Coenzyme Q10 (CoQ10), also known as ubiquinone, is a lipid-soluble, vitamin-like substance located in the hydrophobic interior of the phospholipid bilayer of the cellular membrane. CoQ10 increases mitochondrial activity related to the synthesis of ATP(Reference Turunen, Olsson and Dallner1). In addition, CoQ10 acts as an antioxidant in both the mitochondria and lipid membranes by scavenging reactive oxygen species (ROS), either directly or in conjunction with α-tocopherol(Reference Ernster and Dallner2–Reference Lass and Sohal5). This antioxidant activity appears only with the reduced form (ubiquinol). The oxidized form (ubiquinone) is readily reduced to ubiquinol enzymically after dietary uptake(Reference Mohr, Bowry and Stocker6). Although CoQ10 is present in meat and fish, its content in such foods is very low(Reference Weber, Bysted and Holmer7). Therefore, synthetic CoQ10 is used as a dietary supplement by both health-conscious individuals and those with ailments because of its important biological roles, such as mitochondrial energy metabolism and antioxidant activity(Reference Hosoe, Kitano, Kishida, Kubo, Fujii and Kitahara8).
Aerobic energy production generates ROS in muscle cells, and the amount of ROS increases approximately 10- to 20-fold during physical exercise(Reference Sjodin, Hellsten Westing and Apple9). Evidence exists to suggest that ROS induce muscular injury(Reference McArdle, van der Meulen, Catapano, Symons, Faulkner and Jackson10–Reference Finaud, Lac and Filaire12) with a subsequent decrease in physical performance(Reference Radak, Pucsok, Mecseki, Csont and Ferdinandy13). Recent research has suggested that supplementation with certain antioxidants is practical for physically active individuals to hasten recovery from fatigue and to prevent exercise damage(Reference Banerjee, Mandal, Chanda and Chakraborti14). Supplementations with other antioxidant nutrients, such as vitamin C and vitamin E, can prevent exercise-induced oxidative damage in human subjects and rats(Reference Kumar, Reddy, Prasad, Thyagaraju and Reddanna15, Reference Mastaloudis, Morrow, Hopkins, Devaraj and Traber16). However, little is known about the effect of CoQ10 supplementation on muscular injury and oxidative stress resulting from strenuous exercise in human subjects.
Shimomura et al. (Reference Shimomura, Suzuki, Sugiyama, Hanaki and Ozawa17) reported that intravenous CoQ10 supplementation attenuates the rise in markers of muscle damage in rats following downhill running. In addition, Okamoto et al. (Reference Okamoto, Kubota, Takahata, Takahashi, Goshima and Kishi18) provided evidence that CoQ10 protects cultured skeletal muscle cells from electrical stimulation-induced lactate dehydrogenase release. From these experimental results, CoQ10 supplementation may have the potential to reduce exercise-induced muscular cell damage and oxidative stress in human individuals.
Kendo is a traditional Japanese sport and involves duelling between two people who are each equipped with protective armour and a sword-like stave made of bamboo. A match may last up to 5 min and the winner is the first to score the second of a maximum three points. Points are scored by inflicting blows to the head, torso, forearm or throat. Some previous studies including our own have shown that kendo exercise in training camp is a highly intense exercise that causes an increase of oxidative stress and cellular damage(Reference Imai, Hayashi, Negawa, Nakamura, Tomida, Koda, Tajima, Koda, Suda and Era19–Reference Kon, Nakamura, Natsui, Kimura, Lee, Akama and Kono21). The purpose of the present study, then, was to examine the effect of CoQ10 supplementation on the exercise-induced muscular injury and oxidative stress of collegiate kendo athletes during training camp. We hypothesized that CoQ10 supplementation would reduce exercise-induced muscular damage and oxidative stress. To test this hypothesis, we investigated the time course changes of muscular damage and oxidative stress markers in kendo athletes during training camp.
Materials and methods
Subjects
Eighteen male students of the University of Tsukuba, all elite Japanese kendo athletes, participated in a 6 d training camp from 25 to 30 March 2006. The characteristics of the subjects are shown in Table 1. All subjects completed a medical and supplementation history questionnaire so we could determine their eligibility for the study. No subjects used anti-inflammatory drugs or dietary supplements during this study. The experimental procedure was approved by the Human Research Ethics Committee of Tsukuba University and was explained to the subjects before they signed informed consent forms.
Table 1 Characteristics of the subjects*
(Mean values and standard deviations)

CoQ10, coenzyme Q10.
* For details of subjects and procedures, see Materials and methods.
Treatment
All subjects were randomly assigned to either a CoQ10 supplemented group (n 10) or a placebo supplemented group (n 8) in a double-blind manner. Subjects in the CoQ10 group took three Kaneka CoQ10 100 mg capsules once per d, in the morning after breakfast, from 14 d before the training camp started until it ended. Subjects in the placebo group consumed three placebo capsules per d for the same duration. Both the CoQ10 and placebo were identical in appearance. Also, all subjects ate the same diet during the training camp.
Composition of Kaneka Q10 capsules
The CoQ10 capsules used in the present study were provided by the Kaneka Corporation (Osaka, Japan). Each capsule contained 100 mg Kaneka CoQ10, 0·4 mg lecithin SLP-Paste NGS (Tsuji Oil Mill, Mie, Japan), 134·67 mg safflower oil (Nisshin Oillio Group, Tokyo, Japan), 0·6 mg Poem S-100V (Riken Vitamin, Tokyo, Japan) and 4·33 mg yellow beeswax (Miki Chemical Industry, Hyogo, Japan). The placebo capsules were the same, except they contained safflower oil instead of CoQ10.
Exercise protocol
During the camp, there were two separate training sessions on each day: 2·5 h (09.00–11.30 hours) in the morning and 3 h (14.30–17.30 hours) in the afternoon. There was no morning session on the first day of the training camp. Morning practices consisted of 20 min warming-up, 40 min kihon-keiko (practising to acquire the basic movements), a 10 min break, 60 min gokaku-keiko (keiko practised by persons of almost equal skill), 15 min kakari-keiko (the keiko method in which, for a short time period, the trainee practises striking the motodachi, the person acting as instructor, with all learned waza techniques without thinking of being struck or of dodging strikes) and 5 min cooling down. Afternoon practices consisted of 20 min warming-up, 100 min shiai-keiko (a method of keiko performed in the presence of referees, as in a match), a 10 min break, 45 min gokaku-keiko and 5 min cooling down. The VO2max percentages were approximately 40 for kihon-keiko, approximately 55 for gokaku-keiko and approximately 70 for kakari-keiko. The VO2max percentage for shiai-keiko at its maximum value was also approximately 70(Reference Imai, Hayashi, Negawa, Nakamura, Tomida, Koda, Tajima, Koda, Suda and Era19).
Blood sampling
A 20 ml venous blood sample was obtained from each athlete's forearm in a resting condition between 13.30 and 14.15 hours every afternoon: 2 weeks before the training camp (pre), first day (1 d), third day (3 d), fifth day (5 d) and 1 week after the camp (post). Serum was separated from blood cells by centrifugation (3000 rpm for 10 min) and stored at − 30°C until analysis. Serum volume was adjusted according to Dill and Costill's equation(Reference Dill and Costill22).
Biochemical analysis
Creatine kinase (CK) activity in the serum, as a marker of muscle damage, was measured by using a commercial kit (Kanto Chemical Co., Tokyo, Japan). Another marker of muscle damage, serum myoglobin (Mb) concentration, was determined by using a commercial kit (Eiken Chemical Co., Tokyo, Japan). Counts of leucocytes (WBC), neutrophil cells and monocyte cells were obtained using an automated cell counter (SE-9000; Sysmex, Kobe, Japan). Serum lipid peroxide (LPO), which is an index of oxidative stress concentration, was determined by using a commercial kit (Kyowa Medex Co., Tokyo, Japan). Serum concentration of CoQ10 was measured by HPLC, a method described in Ikematsu et al. (Reference Ikematsu, Nakamura, Harashima, Fujii and Fukutomi23).
Electron spin resonance measurement of scavenging activity against superoxide
Scavenging activity against superoxide was measured using the method described by Tanabe et al. (Reference Tanabe, Masuda, Hirayama, Nagase, Kono and Kuno24). The serum scavenging activity against superoxide anions derived from the xanthine oxidase–hypoxanthine reaction was determined by calculating the inhibition rate of electron spin resonance (ESR) (JES-TE25X; JEOL, Tokyo, Japan) signals in a mixture of serum and a superoxide-generating system. For measuring the scavenging activity against superoxide the reaction mixture consisted of 50 μl serum, 5·5 mm-hypoxanthine (6-hydroxypurine), 0·4 U/ml xanthine oxidase and 15 μl 9·2 m-5,5-dimethyl-1-pyrroline-N-oxide as a spin trap agent. The ESR spectra were recorded 45 s after xanthine was added at room temperature. The blank spectrum was considered as a control and the standard curve of superoxide dismutase activity was constructed based on the spectra with 6·25, 12·5, 25 and 50 U/ml superoxide dismutase(Reference Tanabe, Masuda, Hirayama, Nagase, Kono and Kuno24). Signal intensity was expressed as a ratio of the peak located at the lowest magnetic field of the four-line 5,5-dimethyl-1-pyrroline-N-oxide-superoxide adduct signal to the signal intensity of internal standard Mn2+. Scavenging activity was calculated as SOD activity based on the standard curve(Reference Tanabe, Masuda, Hirayama, Nagase, Kono and Kuno24).
Statistics
All data were analysed by a two-way ANOVA with repeated measures using StatView 5.0 (Hulinks, Tokyo, Japan). If significant differences existed, a posthoc analysis test (Bonferroni/Dunn) was performed. The percent changes between the groups were compared using unpaired t tests. The level of statistical significance was set at P < 0·05.
Results
Weight and body fat
Weight and body fat remained unchanged in both the CoQ10 and placebo groups over the training period (data not shown).
Serum coenzyme Q10 concentration
Fig. 1 shows serum CoQ10 concentration data before (pre) and during (1 d and 5 d) the training camp. In the CoQ10 group, serum CoQ10 concentration significantly increased (P < 0·01) in 2 weeks (from pre to 1 d). On the other hand, serum CoQ10 concentration in the placebo group did not change from pre to 5 d. Percent changes in serum CoQ10 concentration in the CoQ10 group were higher than those of the placebo group at 1 d and 5 d (P < 0·01). Therefore, our finding was that oral supplementation with CoQ10 for 2 weeks significantly increases serum CoQ10 level.

Fig. 1 Serum coenzyme Q10 (CoQ10) concentration before (pre) and during (1 d and 5 d) training camp (–♦–, CoQ10; –○–, placebo). Values are means and standard deviations. Mean values were significantly different from pre: **P < 0·01. Mean values were significantly different between CoQ10 and placebo groups: ††P < 0·01.
Serum creatine kinase activity
Fig. 2 shows serum CK activity data before (pre), during (1 d, 3 d, 5 d) and after (post) training camp. In both the CoQ10 and placebo groups, serum CK activity significantly increased at 3 d and 5 d compared with pre (P < 0·01). Percent changes in serum CK activity in the CoQ10 group were significantly lower than those of the placebo group at 3 d (P < 0·05). This result suggests that supplementation of CoQ10 reduced exercise-induced muscular injury in athletes.

Fig. 2 Serum creatine kinase (CK) activity before (pre), during (1 d, 3 d, 5 d), and after (post) training camp (–♦–, coenzyme Q10; –○–, placebo). Values are means and standard deviations. Mean values were significantly different from pre: **P < 0·01. Mean values were significantly different between coenzyme Q10 and placebo groups: †P < 0·05.
Serum myoglobin concentration
Fig. 3 shows serum Mb concentration data before (pre), during (1 d, 3 d, 5 d) and after (post) training camp. In both the CoQ10 and placebo groups, serum Mb concentration significantly increased at 3 d and 5 d compared with pre (P < 0·01). Percent changes of serum Mb concentration in the CoQ10 group were significantly lower than those of the placebo group at 3 d (P < 0·05). This data is further evidence that CoQ10 reduced exercise-induced muscular injury.

Fig. 3 Serum myoglobin (Mb) concentration before (pre), during (1 d, 3 d, 5 d) and after (post) training camp (–♦–, coenzyme Q10; –○–, placebo). Values are means and standard deviations. Mean values were significantly different from pre: **P < 0·01. Mean values were significantly different between coenzyme Q10 and placebo groups: †P < 0·05.
Serum lipid peroxide concentration
Fig. 4 shows serum LPO concentration data before (pre), during (1 d, 3 d, 5 d) and after (post) training camp. LPO concentration did not change in either group. Percent changes of serum LPO concentration in the CoQ10 group were lower than those of the placebo group at 3 d and 5 d (P < 0·05). Therefore, in the present study, the effect of CoQ10 supplementation on exercise-induced oxidative stress in athletes was unclear.
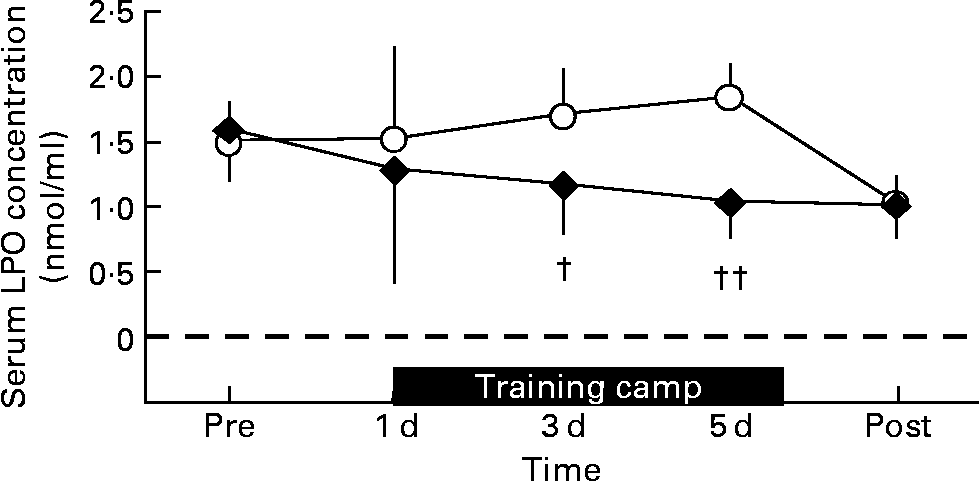
Fig. 4 Serum lipid peroxide (LPO) concentration before (pre), during (1 d, 3 d, 5 d) and after (post) training camp (–♦–, coenzyme Q10; –○–, placebo). Values are means and standard deviations. Mean values were significantly differences between coenzyme Q10 and placebo groups: †P < 0·05; ††P < 0·01.
Leucocytes, neutrophil cells and monocyte cells
Table 2 shows WBC, neutrophil and monocyte data before (pre), during (1 d, 3 d, 5 d) and after (post) training camp. In the placebo group, the WBC count significantly increased at 3 d compared with pre (P < 0·01). In contrast, WBC did not change in the CoQ10 group. The neutrophil count in both groups also significantly increased at 3 d and 5 d compared with pre (P < 0·05), whereas monocyte count did not change in either group. WBC, neutrophil and monocyte counts did not differ significantly between the CoQ10 and placebo groups. Therefore, these results suggested that oral supplementation with CoQ10 has no effect on the changes of WBC, neutrophil and monocyte.
Table 2 Changes of leucocyte (WBC), neutrophil and monocyte count before (pre), during (1 d, 3 d, 5 d) and after (post) training camp†
(Mean values and standard deviations)

CoQ10, coenzyme Q10.
Mean values were significantly different from pre: *P < 0·05; **P < 0·01.
† For details of subjects and procedures, see Materials and methods.
Scavenging activity against superoxide anion
Fig. 5 shows serum scavenging activity against superoxide anion data before (pre), during (1 d, 3 d, 5 d) and after (post) training camp. Serum scavenging activity against superoxide anion did not change in either group.

Fig. 5 Scavenging activity against superoxide anion before (pre), during (1 d, 3 d, 5 d) and after (post) training camp (–♦–, coenzyme Q10; –○–, placebo). Values are means and standard deviations.
Discussion
The aim of the present study was to determine the effect of CoQ10 supplementation exercise-induced muscular injury and oxidative stress in kendo athletes during a training camp. The study revealed that CK activity and Mb concentration were lower in the CoQ10 group compared with the placebo group. This finding, which is novel, is important because it indicates that supplementation of CoQ10 is useful for reducing exercise-induced muscle damage in athletes. It has been reported that oral administration of CoQ10 increases plasma and skeletal muscle levels of CoQ10(Reference Dill and Costill22, Reference Kwong, Kamzalov, Rebrin, Bayne, Jana, Morris, Forster and Sohal25). In the present study, after 2 weeks of supplementation, serum concentrations of CoQ10 significantly increased only in the CoQ10 group, and the serum CoQ10 level remained stable during administration (see Fig. 1). CoQ10 supplementation (300 mg/d) for 2 weeks resulted in a 4-fold increase of serum CoQ10 concentration compared with pre level. This result is consistent with that of a previous study(Reference Dill and Costill22).
CK and Mb have been the most commonly used markers of skeletal muscle damage(Reference Koutedakis, Raafat, Sharp, Rosmarin, Beard and Robbins26, Reference Peake, Suzuki, Wilson, Hordern, Nosaka, Mackinnon and Coombes27). They represent a proxy marker of damage to the muscle cell membrane(Reference Koutedakis, Raafat, Sharp, Rosmarin, Beard and Robbins26, Reference Peake, Suzuki, Wilson, Hordern, Nosaka, Mackinnon and Coombes27). In the present study, serum CK activity in the placebo group significantly increased by 5-fold during the training camp (see Fig. 2). Also, in the placebo group, serum Mb concentration significantly increased by 7·7-fold during the training camp (see Fig. 3). The increases of serum CK and Mb in the present study indicated that kendo training camp causes muscular injury. CK and Mb are indirect markers of muscle damage. Further work involving more direct measures of muscle damage (e.g. electron micrographs) is necessary.
Other studies have investigated the effect of CoQ10 supplementation on exercise-induced muscle damage in both rats(Reference Shimomura, Suzuki, Sugiyama, Hanaki and Ozawa17) and in human subjects(Reference Kaikkonen, Kosonen, Nyyssonen, Porkkala-Sarataho, Salonen, Korpela and Salonen28). CoQ10 supplementation attenuates CK activity following downhill running in rats(Reference Shimomura, Suzuki, Sugiyama, Hanaki and Ozawa17), but not in human subjects following a marathon run(Reference Kaikkonen, Kosonen, Nyyssonen, Porkkala-Sarataho, Salonen, Korpela and Salonen28). The difference in results between the previous human study(Reference Kaikkonen, Kosonen, Nyyssonen, Porkkala-Sarataho, Salonen, Korpela and Salonen28) and the present study may be attributable to the intake of CoQ10. In the previous study by Kaikkonen et al. (Reference Kaikkonen, Kosonen, Nyyssonen, Porkkala-Sarataho, Salonen, Korpela and Salonen28), the intake of CoQ10 was 90 mg daily. On the other hand, the intake of CoQ10 in the current study was 300 mg daily. Therefore, there is a possibility that exercise-induced muscular injury was not reduced because the intake of CoQ10 necessary to increase the tissue (muscle) CoQ10 concentrations was low in the previous human study. CoQ10 stabilizes the structure of cell membrane phospholipids(Reference Kambara, Takagi, Satake, Sugiyama and Ozawa29, Reference Nagai, Miyazaki, Ogawa, Satake, Sugiyama and Ozawa30) and protects cultured skeletal muscle cells from electrical stimulation-induced muscular cell injury(Reference Okamoto, Kubota, Takahata, Takahashi, Goshima and Kishi18). Bello et al. (Reference Bello, Gomez-Diaz, Buron, Alcain, Navas and Villalba31) have reported that oral supplementation with CoQ10 significantly increases the CoQ10 count of cell membranes. Therefore, CoQ10 supplementation may reduce exercise-induced muscular injury by raising CoQ10 concentration in muscle cell membranes and stabilizing the cell membrane.
Jackson et al. (Reference Jackson, Edwards and Symons32) indicated that muscle damage is associated with an increase in muscle ROS generation. After exercise leading to muscle damage, inflammatory cells, mainly neutrophils and macrophages, infiltrate damaged skeletal muscle and initiate phagocytosis of injured tissue via their arsenal of ROS(Reference Moncada and Higgs33). They sometimes even release ROS into healthy bystander tissues(Reference Cheeseman and Slater34). Therefore, ROS released from inflammatory cells may cause oxidative damage to muscle cell membranes(Reference Ji35). Because CoQ10 is located in membranes in close proximity to unsaturated lipid chains, it acts as a primary scavenger of ROS(Reference Close, Ashton, Cable, Doran, Noyes, McArdle and MacLaren36) and prevents lipid peroxidation(Reference Turunen, Olsson and Dallner1). In the present study, serum LPO concentration did not change in either group although LPO in the CoQ10 group was lower than in the placebo group (see Fig. 4). Therefore, the effect of CoQ10 supplementation on exercise-induced oxidative stress in athletes was unclear in the current study. CoQ10 may have a greater effect on oxidative stress within skeletal muscle. Other data indicate a possible relationship between infiltration of inflammatory cells and oxidative stress in response to contraction-induced muscle injury(Reference Kon, Tanabe, Lee, Kimura, Akimoto and Kono37). Thus, future research could address the effect of CoQ10 supplementation on oxidative damage within skeletal muscle.
ROS produced by neutrophils contribute to muscle damage and circulating neutrophils increase after exercise leading to muscle damage(Reference Peake, Suzuki, Wilson, Hordern, Nosaka, Mackinnon and Coombes27, Reference Close, Ashton, Cable, Doran, Noyes, McArdle and MacLaren36). However, the influence of oral CoQ10 supplementation on the changes in neutrophil counts after exercise leading to muscle damage is currently unknown. The present study indicates that CoQ10 supplementation had no influence on changes in neutrophil counts after exercise resulting in muscle damage. Other research has reported that CoQ10 treatment attenuated neutrophil oxidative activity(Reference Dlugosz, Kuzniar, Sawicka, Marchewka, Lembas-Bogaczyk, Sajewicz and Boratynska38). Therefore, future studies could examine whether CoQ10 supplementation scavenges the ROS produced by neutrophils that have migrated to the site of injury in skeletal muscle.
The effect of CoQ10 supplementation on antioxidant capacity in serum was determined by ESR with a spin-trapping technique in the present study. Since the superoxide anion scavenging activity in both groups did not change during training camp (see Fig. 5), it is difficult to assess the effect of CoQ10 on scavenging activity. In the present study, the superoxide anion scavenging activity was measured in blood and not in skeletal muscle. Tanabe et al. (Reference Tanabe, Masuda, Hirayama, Nagase, Kono and Kuno24) demonstrated that exercise training increases the superoxide anion scavenging activity in skeletal muscle, as determined by ESR with a spin-trapping technique. In addition, Zhou et al. (Reference Zhou, Zhi, Tang, Yu and Han39) showed that CoQ10 can directly scavenge hydroxyl radicals from the Fenton reaction, but not superoxide anion radicals from the xanthine/xanthine oxidase system. Hydroxyl radicals have the potential to react with and damage most cellular targets including lipids, proteins and DNA. Hydroxyl radicals are not eliminated by antioxidant enzymes in the cell, but by non-enzymic antioxidants such as CoQ10. Future investigations might consider examining the effect of CoQ10 supplementation on scavenging activity against superoxide anion and the production of hydroxyl radicals in skeletal muscle.
As outlined earlier, blood markers of muscle injury and oxidative stress do not necessarily reflect what events are occurring locally within skeletal muscle during exercise. Because the subjects in the present study were athletes, it was difficult to obtain skeletal muscle samples. A detailed study using human skeletal muscles and/or animal studies are needed in future research. The present study is valid as an initial step to determine the effects of CoQ10 supplementation on exercise-induced muscular injury in athletes.
Conclusion
In summary, we showed that supplementation of CoQ10 reduced serum CK activity and Mb concentration in collegiate kendo athletes during training camp. Our data indicated that muscular injury in these collegiate athletes was attenuated by CoQ10 supplementation. Thus, the present results support the notion that CoQ10 supplementation is useful for reducing muscular injury in athletes.
Acknowledgements
We thank Kaneka Corp. for their generous gift of CoQ10. We also thank R. DiGovanni (Waseda University, Japan) and Jonathan Peake (University of Queensland, Australia) for careful review of the manuscript. None of the authors had a conflict of interest.