natural – human lifestyle. Vitamin D status is a proxy for a healthy lifestyle; thus, it is difficult to assess whether significant deficiency in vitamin D is the cause or effect of illnesses like those mentioned above. However, vitamin D deficiency is a global issue, and the primary source of this deficiency is the widespread isolation of the skin from exposure to sunlight(Reference Ning, Song and Miao5,Reference Gromova, Doschanova and Lokshin6) . Since this phenomenon shows a progressive character, it is necessary to uncover precisely all mechanisms influencing vitamin D levels in the body. The selection of all the factors involved and thorough knowledge of the relationships between them will help to create principles for the effective and safe supplementation of vitamin D.
Numerous studies that have examined plasma 25(OH) vitamin D concentrations show significant differences between sexes(Reference Ning, Song and Miao5–Reference Luttmann-Gibson, Mora and Camargo9). Additionally, analysis of the National Health and Nutrition Examination Survey (NHANES) dataset indicates that vitamin D supplementation should be sex-dependent(Reference Wallace, Reider and Fulgoni10). Moreover, a transcriptome study investigating blood samples of persons supplemented with vitamin D revealed that vitamin D regulates 3·2 times more genes in women than in men(Reference Pasing, Fenton and Jorde11). In addition, many studies assessing the effects of vitamin D on disease units have demonstrated different outcomes in men and women. Despite this, many analyses relating to the impact of vitamin D have not included sex as an important factor. Further, experimental animal studies usually use only one sex to increase the standardisation of results. However, as many studies have indicated, sex can be important in determining the effectiveness of vitamin D supplementation.
The goal of this article is to review scientific reports that illustrate the occurrence of sex differences related to the effects and levels of vitamin D in mammals.
This article consists of four main parts. The first part explains how vitamin D is synthesised and metabolised, and the second part outlines which factors modulate vitamin D levels in the body. The third section characterises the differences between males and females in blood 25(OH) vitamin D. The last and largest part of the article is devoted to sex differences in the effect of vitamin D on various disease entities.
Synthesis and regulation of vitamin D levels
Vitamin D possesses a secosteroid structure similar to that of steroids(Reference Pérez-López12). In general, the term ‘vitamin D’ is most often used in reference to the different isoforms and metabolites of vitamin D.
There are two forms of vitamin D: D2 and D3. Both are the result of non-enzymatic reactions involving solar radiation(Reference Gholami, Moradi and Zareei13). Yeast, fungi and plants synthesise vitamin D2 or ergocalciferol. In those mentioned above, the substrate for the production of vitamin D2 is ergosterol (i.e. a compound that is part of cell membranes that performs the same function as cholesterol in animal cells). Under the influence of UVB radiation (280–315 nm), vitamin D2 is synthesised from yeast, fungi and plants from ergosterol. Vitamin D3, or cholecalciferol, is synthesised in animal tissues in a two-step process. The substrate needed to produce vitamin D3 is 7-dehydrocholesterol (7-DHC), whose level is regulated by 7-DHC reductase (DHCR7)(Reference Gholami, Moradi and Zareei13). Under the influence of UVB radiation, 7-DHC is converted in the skin of animals into previtamin D3, which isomerises to vitamin D3 (cholecalciferol l) as a result of the heat of the skin. The process of synthesising vitamin D3 in animal skin takes several hours. Initial products in the form of ergocalciferol and cholecalciferol are not biologically active (Fig. 1).
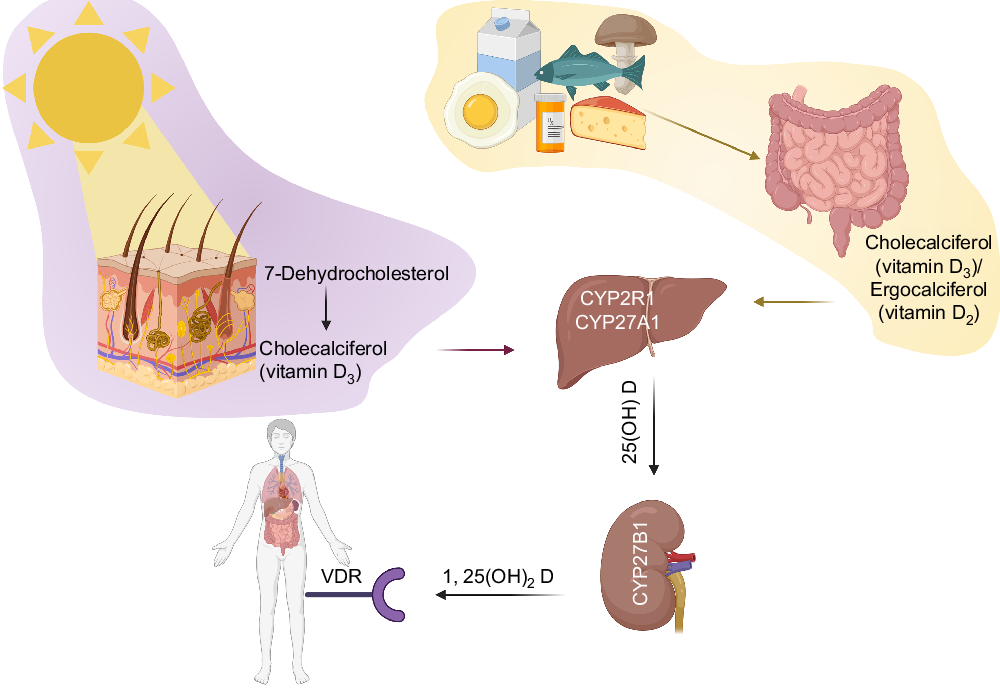
Fig. 1. The scheme shows the sources of vitamin D and its main activation pathway in the body. Vitamin D can be supplied to the body through dermal synthesis, during which 7-dehydrocholesterol is converted to cholecalciferol (D3) under the influence of UVB radiation and heat generated by the body. Vitamin D can also be taken orally with food or in the form of dietary supplements. Vitamin D taken orally may be in the form of D3 or D2. Regardless of its source or form, vitamin D enters the liver where it undergoes its first hydroxylation to form 25(OH)D by CYP2R1 and CYP27A1 enzymes. The 25(OH)D then reaches the kidney where it is converted to 1,25(OH)D under the action of the enzyme CYP27B1. 1,25(OH)D is the active metabolite of vitamin D and is recognised by the vitamin D receptor (VDR). (Figure made with BioRender). VDR, vitamin D receptor.
Vitamin D3, produced in the skin, binds to vitamin D transporting protein (DBP) in the bloodstream. DBP transports all vitamin D metabolites. Cholecalciferol and ergocalciferol from food and dietary supplements, after absorption from the intestines, enter the circulatory system, initially on chylomicrons, and then it is slowly transferred to DBP(Reference Pludowski, Holick and Grant1,Reference Bizzaro, Antico and Fortunato14) . As a result, cholecalciferol and ergocalciferol can be absorbed directly by peripheral tissues (e.g. muscle). However, cholecalciferol and ergocalciferol, bound to DBP, go mainly to the liver, where the first stage of its metabolism takes place. In the liver, 25-hydroxylases-CYP2R1 and CYP27A1 convert cholecalciferol and ergocalciferol into 25(OH) vitamin D (calcidiol – 25(OH)D)(Reference Sang-Min and Eun-Ae15). CYP2R1 in humans exhibits the highest levels of expression in the liver and testis microsomal fraction(Reference Kerschan-Schind16). 25(OH)D, resulting from 25-hydroxylation, is the primary circulating form of vitamin D. DBP has twenty times greater affinity for this form of vitamin D than the active form(Reference Fond, Faugere and Faget-Agius17).
The most important site for the next stage of vitamin D activation is the kidney. In mitochondrial kidney cells under the influence of 1α-hydroxylase-CYP27B1, 25(OH)D is hydroxylated to 1,25(OH)2 vitamin D (calcitriol – 1,25(OH)2D). The CYP27B1 enzyme is also active in the cells of other tissues. CYP27B1 expression has been observed, among others, in the liver, gonads, prostate epithelium, immune cells, thyroid cells and pancreas. However, CYP27B1 activity varies depending on the site of expression. In the kidney, the primary regulators of CYP27B1 action are changes in parathyroid hormone (PTH) and fibroblast growth factor 23 (FGF23), while at other locations, its activity is regulated by a large group of cytokines(Reference Kerschan-Schind16,Reference Grossi de Oliveira, Thereza Chaves and Alves Silva Menezesb18) .
1,25(OH)2D is the biologically active form of vitamin D produced by many organisms including mammals and plants(Reference Boland, Skliar and Curino19). 1,25(OH)2D enters the circulatory system, where after binding to DBP, it reaches the target tissues(Reference Passeron, Bouillon and Callende20). Calcitriol can perform its biological function thanks to its assembly with vitamin D receptor (VDR). VDR, belonging to the group of nuclear receptors, is located in most mammalian tissues. However, the level of its expression is tissue-dependent, being the most pronounced in the liver, intestines, bones, parathyroid glands and kidney (i.e. in tissues involved in maintaining the body’s Ca-phosphate homoeostasis)(Reference Pludowski, Holick and Grant1,Reference Wang, Zhu and DeLuca21) . VDR is dimerised together with the nuclear retinoid receptor RXR(Reference Kerschan-Schind16). Calcitriol in the bound form with the VDR–RXR complex regulates the transcription of many genes, including CYP27B1 and CYP24A1 (Reference Sang-Min and Eun-Ae15,Reference Kerschan-Schind16) .
The CYP24A1 enzyme is produced mainly in the kidneys, and it is responsible for calcidiol and calcitriol catabolism. 24-Hydroxylase-CYP24A1 metabolises 1,25(OH)2D and 25(OH)D, although it has a higher affinity for 1,25(OH)2D(Reference Fond, Faugere and Faget-Agius17). CYP24A1 inactivates these vitamin D metabolites in the form of calcitroic acid. The regulation of CYP24A1 expression levels is a major factor in maintaining normal levels of vitamin D in the body. Calcitriol, acting as a hormone, uses a negative feedback mechanism to strictly regulate vitamin D metabolism. Thanks to calcitriol-dependent CYP24A1 activity in the kidneys, it is possible to control both 25(OH)D and 1,25(OH)2D levels. Calcitriol also regulates the level of CYP27B1 in the kidneys, which is essential to prevent the accumulation of excessive levels of vitamin D in the body. In addition to using the negative feedback mechanism, vitamin D levels in the body are controlled by PTH, mentioned above, and FGF-23. The parathyroid glands secrete PTH in response to low blood Ca, and it stimulates CYP27B1 activity in the kidneys. FGF-23, produced by osteoblasts and osteocytes in response to too high levels of phosphates and calcitriol in the blood, inhibits the renal expression of CYP27B1 while enhancing CYP24A1 activity(Reference Passeron, Bouillon and Callende20). Moreover, calcitonin (i.e. a hormone produced mostly in thyroid C cells) affects the level of vitamin D in the body. Calcitonin is synthesised in a state of increased serum Ca. Lowering Ca levels due to calcitonin occurs by inhibiting osteoclasts formation(Reference Fond, Faugere and Faget-Agius17).
Interestingly, according to our recent study in rats, the expression of metabolising genes differs between sexes. In the liver, the expression of Vdr and Cyp2r1 genes is significantly higher in females compared with males, while the expression of Cyp27b1 does not differ significantly between the sexes. Significant differences in the expression of these genes were also observed in kidney tissue. Again, Cyp2r1 gene expression was higher in females, while the Cyp27b1 gene, only under the influence of vitamin D supplementation, showed higher expression in males compared with females. Moreover, this study revealed higher expression of Cyp2r1 and Cyp27b1 in the rat brain tissue in females compared with males. Furthermore, females have a higher expression of the Cyp27b1 gene in adipose tissue compared with males(Reference Oczkowicz, Szymczyk and Świątkiewicz22).
An exogenous vitamin can be delivered to the body in both the D2 and D3 forms. Both forms undergo the same, two-stage biological activation process. However, the effect of vitamin D2 diet supplementation shows a weaker effect(Reference Cipriani, Pepe and Piemonte23). Nevertheless, in the absence of skin exposure to UVB radiation, a varied diet cannot cover the daily requirement for calcitriol. Therefore, in many cases, vitamin D dietary supplementation is necessary. Despite the availability of many different dietary supplements with vitamin D, specific recommendations for their use remain controversial. Currently, the recommended doses refer to the general population and perhaps men and women should be treated differently in this regard. What is more, perhaps different recommendations should be applied depending on specific disease or condition. A routine way to determine the body’s vitamin D levels is by testing 25(OH)D in blood plasma. In most recommendations, >30 ng/ml (>75 nmol/l) is considered an appropriate outcome(Reference Pludowski, Holick and Grant1,Reference Holick, Binkley and Bischoff-Ferrari24) .
Sources of vitamin D and factors affecting its synthesis
Vitamin D is called the sun’s vitamin because the most efficient source is endogenous synthesis, starting in the skin under the influence of UV radiation. Therefore, a key element in maintaining proper levels of vitamin D in the body is regularly exposing the skin to the sun. Apart from recent limitations in exposure to UV rays related to the significant reduction in outdoor activities, attention should be paid to the popularity of cosmetics with UV protection filters. The use of cosmetics with a sun protection factor (SPF) 30 reduces the synthesis of vitamin D in the skin, as much as 99 %(Reference Dharambir, Sanghera and Christopher25). An additional obstacle to maintaining proper levels of vitamin D in plasma is the seasonal variability in the length and intensity of sun exposure. In many areas, the time and level of insolation change dramatically throughout the year, which leads to seasonal deficiency of vitamin D in humans(Reference Dharambir, Sanghera and Christopher25). Moreover, the range of seasonal variations in 25(OH)D in the blood depends on sex(Reference Shoben, Kestenbaum and Levin26,Reference Lagunova, Porojnicu and Lindberg27) .
The amount of vitamin D contained in the Western diet is low. The most vitamin D-rich foods are fatty ocean fish such as salmon or eel. Apart from that, vitamin D is present in eggs, yellow cheese, or milk; however, the daily requirement (1000 IU) from food can only be covered by consuming fish. The impossibility of simultaneously maintaining a varied diet and supplementing the daily requirement of vitamin D, as well as the widespread and robust reduction of skin contact with the sun, contribute to the need for vitamin D supplementation.
When determining the appropriate daily dose of vitamin D, attention was given to the recipient’s age, skin colour and physiological condition. Age is one of the best-proven factors correlated with vitamin D levels in the body(Reference Bhattarai, Shrestha and Rokka2). Metabolic processes in the elderly are significantly slowed down. Older people are also characterised by lower absorption of vitamins and minerals from the digestive tract, as well as a slowdown in the synthesis of cholecalciferol through the skin. Moreover, recommendations for vitamin D supplementation are correlated with women’s physiological status. The reason for these recommendations is fluctuations in oestrogenic concentrations associated especially with pregnancy and menopause.
Another important factor defining the accurate level of vitamin D supplementation is genetic background. Recently, much attention has been given to the effects of polymorphisms of genes involved in the action and metabolism of vitamin D(Reference Cummings, Cawthon and Ensrud28). For example, a meta-analysis performed by Duan et al. proved that the rs10741657 polymorphism of the CYP2R1 gene plays a vital role in determining the presence of vitamin D deficiency(Reference Duan, Xue and Ji29). Nevertheless, establishing the exact relationship between the genetic profile and the ability to maintain adequate vitamin D levels requires many years of research, and it seems unattainable now.
Among significant aspects influencing the status of vitamin D in the body, we should also mention obesity, low levels of physical activity(Reference Welsh, Doolin and McConnachie30–Reference Turhan, Doğan and Boğdaycioğlu32) and smoking cigarettes(Reference Hutchinson, Grimnes and Joakimsen33). For example, it was shown that obese men have a higher vitamin D deficiency index than men with normal body weight(Reference Lagunova, Porojnicu and Lindberg27). Moreover, according to a Norwegian study, seasonal variations in vitamin D do not appear to be strictly related to BMI in women, while obese men have much smaller seasonal variations than non-obese men. In addition, the analysis of NHANES 2011–2014 data indicates that the most significant differences in vitamin D levels are observed between obese women and women with normal body weight(Reference Jun, Cowan and Bhadra34).
One of the most important factors influencing the level of vitamin D synthesis in the body is skin colour. An increased amount of melanin in the skin guarantees its protection from the sun. Consequently, the darker one’s skin colour is, the weaker the synthesis of vitamin D in the skin(Reference Passeron, Bouillon and Callende20). This draws attention to the problem of people with high pigment levels living in sun-poor areas. The incidence of vitamin D deficiency is more than three times higher among African Americans than among Caucasians(Reference Kestenbaum, Katz and Boer7).
There is a growing number of indications that sex is an additional, crucial non-environmental factor regulating the level and effect of vitamin D(Reference Ning, Song and Miao5,Reference Muhairi, Mehairi and Khouri8,Reference Dharambir, Sanghera and Christopher25,Reference Lagunova, Porojnicu and Lindberg27,Reference Parka, Hamb and Lee35–Reference Jarrah, Mhaidat and Alzoubi38) . To date, a number of studies have been carried out in which vitamin D levels have differed between women and men, most of them defining the female sex as being more vulnerable to vitamin D deficiency(Reference Ning, Song and Miao5,Reference Kestenbaum, Katz and Boer7,Reference Hutchinson, Grimnes and Joakimsen33) .
Importantly, several studies confirm that sex hormones (androgens and oestrogens) affect the expression levels of genes related to vitamin D. The results of an experiment conducted on a human breast cancer (BC) cell line demonstrate that both dihydrotestosterone and estradiol-17 β (E2) increase the VDR gene expression(Reference Escaleira, Sonohara and Brentani39). The effect of E2 on Vdr expression was also confirmed in a study conducted on rat duodenal mucosa, which suggests that oestrogen up-regulates Vdr expression in this tissue and concurrently increases the responsiveness to endogenous 1,25(OH)2D(Reference Liel, Shany and Smirnoff40). Additionally, Schwartz et al. confirmed these results using several omics techniques. According to their findings, oestrogen increases VDR gene transcript level, protein expression and endogenous 1,25(OH)2D bioactivity in colonic mucosa(Reference Schwartz, Smirnoff and Shany41). Central nervous system studies also confirm the effect of E2 on Vdr gene expression(Reference Spanier, Nasholda and Mayne42–Reference Nashold, Spach and Spanier43). Analysis of the effect of E2 in T cells in vitro by Spanier et al. showed that E2 decreased Cyp24a1 expression and increased Vdr expression. This result suggests that E2 may slow the rate of calcitriol degradation while increasing the reactivity to calcitriol in T cells(Reference Spanier, Nasholda and Mayne42). Moreover, one of the more recent studies showed that testosterone may increase the catabolism of vitamin D. The result of this experiment indicates that testosterone induced CYP24A1 while inhibiting CYP27B1 gene expression in placental cell cultures(Reference Olmos-Ortiz, García-Quiroz and López-Marure44). Remarkably, not only do sex hormones affect vitamin D metabolism but also vitamin D affects sex hormone synthesis. According to one study, an active form of vitamin D stimulates the production of progesterone by 13 %, oestrogen by 21 % and estradiol by 9 % in cultured human ovarian cells(Reference Parikh, Varadinova and Suwandhi45).
Interestingly, the size of sex differences in plasma levels of vitamin D is correlated with ethnic origin. The most significant differences, regardless of age or health abnormalities, are between women and men from Latin American countries(Reference Martins, Wolf and Pan46).
As shown above, serum vitamin D levels are determined by many non-environmental and environmental factors. Moreover, factors such as area of residence, exposure time to UVB radiation, age, skin colour, sex, genetic profile, physiological state and BMI interact with each other, making the search for the causes of insufficient vitamin D levels very individual. Therefore, the idea of creating a personal index has recently emerged, which would enable the calculation of one’s own vitamin D requirement(Reference Carlberga and Haq47). However, this may require a precise determination of the significance of all factors that considerably modulate vitamin D levels, including the role of sex.
Sex as a non-environmental factor may influence both overall vitamin D levels in the body and may also modulate the body’s response to vitamin D supplementation in the prevention and treatment for various conditions. Both of these aspects are discussed below.
Sex differences in the serum levels of vitamin D
The results of numerous studies carried out in different countries regarding sex differences in the levels of vitamin D measured in plasma are inconsistent. A survey on a group of as many as 15 804 people revealed that in the USA, vitamin D deficiency is more common in men (P < 0001); however, an earlier study pointed out that women are more prone to vitamin D deficiency in this country(Reference Kestenbaum, Katz and Boer7,Reference Luttmann-Gibson, Mora and Camargo9) . The difference in the outcomes may be due to the fact that the first study was part of a clinical trial where the average age was 68 years, while the second study involved people over 65 years of age. On the other hand, Muhairi et al. conducted a study on a group of adolescents between 15 and 18 years of age in the United Arab Emirates. Their results showed that vitamin D deficits are much more prevalent in girls(Reference Muhairi, Mehairi and Khouri8). Similar findings were obtained by researchers in Brazilian adolescents and children aged 12–17 years. Additionally, in this case, 25(OH)D deficiency (≤ 20 ng/ml) was much more common in girls(Reference Lacroix de Oliveira, Cureau and Cople-Rodrigues48). Similarly, an analysis of the Taiwanese middle-aged and elderly population indicated that as many as 72·3 % of people with vitamin D deficiency were women, and in the group of people with adequate vitamin D levels, women constituted only 23·9 %(Reference Chia-Hung, Li-Kuo and Mei-Ju49). Lower vitamin D levels in females were also noted in a study performed on a group of people aged 5–101 years living in urban parts of China. According to the outcomes, the group most at risk of low levels, including severe plasma vitamin D deficits, consisted of females under 20 years of age and females over 80 years of age(Reference Ning, Song and Miao5).
Sexual differences in vitamin D levels are most evident in overweight and obese people, especially children(Reference Turer, Lin and Flores3). A cross-sectional survey with Brazilian children aged 12–17 years pointed to a significant relationship between weight category and hypovitaminosis D. According to the results, obese boys are particularly predisposed to vitamin D deficiency(Reference Lacroix de Oliveira, Cureau and Cople-Rodrigues48). Moreover, insufficient levels of vitamin D are especially common in young obese men(Reference Quaiz, Kazi and Fouda36). Similarly, Zhu et al. discovered that BMI and visceral obesity rates are significantly related to low serum concentrations of 25(OH)D in men(Reference Zhu, Chen and Li50). Additionally, Lagunov et al. demonstrated that women with obesity (BMI ≥ 40) had higher concentrations of 25(OH)D than men from the same BMI category. Approximately 40 % of obese women and 75 % of obese men have vitamin D deficiency during winter and spring(Reference Lagunova, Porojnicu and Lindberg27). Further, an epidemiological study by McCullough et al. confirmed the link between low levels of vitamin D and obesity in men(Reference McCullough, Weinstein and Freedman51). In contrast, the results of one of the latest cohort studies in Italy by Barrea et al. clearly signals that the level of vitamin D is significantly lower in women, regardless of the BMI class(Reference Barrea, Muscogiuri and Laudisio52). Moreover, the results of the analysis of patients before undergoing bariatric surgery indicate that as many as 72·5 % of them have insufficient levels of vitamin D. Interestingly, this deficiency was more often observed in women (77·5 % v. 69·2 %; P = 0·019)(Reference Ben-Porat, Weiss and Sherf-Dagan53). Recently, a correlation has also been found between vitamin D deficiency and the fat-free mass in the Italian population(Reference Muscogiuri, Barrea and Di Somma54). In addition, women deficient in vitamin D had higher levels of fat mass than men. The authors concluded that the lower 25(OH)D levels in women can be explained by the fact that generally women have more fat than men(Reference Muscogiuri, Barrea and Di Somma54). According to other research, the greater amount of body fat in women results in an ability to store more vitamin D from skin synthesis than in men, which also explains the fact that vitamin D levels are more variable over the year in men. Vitamin D accumulated in larger amounts of adipose tissue may be the reason for a more stable vitamin D level throughout the year in women than in men(Reference Lagunova, Porojnicu and Lindberg27).
The molecular basis for differences in 25(OH)D serum level between the sexes is not fully understood. Recently, an increase in 25(OH)D with the use of oestrogen-containing contraceptives was observed(Reference Harmon, Umbach and Baird55), which is in line with a large multi-ethnic study of men and women aged 45–84 years suggesting that lower 25(OH)D concentrations were associated with the lower sex hormone-binding globulin and higher free testosterone in both men and women(Reference Zhao, Ouyang and de Boer56). Moreover, it is hypothesised that females have a higher ability to synthesise vitamin D in the skin than males. In mice, it was shown that males exhibit lower levels of 25(OH)D after UV exposure than females because of a lower level of 7-DHC (the precursor to vitamin D) in male skin(Reference Rebel, Dingemanse-van der Spek and Salvatori57). Furthermore, it was revealed that women have higher DBP (vitamin D-binding protein) concentration which is combined with oestrogen-dependent DBP synthesis(Reference Lagunova, Porojnicu and Lindberg27). Undoubtedly, the fact that sex hormones affect the expression levels of genes related to vitamin D is of great importance. Nevertheless, it may not be the only reason since the research results of different studies are inconsistent, indicating both men and women have lower average levels of vitamin D depending on a study. Therefore, there might be other mechanisms responsible for the occurrence of these variances.
One possible explanation for the discrepancy between the results of different experiments may be the cultural differences in the lifestyles of men and women around the world. For example, a cross-sectional study carried out in Saudi Arabia showed that women exhibit a lower general exposure to the sun, although their knowledge about vitamin D importance is greater than in men (P < 0·002)(Reference Alharbi, Alharbi and Aljafen58). Moreover, they take vitamin D supplements more frequently than men(Reference Geddawy, Al-Burayk and Almhaine59). Furthermore, baseline characteristics of participants in the Vitamin D and OmegA-3 TriaL (VITAL) in the USA suggest more frequent consumption of foods containing vitamin D by women than men(Reference Donlon, LeBoff and Chou60). Conversely, in several regions of Europe, men tended to have higher intakes of vitamin D than did women (P < 0·01)(Reference Jenab, Salvini and Gils61).
Generally, the findings of studies regarding different levels of vitamin D in males and females are inconsistent (Table 1). Overall, it appears that in some geographical regions, women are at higher risk of vitamin D deficiency(Reference Yao, Fu and Li62). This tendency may change with an increasing public awareness of the impact of vitamin D on health. There are some interesting suggestions attempting to explain sex differences in vitamin D status, but so far, no comprehensive research has been conducted to identify the causes of this imbalance unequivocally.
Table 1. Sex differences in the level of vitamin D measured in the serum
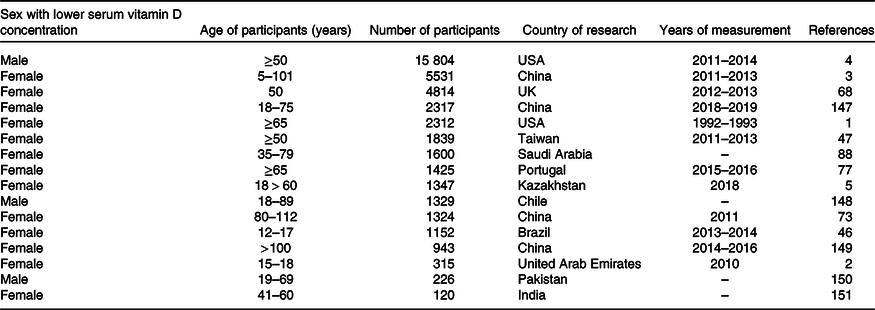
Sex differences observed in association studies examining the relationship between vitamin D status and the occurrence and course of skeletal and non-skeletal disorders
Recently, an increasing number of scientific articles have taken into account sex differences in the effectiveness of vitamin D in preventing and alleviating the course of human disorders. In this chapter, we aimed to systematise the most relevant articles on sex differences in the effect of vitamin D supplementation between females and males.
Skeletal and muscle disorders
Vitamin D deficiency in the body inhibits the absorption of Ca and P supplied from the diet. With significant deficits of vitamin D, only up to 15 % of Ca is absorbed from food. Low concentrations of Ca in the blood stimulate PTH synthesis. PTH increases Ca resorption in kidney tubules. Additionally, in the case of decreased Ca in blood plasma, PTH works similarly to the active form of vitamin D. Mammalian organisms are overly sensitive to fluctuations in Ca concentration in blood plasma. Therefore, when the plasma Ca level in plasma drops, 1,25(OH)2D interacts with VDR in bone blasts to cause preosteoclasts to mature. Mature osteoclasts, releasing hydrochloric acid and collagenases, dissolve bones, thanks to which Ca and phosphorus are released into circulation(Reference Dawson-Hughes, Harris and Krall63). For this reason, low levels of Ca and vitamin D in blood plasma cause abnormalities in the bone system. It is well known that a number of systemic hormones and transcription factors regulate bone turnover; however, the hormone whose action is best documented in this regard is oestrogen, which prevents bone loss via estrogen receptor (ER) α and induction of the Fas ligand (FasL) in osteoclasts(Reference Nakamura, Imai and Matsumoto64). Moreover, genetic variation at the ER locus, singly and in relation to the VDR locus, may influence bone mass and provide insight into the biological nature of the variation in susceptibilities to osteoporosis(Reference Willing, Sowers and Aron65). Nevertheless, the exact mechanism of the interaction between oestrogens and vitamin D is not fully understood.
Several investigations indicate that older women are characterised by lower bone mineral density than older men(Reference Ozturk, Gundogdu and Tonuk66–Reference Torsney, Noyce and Doherty67). Moreover, a body of evidence suggests that the risk of bone fractures depends on sex(Reference Cummings, Cawthon and Ensrud28,Reference Bischoff-Ferrari, Can and Staehelin68) . In the group of people between 65 and 70 years of age, women were 2·2 times more likely to have a non-vertebral fracture than men(Reference Cummings, Cawthon and Ensrud28). Another cross-sectional study revealed a correlation between the incidence of osteoporosis and Parkinson’s disease. Osteoporosis and osteopenia were found in as many as 91 % of women with Parkinson’s disease, while in the group of men with Parkinson’s disease, the same diseases were present in 61 % of respondents(Reference Ozturk, Gundogdu and Tonuk66). Interesting information is provided by a case study that searched for differences in the type of hip fracture in relation to 25(OH)D and PTH serum concentrations. Researchers have found, among other outcomes, that severe vitamin D deficiency and being female are associated with a more frequent recurrence of falls.
Further, a UK cross-sectional study, using data from the English Longitudinal Study of Aging (ELSA) and conducted with people over 50 years old, demonstrated that a higher plasma concentration of 25(OH)D lowers the risk of disability in women, but not in men(Reference Luiz, Máximo and Oliveira69). Moreover, the risk of basic activities of daily living disability is related to vitamin D deficiency in both sexes; however, in females, this risk is much higher (53 % v. 44 %)(Reference Luiz, Máximo and Oliveira69). In addition, a greater risk of female skeletal abnormalities associated with vitamin D deficiency was confirmed by a study on so-called residual rickets. Residual rickets is diagnosed in adults who have suffered rickets in childhood due to severe vitamin D deficiency(Reference Brickley, Mays and Ives70). The survey, carried out on a group of 200 persons (100 women and 100 men), who were not diagnosed with osteomalacia, showed that there were as many as 21 women among 29 subjects with residual rickets(Reference Veselka, Merwe and Hoogland71).
In turn, a study of people with inflammatory bowel disease listed men as a group with a higher risk of reduced bone mineral density associated with low levels of vitamin D(Reference Abraham, Prasad and Malaty72–Reference Dretakis and Igoumenou73). Additionally, multidimensional logistic regression models indicated a significant inverse relationship between the level of 25(OH)D and the risk of frailty and geriatric syndrome(Reference Xiao, Wu and Cui74). However, these studies have not proven that the relationship between 25(OH)D level and frailty risk is sex- or age-dependent. In contrast, an experimental study carried out on pregnant mice clearly underlines a different effect of vitamin D on offspring’s bones according to sex. Further, vitamin D supplementation in pregnant C57BL/6J mice improves bone strength and structure, but only in male offspring(Reference Villa, Taibi and Chen75). Similar information is offered by a cross-sectional study that traced the relationship between 25(OH)D levels in two stages of pregnancy and bone measurements in offspring at the age of 11 years. This study signalled a positive correlation between 25(OH)D in the mother in early pregnancy and bone mineral content and density in boys. A similar relationship was not observed in girls(Reference Hyde, Brennan-Olsen and Mohebbi76).
Further information about the different effects of vitamin D on females and males is provided by research on sarcopenia (i.e. loss of muscle mass and strength). The study indicated that the sarcopenic condition was significantly related to total serum 25(OH)D concentration and cholesterol level in females only, and PTH level in males only(Reference Parka, Hamb and Lee35). Additionally, cross-sectional study demonstrated that vitamin D levels were positively correlated with relative skeletal muscle mass in males with sarcopenia(Reference Kuo, Wang and Liu77). Another study was conducted on a group of people aged 65 years to determine the relationship between vitamin D deficiency and the lowest values of gait speed and hand grip. The results revealed a relationship between vitamin D deficiency and gait speed and grip strength. This relationship was particularly important in the male group compared with the female group(Reference Mendes, Santos and Borges78).
In sum, women are more likely to suffer damage and abnormalities in the functioning of the skeletal system associated with low levels of vitamin D than men. In turn, the relationship between vitamin D deficiency and muscle mass and strength seems to be stronger for men than for women.
Cardiovascular and cardiometabolic disorders
The importance and complexity of functions and the associated ubiquitous presence of blood vessels make CVD not only one of the most dangerous, but also one of the most common conditions in the world. Many studies have linked the functioning of the circulatory system with vitamin D(Reference Welsh, Doolin and McConnachie30,Reference Gholami, Moradi and Zareei79,Reference Kim, Perrelli and Ragni80) .
Moreover, many indicate different effects of vitamin D in women and men. Further, studies have confirmed the positive impact of vitamin D supplementation on the cardiovascular system and connected its deficiency to an increased risk of CVD(Reference Martins, Wolf and Pan46,Reference Gholami, Moradi and Zareei79,Reference Kim, Perrelli and Ragni80) . Low vitamin D content may lead to increased renal renin and angiotensin II production, consequently increasing blood pressure and leading to the development of hypertension and systemic and vascular inflammation(Reference Parikh, Varadinova and Suwandhi45,Reference Anderson, May and Horne81) . In the USA, a survey was carried out on a group of over 15 000 people concerning the connection of vitamin D with the occurrence of CVD ailments. The results verified that low levels of vitamin D in plasma were significantly related to factors increasing the risk of CVD, such as inadequate blood pressure, diabetes mellitus, high BMI and high TAG levels(Reference Martins, Wolf and Pan46). A clinical study in rats also established that the occurrence of heart disease is associated with low levels of vitamin D, which suggests the usefulness of vitamin D supplementation in treating heart failure(Reference Przybylski, Mccune and Hollis82). Moreover, a recent study showed that even short-term use of vitamin D affects the expression of genes associated with CVD(Reference Guénard, Jacques and Gagnon37).
One experiment examined how excess androgens, in combination with vitamin D deficiency, affect the thickness of the anterior cerebral arteries (ACA) in cerebrovascular disease in rats. The obtained results proved that females with vitamin D deficiency and excess androgens are characterised by increased ACA wall thickness, whereas among males, vitamin D deficiency alone is sufficient to increase ACA wall thickness. Moreover, immunohistochemical investigation indicated that vitamin D deficiency does not significantly affect the expression of androgen receptor (AR) protein in ACA walls in females, but in males, it is significantly higher than in females. This study proves the significance of vitamin D in correlation with androgens for ACA vasoconstriction, which reduces the cerebral circulation efficiency leading to stroke(Reference Pal, Hadjadj and Fontanyi83), implying that men are more likely to reduce cerebral circulation efficiency in the case of vitamin D deficiency than women.
Other evidence of sex differences in vitamin D action is provided by the results of a clinical study on total antioxidant capacity among candidates for coronary artery bypass grafting surgery. An appropriate total antioxidant capacity level in the body prevents CVD. According to the findings of the study, a higher serum vitamin D content was accompanied by a higher total antioxidant capacity, but only in males, suggesting that the appropriate level of vitamin D, especially in the male diet, can help to protect against cardiometabolic risk factors(Reference Farhangi and Najafi84). In contrast, a cross-sectional study on the relationship between cardiovascular mortality and vitamin D levels, based on data from the Swiss MONICA study, showed that serum 25(OH)D was inversely proportional to cardiovascular mortality in women, but not in men(Reference Rohrmann, Braun and Bopp85). The outcomes of the coronary artery disease study by Verdoia et al. also provide interesting information. According to their findings, low levels of vitamin D are associated with a higher incidence and severity of coronary artery disease in women. In the case of men, the status of vitamin D is independently related to the incidence of coronary artery disease, but not to its severity(Reference Verdoia, Schaffer and Barbieri86), signalling that woman have a worse course of CVD due to vitamin D deficiency than men. Contrary, Jarrah et al. study did not confirm the relationship between vitamin D and coronary artery disease in either men or women(Reference Jarrah, Mhaidat and Alzoubi38).
Type 2 diabetes mellitus (T2DM) and metabolic syndrome increase the probability of death from cardiovascular causes. The leading mechanism proposed to explain the relationship between vitamin D deficiency and predisposition to metabolic syndrome and T2DM increased insulin resistance and dysfunction of pancreatic β cells(Reference Lips, Eekhoff and Schoor4,Reference Anderson, May and Horne81) . Some investigations hint that vitamin D supplementation may prevent abnormalities in glucose metabolism and thus the development of T2DM and metabolic syndrome(Reference Pittas, Lau and Hu87). However, research in this area presents very inconsistent results(Reference Lips, Eekhoff and Schoor4,Reference Pittas, Lau and Hu87) .
Abudawood et al. indicated the relevance of the link between vitamin D deficiency and the occurrence of T2DM as a factor increasing the risk of CVD. This study compared glycaemic indexes, lipid profiles and vitamin D levels of healthy individuals and those with T2DM. The level of vitamin D in patients, regardless of sex, was significantly lower than in healthy people. Vitamin D and total cholesterol levels were positively correlated in both sexes; however, the significance of this correlation was demonstrated only in men(Reference Abudawood, Tabassum and Ansar88). In contrast, apparent intersex differences in vitamin D activity were found by analysing the relationship between vitamin D supplementation and apo expression(Reference Al-Daghria, Wania and Sabicoa89). The study revealed that under the influence of vitamin D supplementation, the production of apo C2 and C3 increased significantly, but only in men. Interestingly, the expression of apo C1 rose significantly, but only in women. At the same time, under the influence of vitamin D, the expression of apo B fell significantly in women only. These results led the authors to conclude that sexual dimorphism, in the modulatory action of lipoproteins in circulation, may explain the sex imbalance in cardiometabolic health(Reference Al-Daghria, Wania and Sabicoa89). Similarly, a cross-sectional study on MetS indicated potential differences in vitamin D activity between the sexes. The results of multifactorial logistic regression analysis, using data collected in this study, demonstrated that vitamin D deficiency (below 20 ng/ml) was associated with an increased risk of MetS in men only(Reference Kim, Lee and Ha90). Further, participants from the Asian Indian Diabetic Heart Study exhibited sex differences in the association of serum vitamin D deficiency with increased risk for cardiometabolic disorders in 3879 Asian Indians(Reference Dharambir, Sanghera and Christopher25). The authors observed that vitamin D deficiency and obesity may together have additive effects on cardiometabolic risk. However, the association of serum vitamin D with fasting blood glucose and homoeostasis model assessment (HOMA-B) in normoglycemic individuals was significant, but only in males(Reference Dharambir, Sanghera and Christopher25).
Another study pointed out that women with vitamin D deficiency are characterised by a younger age and more frequent occurrence of dyslipidaemia than men. Further analysis based on the results of this study inferred a significant relationship between vitamin D deficiency and increased cardiometabolic risk (including high DBP, high total cholesterol, low-density lipoprotein and TAG) in men. However, the link between vitamin D deficiency and cardiometabolic risk in women was weaker(Reference Chia-Hung, Li-Kuo and Mei-Ju49). The cross-sectional study conducted by Quaiz et al. signalled that the risk of low HDL cholesterol associated with 25(OH)D deficiency was 2·1 times higher in men and 1·3 times higher in women than in their counterparts with normal vitamin D status. Simultaneously, an increased probability of having high TAG levels associated with 25(OH)D deficiency was observed in females, but not in males(Reference AlQuaiz, Kazi and Youssef91).
Recently, a quantitative, serum proteomics study in a cross-sectional cohort of non-diabetic adults with obesity and low v. high vitamin D status revealed sexual dimorphism in vitamin D action. According to the results, a high status of vitamin D correlates with high expression of serum insulin-like growth factor 2-binding protein (IGFBP-2), but only in men with obesity. Further, the association between vitamin D level and the expression of IGFBP-3 protein is positively regulated, but only in obese women with high vitamin D levels. Moreover, the authors observed that IGFBP-2 and IGFBP-3 may serve as markers of risk reduction in cardiometabolic disease(Reference Al-Daghri, Manousopoulou and Alokail93).
Additionally, studies on non-alcoholic fatty liver disease have shown a different effect of vitamin D supplementation on alanine aminotransferase levels in women and men. The results of the cross-sectional analysis of the population cohort revealed that vitamin D deficiency was associated with elevated alanine aminotransferase levels only in men with normal body weight. A similar relationship was not observed in any of the BMI classes in women. Therefore, the authors stressed the need to conduct further studies on the relationship between vitamin D and non-alcoholic fatty liver disease, taking sex into account(Reference Zelber-Sagi, Zur and Thurm92).
Maintaining a normal level of vitamin D in the body in the context of cardiometabolic health seems more important for men than for women. Importantly, a clinical study confirmed the therapeutic effect of vitamin D on the cardiovascular system of men only(Reference Pal, Hadjadj and Fontanyi83). In addition, men are more frequently victims of CVD, but women have a worse course of events of this kind(Reference Gao, Chen and Sun94).
Nervous system disorders
The effect of vitamin D supplementation on the occurrence and course of multiple sclerosis (MS) is currently the best proven relationship among all non-skeletal diseases. MS is a chronic, progressive disease that leads to increasing disability in many individuals. The clinical status of people with MS is routinely determined using the Expanded Disability Status Scale (EDSS). Kragt et al. detected a negative correlation between EDSS scores and 25(OH)D levels(Reference Kragt, Amerongen and Killestein95). Moreover, high levels of vitamin D were associated with a lower risk of MS, but only in women(Reference Kragt, Amerongen and Killestein95). The prevalence of MS increases with latitude, which verifies the negative influence of low concentrations of vitamin D on the occurrence of this disease(Reference Kurtzke96). MS is diagnosed most frequently in women of reproductive age (female:male ratio 3:1), whereas the incidence of MS in pre-pubertal and postmenopausal women is equal compared with men(Reference Krysko, Graves and Dobson97). Vitamin D3 levels in the global population have dropped dramatically over the past few decades(Reference Adams and Hewison98), correlating with growing female MS incidence. Therefore, decreased vitamin D status may be a reason for women’s higher susceptibility to MS(Reference Spanier, Nasholda and Mayne42). Several other studies indicate a different vitamin D metabolism in females and males with MS. Kragt et al. showed that a 10 nmol/l increase in serum 25(OH)D levels in women reduces the risk of MS by up to 19 %(Reference Kragt, Amerongen and Killestein95). Moreover, Woolmore et al. confirmed that women with MS, with increased sensitivity to sunlight, have a lower risk of a severe course of disease(Reference Woolmore, Stone and Pye99).
A study conducted on a group of people with MS showed that the active form of vitamin D inhibits T cell proliferation in women much more strongly than in men(Reference Correale, Ysrraelit and Gaitán100). Moreover, the increase in the number of regulatory T lymphocytes induced by 1,25(OH)2D was higher in a group of females with MS. Additionally, exposure to vitamin D reduced the number of cells producing IFN-γ- and IL-17 and increased the number of cells producing IL-10. In this case, the effect of vitamin D was also stronger in females. Interestingly, exposure to the active form of vitamin D in healthy people and those suffering from MS caused a significant increase in CYP24A1 expression in T cells, but only in males. In this study, the amount of DBP associated with T cells in female patients with MS was 2·5 times higher than in male patients with MS. Moreover, male cells treated with 17-β estradiol showed a similar response to 1,25(OH)2 D as female cells(Reference Correale, Ysrraelit and Gaitán100). These findings suggest that women have a better ability to accumulate the active form of vitamin D; thus, it has a stronger anti-inflammatory effect in women than in men.
The results of the study, aiming to compare the levels of vitamin D and FGF23 metabolites in subjects with MS and in healthy controls, indicated that the concentration of DBP was higher in patients with MS than in healthy subjects (P = 0·02). This difference was particularly significant in men. However, the authors suggested that the role of DBP in MS, as well as sex differences in the VD-FGF23 axis, require further research(Reference Vlot, Boekel and Kragt101).
Furthermore, experiments on an animal model for investigating MS, mice with autoimmune encephalomyelitis (EAE), revealed that vitamin D3-fed female mice had significantly less clinical, histopathological and immunological signs of EAE(Reference Spach and Hayes102). Moreover, intact female mice fed the feed with vitamin D had significantly more 1,25(OH)2D and fewer CYP24A1 transcripts in the spinal cord than ovariectomised females, intact or castrated males. The authors hypothesised that an ovarian hormone inhibited CYP24A1 gene expression in the spinal cord, leading to the locally produced 1,25(OH)2D and its accumulation, which may prevent the inflammation before severe EAE developed(Reference Spach and Hayes102). On the other hand, a study conducted by Correale et al. suggested that T cells in females and males synthesise similar amounts of 1,25(OH)2D, although females activate it slower, thus its accumulation in self-reactive T cells 1,25(OH)2D(Reference Correale, Ysrraelit and Gaitán100).
Additionally, Nashold et al. proved that female mice with ovarian hormone production disorder have lost the protective effect of vitamin D against EAE. This study also revealed that despite the administration of E2 (estradiol) to males, vitamin D did not inhibit EAE disease. The results indicate that vitamin D increases the synthesis of E2 and the expression of VDR only in females, which favours vitamin D’s therapeutic effect on EAE disease(Reference Nashold, Spach and Spanier43). These observations provide evidence there are synergies between the action of oestrogen and 1,25(OH)2D in MS regulation, which suggests vitamin D supplementation has greater potential to prevent autoimmune-related nervous system diseases in women than in men.
Research on Alzheimer’s disease (AD) has also detected sex differences in response to vitamin D supplementation. AD is one of the most common, incurable and progressive neurodegenerative ailments. Age and being female increase the risk of AD(Reference Zhu, Beal and Wang103). According to the results of one study, women are 2·6 times more likely to develop this disease than men(Reference Ouma, Suenaga and Bölükbaşı Hatip104). Studies have confirmed the positive role of vitamin D in the treatment or preventing of AD(Reference Gangwar, Rawat and Tiwari105). Morello et al. established that vitamin D supplementation improves the working memory of mice with AD. In females with AD, chronic vitamin D supplementation was effective for working memory and amyloid loading only when taken during the symptomatic phase. The authors pointed to the need for additional preclinical studies to better understand the sex-specific mechanisms of vitamin D action in AD(Reference Morello, Landel and Lacassagne106). Additionally, Ouma et al. revealed sex differences in relation to vitamin D and mild cognitive impairment and various stages of AD. In the case of mild cognitive impairment, 25(OH)D and 1,25(OH)2D were correlated with male sex, whereas in the case of AD, this correlation occurred in women(Reference Ouma, Suenaga and Bölükbaşı Hatip104).
Interesting results are also provided by another study on the therapeutic effect of vitamin D supplementation. This study involved a group of people with severe depressive disorders (major depressive disorder) who underwent 3 months of vitamin D supplementation. The effect of vitamin D in this study was determined using the Beck Depression Inventory (BDI) scale. Females with moderate, severe and extreme depression had significantly lower BDI results after vitamin D treatment (P < 0·05). In males, a significant improvement in the BDI score (P < 0·05) was diagnosed only in the group with severe depression(Reference Alghamdi, Alsulami and Khoja107). The findings suggest that vitamin D therapy may be helpful in treating major depressive disorder, especially in women.
Further, Lowe et al. investigated whether vitamin D affects functional results in a rat model of infant hypoxia and ischemia(Reference Lowe, Fraser and Rollins108). According to the outcomes, treatment with HNAC and vitamin D (Hypothermia + NAC N-acetylcysteine +1,25(OH)2D) significantly reduced the incidence of serious brain pathologies in males, while in females, there were no differences between the use of HNAC (NAC + hypothermia treatment) and HNAC + Vit. D therapy. In addition, HNAC + Vit. D improved motor skills compared with hypothermia (HYPO) treatment alone; however, there were some sex differences (P = 0·01). In general, a beneficial effect of vitamin D supplementation on asymmetry of the feet was observed (P = 0·001). However, males showed significantly greater asymmetry of the feet than females. Additionally, HNAC + Vit. D improved sensory motor adaptation after hypoxia and ischemia more in male rats than in females. Importantly, hypoxia and ischemia injury increased the CYP24A1 enzyme for vitamin D degradation, particularly in males, and males benefit more from 1,25(OH)2D treatment in the long term(Reference Lowe, Fraser and Rollins108). A study on changes in fetal neurodevelopment with maternal vitamin D deficiency also provides interesting information(Reference Hawes, Tesic and Whitehouse109). Mice that were fed vitamin D or vitamin D-deficient feed before and during pregnancy were used as a research model. Here, too, the researchers indicated sex differences in response to supplementation or lack thereof. Morphological analysis of the brains obtained from fetuses signals that a deficiency of vitamin D during pregnancy leads to a reduction in crown-rump length, head size and lateral ventricle volume. Additionally, Foxp2-immunoreactive cells were reduced in the cerebral cortex of female fetuses with vitamin D deficiency. In the case of female fetuses, the expression of tyrosine hydroxylase was also reduced(Reference Hawes, Tesic and Whitehouse109).
The outcomes of the quoted studies underscore both the protective role of vitamin D and sexual dimorphism in relation to vitamin D metabolism in the nervous system. The scientific evidence presented here implies that women are much more at risk from diseases of the nervous system. However, this research also suggests that vitamin D appears to have a stronger therapeutic effect in females with nervous system diseases than in males.
As in the case of CVD, there are many uncertainties about the link between vitamin D and the nervous system(Reference Bivona, Gambino and Iacolino110). However, numerous studies stresses that vitamin D is involved in the pathophysiology and progression of many neurological illnesses(Reference Bhattarai, Shrestha and Rokka2,Reference Luiz, Máximo and Oliveira69) . In addition, low levels of vitamin D are diagnosed in patients with nervous system diseases such as MS and AD(Reference Nashold, Spach and Spanier43).
Cancer
Due to the contribution of vitamin D to the regulation of the survival, proliferation and differentiation of cells, its action is also often associated with the pathogenesis of cancer. It was shown that low levels of vitamin D in serum, regardless of age, sex or BMI, are correlated with advanced cancer(Reference Churilla, Brereton and Klem111). Additionally, a number of studies revealing the link between latitude and the incidence of cancer have confirmed the protective role of vitamin D. For example, Cuomo et al. conducted a study in 172 countries. The incidence of leukaemia was inversely proportional to the intensity of UVB radiation in both women (P < 0·01) and men (P < 0·01)(Reference Cuomo, Garland and Gorham112). In turn, Tas et al. proved that the level of 25(OH)D in blood serum is not related to the duration and activity of the disease in patients with melanoma(Reference Tas, Erturk and Soydinc113). Additionally, a recent study (VITAL) carried out in the USA on a group of more than 25 000 people has not confirmed that vitamin D supplementation results in a lower incidence of invasive cancers(Reference Manson, Cook and Lee114). Therefore, the role of vitamin D in the cancer pathogenesis is still debated.
However, there is also evidence of different effects of vitamin D in female and male organisms in cancer research. For example, a cross-sectional study carried out in the Swiss population revealed that an increase in 25(OH)D concentration of 10 ng/ml reduces overall mortality by as much as 20 %. In addition, the study pointed to an inverse relationship between 25(OH)D concentration and cancer mortality, but only in males(Reference Rohrmann, Braun and Bopp85). Interesting information has been provided by a study on the expression of genes involved in vitamin D metabolism in individuals with colorectal cancer (CRC). In this study, the sex-specific analysis showed a significant difference in CYP27B1 gene expression between rectal and CRC in women but not in men. Additionally, in tumours located in the distal part of the large intestine, the expression of the CYP27B1 gene was more than twice as high in men as in women(Reference Brozek, Manhardt and Kállay115). The association study between vitamin D, the VDR gene and the androgen receptor gene with colorectal and rectal cancer also provides interesting information. The AR gene partially regulates the transcriptional activity of the VDR gene. A significant interaction between vitamin D and the AR gene and the risk of CRC was detected only in men. Men with lower vitamin D intake and lower sun exposure are more likely to develop CRC(Reference Slattery, Sweeney and Murtaugh116). In turn, a study on polymorphisms of the VDR gene and CRC confirmed that the BsmI variant is significantly associated with CRC in women(Reference Alkhayal, Awadalia and Vaali-Mohammed117). Additionally, studies on colorectal adenomas suggest sex-dependent effects of vitamin D. In general, according to the outcome of this study, colorectal adenomas are much more common in men than in women (P < 0·001); however, in men, the level of vitamin D was not associated with the place or amount of lesions in the large intestine. In women, on the other hand, the total number of neoplastic lesions was inversely correlated with the serum level of vitamin D(Reference Aigner, Stadlmayr and Huber-Schönauer118). Similar conclusions were reached by researchers from Arizona. Admittedly, they did not find a significant statistical relationship between 1,25(OH)2D and the chance of metachronic adenoma. Yet according to their results, women – but not men – with the highest concentration of 1,25(OH)2D have a significantly reduced chance of developing adenomas in the distal part of the large intestine. In addition, these researchers suggest that the supply and activity of vitamin D metabolites in different parts of the large intestine may vary according to sex(Reference Hibler, Molmenti and Lance119). Further, research on the carcinogenesis of esophageal adenocarcinoma implies a different effect of vitamin D on females and males. Zhou et al. verified a significantly higher level of VDR expression in precancerous lesions and esophageal adenocarcinoma in males than in females(Reference Zhou, Xia and Bandla120). In another study, a high expression of TGR5, a cell membrane surface receptor-G protein coupled receptor associated with the development of gastrointestinal cancer, was significantly increased in males compared with females. Moreover, VDR was significantly correlated with TGR5 expression. According to the authors of this study, sex differences in TGR5 and VDR expression may explain why the prevalence of esophageal adenocarcinoma is higher in men than in women(Reference Pang, LaLonde and Godfrey121).
On the other hand, the results of a large population study in China revealed an unexpected direct link between higher serum concentrations of 25(OH)D and an increased risk of esophageal squamous cell carcinoma in men, but not in women(Reference Chen, Dawsey and Qiao122). The detrimental effect of higher vitamin D levels on non-Hodgkin’s lymphoma was observed in the San Francisco Bay Area population(Reference Mikhak, Gong and Bracci123). The authors indicated that high levels of vitamin D are associated with an increased risk of non-Hodgkin’s lymphoma, which is surprisingly more significant in men than in women. However, as the authors themselves pointed out, this study is ambiguous and needs to be continued(Reference Mikhak, Gong and Bracci123).
Suggestions about the sex-dependent effect of vitamins can be found in a study on lung cancer conducted in Finland. This study did not demonstrate a generally significant link between vitamin D and the risk of lung cancer. However, serum concentrations of 25(OH)D were inversely related to lung cancer incidence in women (P < 0·001), but not in men (P = 0·81)(Reference Kilkkinen, Knekt and Heliövaara124).
When considering the effects of vitamin D supplements in women and men, we must take into account its impact on various types of cancer that occur only, or predominantly, in one sex, that is, ovarian, uterine and BC in women, and prostate cancer in men. There is a strong theoretical support for the beneficial effects of vitamin D in preventing BC. First of all, vitamin D has the potential to reduce progesterone and estradiol, which is connected with decreasing BC risk in young women. Moreover, it was shown that ER-positive BC cells treated with 1,25(OH)2D induce a cell cycle shutdown.
Available data strongly suggest an association of 25(OH)D with BC risk in women(Reference de La Puente-Yagüe, Cuadrado-Cenzual and Ciudad-Cabañas125–Reference Estébanez, Gómez-Acebo and Palazuelos127). The analysis by Song et al. showed that a 5 nmol/l increase in blood vitamin D levels was associated with a 6 % decrease in BC risk(Reference Song, Deng and Liu126). Furthermore, epigenetic analysis has shown that the interaction of 25(OH)D levels with methylation of CpG in vitamin D-related genes (specifically cg21201924 in RXRA) may influence BC risk(Reference O’Brien, Sandler and Xu128). Moreover, in the case of the most lethal gynaecological cancer among women, that is, ovarian cancer, the beneficial effect of 1,25(OH)2D was observed(Reference Guo, Guo and Xie129–Reference L’Espérance, Datta and Qureshi131). For example, the results of the Prejovic et al. study suggest a chemo-preventive role of vitamin D in the development of epithelial ovarian cancer in women. This study investigated the association between vitamin D and ovarian cancer development in breast cancer susceptibility gene (BRCA) mutation carriers. BRCA gene mutation is strongly associated with the occurrence of epithelial ovarian cancer. The results showed that VDR protein levels in BRCA1mut ovarian surface epithelial cell cultures treated with calcitriol were increased by about 95 % compared with untreated cells. Furthermore, the same cancer cells treated with calcitriol showed a lower proliferation rate in a dose-dependent manner(Reference Pejovic, Joshi and Campbell132).
Another possible target for vitamin D is the prostate gland. In men with prostate cancer, higher 25(OH)D plasma concentrations were associated with longer survival(Reference Mondul, Weinstein and Moy133). A controlled clinical trial by Deschasaux et al. found that high 25(OH)D concentrations were associated with a reduced rate of prostate cancer(Reference Deschasaux, Souberbielle and Latino-Martel134). Furthermore, a randomised controlled trial by Li et al. showed that both 25(OH)D and 1,25(OH)2D may play an important role in preventing prostate cancer progression(Reference Li, Stampfer and Hollis135). In addition, these researchers showed that the VDR FokI polymorphism and plasma 25(OH)D concentration interact and alter prostate cancer risk. According to this study, men with low 25(OH)D concentrations and the FokI ff genotype are at the highest risk of developing prostate cancer. Similarly, high plasma 25(OH)D concentrations correlated with an approximately 70 % reduction in the risk of aggressive prostate cancer in men with the ff genotype(Reference Li, Stampfer and Hollis135).
Vitamin D exhibits a number of actions, including anti-inflammatory activity, indicating that it may influence the process of cancer formation(Reference Giangreco, Dambal and Wagner136). However, not all studies confirm the beneficial effect of vitamin D on preventing cancerogenesis. Remarkably, the study of the population of 217 244 individuals in Denmark revealed that vitamin D levels are not associated with the incidence of several major cancer types including breast, ovary or corpus uteri cancer, but higher vitamin D concentrations are associated with a significantly higher incidence of skin, prostate and haematological cancer, as well as a lower incidence of lung cancer(Reference Vojdeman, Madsen and Frederiksen137). Although these are correlations, and do not indicate a direct negative impact of vitamin D supplementation on the development of prostate cancer, they show how important it can be to understand sex-specific vitamin D actions.
Overall, the current knowledge does not allow us to regard vitamin D as a strong anticancer agent yet, and it only indicates the need to maintain its blood level within the laboratory norms. Furthermore, there are many scientific indications of potentially different effects of vitamin D on tumour formation in females and males.
Immunity/autoimmunity
The link between vitamin D action and immune system function has been known for a long time. The beneficial effects of vitamin D on tuberculosis were observed in 1943(Reference Dowling, Gauvain and Macrae138). However, only a few years ago, it was found that calcitriol induces the expression of antimicrobial peptides, such as CAMP and DEFB4(Reference Gombart139). Additionally, several meta-analyses(Reference Autier, Mullie and Macacu140) have proven the beneficial effect of vitamin D supplementation on the incidence of upper respiratory tract infections. However, it is not known whether vitamin D improves the immunity of males and females to the same extent, or whether it is responsible to some degree for the differences between female and male immunity. Currently, the improvement of immunity is of great importance due to the widespread COVID-19 infection. Recently, it has been hypothesised that the interaction between vitamin D and oestrogen could affect the sex imbalance in the outcomes of patients with COVID-19(Reference Pagano, Peruzzu and Ruggieri141). However, further research to elucidate these relationships is still required. A good example of the investigation of sex hormones and vitamin D relationships in the context of immunity is a study by Olmos-Ortiz et al., which points out that male newborns are less resistant to postpartum infections(Reference Olmos-Ortiz, García-Quiroz and López-Marure44). At the same time, cotyledons of male fetuses expressed lower CYP27B1 gene expression than those of female fetuses. This led to lower local production of active vitamin D, and consequently, to lower expression of the antimicrobial peptide cathelicidin(Reference Olmos-Ortiz, García-Quiroz and López-Marure44). Sex differences were also observed in the immune system response to vitamin D through the secretion of inflammatory cytokines (TNF-α and IL-6) in human adipose tissue. According to the results, 25(OH)D and 1,25(OH)2D reduced the lipopolysaccharide-induced increase in cytokine concentration in adipose tissue in women, but not in men(Reference Roy, Nadeau and Valle142). Further, a randomised control trial that investigated the effect of long-term vitamin D supplementation on gene expression in blood identified a much stronger effect in women than in men. Gene set enrichment analysis highlighted the engagement of identified genes in the immune system, among others(Reference Pasing, Fenton and Jorde11).
Strong evidence of the importance of sex in the action of vitamin D in shaping immunity is provided by studies on autoimmune diseases. The occurrence of autoimmune diseases such as MS, systemic lupus erythematosus, rheumatoid arthritis, irritative bowel syndrome or Hashimoto’s disease is much more common in women than in men(Reference Kim and Kim143–Reference Ngo, Steyn and McCombe144). However, male sex is associated with more severe clinical manifestations of these ailments(Reference Bizzaro, Antico and Fortunato14). This generally indicates greater reactivity of the female immune system. The link between vitamin D deficiency and the change in the incidence of MS in women and men over the years has already been discussed in the section on the nervous system. For other autoimmune diseases, an association between oestrogen level, the course of the disease and the vitamin D content of the serum was also observed, although the role that vitamin D plays in the course of these diseases is less well established(Reference Dupuis, Pagano and Pierdominici145). Undoubtedly, vitamin D deficiency is a risk factor in autoimmune diseases, but further studies are needed to assess the beneficial role of vitamin D supplementation in therapy for males and females with particular disorders.
Conclusion
Vitamin D has been the subject of several research studies over the years. Nonetheless, due to the large number of factors modulating its levels in organisms (Fig. 2), these studies seem to be insufficient. Our article is a narrative, not a systematic review, which may be a limitation. However, it indicates the need for comprehensive intervention studies to establish the real impact of vitamin D on individual diseases. Future research should take into account both sexes and possible differences in the responses of females and males, as well as inter-individual differences in vitamin D metabolism. Particularly at this time of dynamic progress in personalised medicine, differences in physiological processes between the patients require special attention. A better understanding of differences in the levels and effects of vitamin D in females and males may lead to sex-specific dietary recommendations.

Fig. 2. The difference in the production of sex hormones is the most significant relationship leading to the conclusion that vitamin D metabolism is sex-dependent. Sex hormones characteristic of females stimulate vitamin D metabolism, whereas testosterone characteristic of males inhibits vitamin D metabolism. Studies show that males are characterised by lower levels of vitamin D precursors (7-dehydrocholesterol), lower levels of vitamin D transporting protein (DBP), lower levels of body fat and a higher expression of the vitamin D catabolising enzyme (CYP24A1). On the other hand, females are characterised by higher levels of self-reactive T cells and higher levels of vitamin D-activating enzyme (CYP27B1) and vitamin D receptor (VDR). (Figure made with BioRender).
The 2020–2021 quarantine, covering a large part of the world’s population, may undoubtedly contribute to an increase in the number of people suffering from vitamin D deficiency(Reference Grant, Lahore and McDonnell146). Future epidemiological studies are likely to present even more worrying results as an effect of the SARS-CoV-2 pandemic. Further, in light of the current global situation, knowledge already acquired about the effects of vitamin D should be disseminated to improve the general health of the population.
Acknowledgements
The study was supported by the National Research Institute of Animal Production in Balice.
The manuscript was prepared 70 % by A. W. and 30 % by M. O.
There are no conflicts of interest.