The balance between aggressive and defensive factors determines whether gastric lesions develop, with either a relative increase in aggressive factors or a relative decrease in defensive factors resulting in lesions. The gastric mucosa is challenged by a variety of both endogenous and exogenous irritants (aggressive factors), including ethanol (EtOH), gastric acid, pepsin, reactive oxygen species and non-steroidal anti-inflammatory drugs(Reference Holzer1). In order to protect the gastric mucosa against these aggressive factors, a complex defence system, which includes the production of surface mucus (gastric mucin) and bicarbonate and the regulation of gastric mucosal blood flow, has evolved. PG, in particular PGE2, enhance these protective mechanisms, and are therefore thought to be a major gastric mucosal defensive factor(Reference Miller2).
Recently, heat shock proteins (HSP) have also attracted considerable attention as another important defensive factor. When cells are exposed to stressors, HSP are induced in a manner that is dependent on the transcription factor heat shock factor 1 (HSF1), and this cellular up-regulation, in particular the up-regulation of HSP70, provides resistance to such stressors(Reference Mathew and Morimoto3–Reference Hirakawa, Rokutan and Nikawa6). We have recently reported that HSF1-null mice or transgenic mice expressing HSP70 show sensitive or resistant phenotypes, respectively, to irritant-induced gastric lesions(Reference Tanaka, Tsutsumi and Arai7, Reference Suemasu, Tanaka and Namba8), suggesting that HSP, especially HSP70, play an important role in the protection of the gastric mucosa against irritant-induced gastric lesions.
Chemicals that decrease the level of aggressive factors or increase the level of defensive factors are beneficial for protecting the gastric mucosa against the formation of irritant-induced lesions. Acid-control drugs, such as histamine-2 receptor antagonists and proton pump inhibitors, and anti-Helicobacter pylori drugs belong to the former group, while drugs that induce the production of gastric mucins, PG and HSP belong to the latter. These chemicals (anti-ulcer drugs) decrease the incidence of surgery for the treatment of gastric ulcers, resulting in a good quality of life for patients with this disease. However, in order to decrease healthcare costs and to prevent the development of gastric ulcers, it is important to find health foods and supplements (complementary and alternative medicines) that can reduce the level of aggressive factors or increase the level of defensive factors.
β-Glucans are naturally occurring polysaccharides found in the cell walls of yeast, fungi, cereal plants and certain bacteria(Reference Tsoni and Brown9, Reference Chen and Seviour10). As suggested by the fact that various foods contain β-glucans, they are known to have little toxic and adverse effects(Reference Tsoni and Brown9). β-Glucans from fungi occur as β-(1,3)-linked glucose polymers with β-(1,6) side chains of varying length and distribution(Reference Tsoni and Brown9–Reference Brown and Gordon11). Such β-glucans from mushrooms have been used as anti-tumour drugs in Japan(Reference Chen and Seviour10). They are thought to achieve anti-tumour effects through their immunoactivating activities, such as stimulating the release of cytokines, NO and arachidonic acid metabolites(Reference Chen and Seviour10–Reference Kimura, Sumiyoshi and Suzuki14). In addition, β-(1,3)-d-glucans with β-(1,6) branches have been reported to have various other beneficial effects, such as enhancing defence against bacterial, viral, fungal and parasitic challenge, increasing haematopoiesis and radioprotection, stimulating the wound-healing response, and decreasing the levels of serum lipids(Reference Tsoni and Brown9, Reference Chen and Seviour10, Reference Berdal, Appelbom and Eikrem15, Reference Bell, Goldman and Bistrian16). Interestingly, it has recently been reported that β-glucans suppress inflammatory responses in some animal models by decreasing the levels of pro-inflammatory cytokines, chemokines and cell adhesion molecules (CAM)(Reference Sener, Eksioglu-Demiralp and Cetiner17–Reference Shah, Williams and Keshvara22). This suggests that β-glucan is an interesting immunomodulator, causing opposing effects on immune systems. Since the cytoprotective effects of β-glucans and suppression by β-glucans of anti-tumour drug-induced damage in the small intestine have been reported(Reference Sener, Eksioglu-Demiralp and Cetiner17, Reference Sumiyoshi, Suzuki and Kimura23, Reference Ha, Hua and Grant24), it is possible that β-glucans protect against the formation of irritant-induced gastric lesions. However, no studies have examined this possibility to date.
We have used β-(1,3)-d-glucan with β-(1,6) branches isolated from Aureobasidium pullulans (Reference Kimura, Sumiyoshi and Suzuki14, Reference Kimura, Sumiyoshi and Suzuki25). In general, β-(1,3)-d-glucans with β-(1,6) branches have a high molecular weight (over 2000 kDa), high viscosity and low water solubility. In addition, such β-glucans easily form gels containing high-order structures of single or triplet spirals. Therefore, its purification is extremely difficult and, in previous studies, crude β-glucan fractions rather than purified ones have been used in experiments(Reference Tsoni and Brown9). We succeeded in the purification and industrial-scale production of low-molecular-weight β-(1,3 → 1,6)-d-glucan (LMW β-glucan) from the A. pullulans GM-NH-1A1 strain (black yeast, a mutant strain K-1)(Reference Kimura, Sumiyoshi and Suzuki14). The characteristic features of this β-glucan are its LMW (about 100 kDa), low viscosity, high water solubility and high level of β-(1-6) branching (50–80 %)(Reference Kimura, Sumiyoshi and Suzuki14, Reference Kimura, Sumiyoshi and Suzuki25). We have previously reported that LMW β-glucan has various clinically beneficial effects, such as suppression of the allergic response by increasing the levels of IL-12 and interferon-γ; suppression of restraint stress-induced immunosuppression (such as suppression of natural killer cell activity and IL-12 and IL-6 production); anti-tumour and anti-metastatic actions mediated via stimulation of the immune system in the small intestine; and protective effects against anti-tumour drug-induced damage in the small intestine(Reference Kimura, Sumiyoshi and Suzuki14, Reference Sumiyoshi, Suzuki and Kimura23, Reference Kimura, Sumiyoshi and Suzuki25, Reference Kimura, Sumiyoshi and Suzuki26). In the present study, we found that LMW β-glucan protects the gastric mucosa of mice against the formation of irritant-induced lesions. We also suggest that LMW β-glucan achieves this gastroprotective effect by increasing the levels of defensive factors, such as HSP70 and gastric mucin.
Materials and methods
Chemicals and animals
LMW β-glucan was prepared from the conditioned culture medium of A. pullulans GM-NH-1A1, as described previously(Reference Kimura, Sumiyoshi and Suzuki14, Reference Kimura, Sumiyoshi and Suzuki25). Analysis of 1H and 13C NMR spectra and gel-filtration chromatography revealed that LMW β-glucan has approximately 70 % β-(1-6) branches and an average molecular weight of 100 kDa, as described previously(Reference Kimura, Sumiyoshi and Suzuki14, Reference Kimura, Sumiyoshi and Suzuki25). Lipopolysaccharide, U0126 (an inhibitor of extracellular signal-regulated kinase), paraformaldehyde, peroxidase standard, fetal bovine serum, o-dianisidine and hexadecyl trimethyl ammonium bromide were obtained from Sigma (St Louis, MO, USA). α-(1,4 → 1,6)-d-Glucan (pullulan, molecular weight 50 000–100 000) was obtained from WAKO Pure Chemicals (Tokyo, Japan). Terminal deoxynucleotidyl transferase was obtained from Toyobo (Osaka, Japan). SB203580 (an inhibitor of p38 mitogen-activated protein kinase (p38)), SP600125 (an inhibitor of c-jun NH2-terminal kinase) and the ELISA kit for detection of PGE2 were from Cayman Chemicals (Ann Arbor, MI, USA). Biotin 14-ATP and Alexa Fluor 488 conjugated with streptavidin were purchased from Invitrogen (Carlsbad, CA, USA). Mounting medium for immunohistochemical analysis (VECTASHIELD) was from Vector Laboratories (Burlingame, CA, USA). The RNeasy kit was obtained from Qiagen (Valencia, CA, USA), the PrimeScript® 1st strand cDNA Synthesis Kit was from TAKARA Bio (Ohtsu, Japan) and the iQ SYBR Green Supermix was from Bio-Rad (Hercules, CA, USA). Mayer's haematoxylin, 1 % eosin alcohol solution, cold Schiff's reagent, sulphate solution, 1 % periodic acid solution and mounting medium for histological examination (malinol) were from MUTO Pure Chemicals (Tokyo, Japan). The compound 4,6-diamino-2-phenylindole was from Dojindo (Kumamoto, Japan). Antibodies against HSP70 (for immunohistochemical analysis) and dectin-1 were from R&D Systems (Minneapolis, MN, USA). An antibody against HSP70 (for immunoblotting analysis) was from Stressgen (Ann Arbor, MI, USA), while one against HSF1 was kindly provided by Dr Akira Nakai (Yamaguchi University, Yoshida, Japan).
Wild-type ICR mice (6–8 weeks old, male) were used. The experiments and procedures described here were carried out in accordance with the Guide for the Care and Use of Laboratory Animals as adopted and promulgated by the National Institute of Health, and were approved by the Animal Care Committee of Kumamoto University (Kumamoto, Japan).
Gastric damage assay
Gastric ulcerogenic response was examined as described previously(Reference Tanaka, Tsutsumi and Arai7). Mice, which had been fasted for 18 h, were orally administered either EtOH or HCl (5 ml/kg). After 4 h, the animals were killed with an overdose of diethyl ether, after which their stomachs were removed and scored for haemorrhagic damage by an observer unaware of the treatment they had received. Calculation of the scores involved measuring the area of all lesions in millimetres squared and summing the values to give an overall gastric lesion index. Gastric mucosal PGE2 levels were determined by ELISA, as described previously(Reference Futaki, Arai and Hamasaka27).
Histopathological and immunohistochemical analyses and terminal deoxynucleotidyl transferase dUTP nick-end labelling assay
Gastric tissue samples were fixed in 4 % buffered paraformaldehyde, and then embedded in paraffin before being cut into 4 μm-thick sections.
For histopathological examination, sections were stained first with Mayer's haematoxylin and then with 1 % eosin alcohol solution (haematoxylin and eosin staining). Samples were mounted with malinol and inspected with the aid of an Olympus BX51 microscope (Tokyo, Japan).
For immunohistochemical analysis, sections were incubated with 0·3 % H2O2 in methanol for the removal of endogenous peroxidase. Sections were blocked with 2·5 % goat serum for 10 min, incubated for 12 h with an antibody against HSP70 (1:40 dilution) in the presence of 2·5 % bovine serum albumin and then incubated for 2 h with a peroxidase-labelled polymer conjugated to goat anti-mouse Ig. Then, 3,3′-diaminobenzidine was applied to the sections, and the sections were finally incubated with Mayer's haematoxylin. Samples were mounted with malinol and inspected using a fluorescence microscope (Olympus BX51).
For the terminal deoxynucleotidyl transferase dUTP nick-end labelling assay, sections were incubated first with proteinase K (20 μg/ml) for 15 min at 37°C, then with TdTase and biotin 14-ATP for 1 h at 37°C and finally with Alexa Fluor 488 conjugated with streptavidin and 4,6-diamino-2-phenylindole for 2 h. Samples were mounted with VECTASHIELD and inspected with the aid of a fluorescence microscope (Olympus BX51).
Periodic acid Schiff staining
Gastric tissue samples were fixed in Carnoy's fluid (EtOH–acetic anhydride 3:1), and embedded in paraffin before being cut into 4 μm-thick sections. Sections were incubated with 1 % periodic acid solution for 10 min, then with cold Schiff's reagent for 15 min and finally with sulphate solution for 2 min. Sections were incubated with Mayer's haematoxylin, mounted with malinol and inspected using a fluorescence microscope (Olympus BX51).
Real-time RT-PCR analysis
Real-time RT-PCR was performed as described previously(Reference Mima, Tsutsumi and Ushijima28), with some modifications. Total RNA was extracted using an RNeasy kit according to the manufacturer's protocol. Samples (2·5 μg RNA) were reverse-transcribed using the PrimeScript® 1st strand cDNA Synthesis Kit. Synthesised complementary DNA was used in real-time RT-PCR (Chromo 4 instrument; Bio-Rad) experiments using the iQ SYBR GREEN Supermix, and analysed with Opticon Monitor Software (Bio-Rad). Specificity was confirmed by electrophoretic analysis of the reaction products and by inclusion of template- or RT-free controls. To normalise the amount of total RNA present in each reaction, gapdh or actin complementary DNA was used as an internal standard.
Primers were designed using the Primer3 website. The primers used were as follows (name: forward primer, reverse primer). For humans, muc-1: 5′-acacaaacccagcagtggc-3′, 5′-actcagctcagcggcgac-3′; muc-5ac: 5′-cagccacgtccccttcaata-3′, 5′-accgcatttgggcatcc-3′; actin: 5′-ggacttcgagcaagagatgg-3′, 5′-agcactgtgttggcgtacag-3′. For mice, tnf-α: 5′-cgtcagccgatttgctatct-3′, 5′-cggactccgcaaagtctaag-3′; il-1β: 5′-gatcccaagcaatacccaaa-3′, 5′-ggggaactctgcagactcaa-3′; il-6: 5′-ctggagtcacagaaggagtgg-3′, 5′-ggtttgccgagtagatctcaa-3′; macrophage inflammatory protein (mip)-2α: 5′-accctgccaagggttgacttc-3′, 5′-ggcacatcaggtacgatccag-3′; monocyte chemoattractant protein (mcp)-1: 5′-ctcacctgctgctactcattc-3′, 5′-gcttgaggtggttgtggaaaa-3′; vascular cell adhesion molecule (vcam)-1: 5′-ctcctgcacttgtggaaatg-3′, 5′-tgtacgagccatccacagac-3′; intercellular adhesion molecule (icam)-1: 5′-tcgtgatggcagcctcttat-3′, 5′-gggcttgtcccttgagtttt-3′; muc-1: 5′-gccttcagtgccaagtcaat-3′, 5′-gaaggagaccccaacagaca-3′; muc-5ac: 5′-aaagacaccagtagtcactcagcaa-3′, 5′-ctgggaagtcagtgtcaaacca-3′; gapdh: 5′-aactttggcattgtggaagg-3′, 5′-acacattgggggtaggaaca-3′.
Myeloperoxidase activity
Myeloperoxidase (MPO) activity in the gastric tissues was measured as described previously(Reference Krawisz, Sharon and Stenson29, Reference Tanaka, Namba and Arai30). Animals were placed under deep diethyl ether anaesthesia and killed. Stomachs were dissected, rinsed with cold saline and cut into small pieces. Samples were homogenised, freeze-thawed and centrifuged. The protein concentrations of the supernatants were determined using the Bradford method(Reference Bradford31). MPO activity was determined in 10 mm-phosphate buffer with 0·5 mm-o-dianidisine, 0·00 005 % (w/v) H2O2 and 20 μg protein. MPO activity was obtained from the slope of the reaction curve, and its specific activity was expressed as the number of H2O2 molecules converted/min per mg protein.
Preparation of mouse peritoneal macrophages
Mouse peritoneal macrophages were prepared as described previously(Reference Salimuddin, Nagasaki and Gotoh32). Mice were given 2 ml of 10 % proteose peptone by intraperitoneal injection and peritoneal cells were harvested 3 d later. The cells were seeded in 60 mm culture dishes. After incubation for 4 h, non-adherent cells were removed and the adherent cells were cultured for use in the experiments. Virtually, all of the adherent cells were macrophages, as described previously(Reference Nagasaki, Gotoh and Takeya33).
Cell culture and immunoblotting analysis
Human gastric carcinoma cells were cultured in RPMI-1640 medium supplemented with 10 % fetal bovine serum, penicillin (100 μg/ml) and streptomycin (100 μg/ml) in a humidified atmosphere of 95 % air with 5 % CO2 at 37°C.
Whole-cell extracts were prepared as described previously(Reference Tsutsumi, Tomisato and Takano34). The protein concentration of the samples was determined by the Bradford method(Reference Bradford31). Samples were applied to polyacrylamide SDS gels and subjected to electrophoresis, and the resultant proteins were immunoblotted with an antibody for HSP70 or actin.
Statistical analysis
All values are expressed as means with their standard errors. Two-way ANOVA followed by the Tukey test or Student's t test for unpaired results was used to evaluate differences between more than three groups or between two groups, respectively. Differences were considered to be significant for values of P < 0·05.
Results
Effect of low-molecular-weight β-(1,3→1,6)-d-glucan on gastric ulcerogenic and inflammatory responses
The effect of oral pre-administration of LMW β-glucan on the development of gastric lesions following oral administration of EtOH was examined in mice. We have previously reported that oral administration of EtOH induces the expression of HSP70 in a HSF1-dependent manner, and this induction is accompanied by the production of gastric lesions(Reference Tanaka, Tsutsumi and Arai7). As shown in Fig. 1(a), intragastric administration of 100 % EtOH resulted in significant gastric lesion formation and oral pre-administration of LMW β-glucan suppressed this production in a dose-dependent manner. Oral administration of LMW β-glucan (200 mg/kg) alone did not produce gastric lesions (Fig. 1(a)). A similar protective effect of LMW β-glucan was observed for HCl-induced gastric lesions (Fig. 1(b)). Therefore, the protective effects of LMW β-glucan do not appear to be mediated in response to a specific stressor, such as EtOH. Histopathological examination by haematoxylin and eosin staining supports the notion that pre-administration of LMW β-glucan protects the gastric mucosa against EtOH-induced damage (Fig. 1(c)). Also, the level of gastric mucosal apoptosis was determined by the terminal deoxynucleotidyl transferase dUTP nick-end labelling assay. An increase in the number of terminal deoxynucleotidyl transferase dUTP nick-end labelling-positive (apoptotic) cells was observed following the administration of EtOH, and this increase was suppressed by pre-administration of LMW β-glucan (Fig. 1(c) and (d)). The results in Fig. 1 suggest that LMW β-glucan protects the gastric mucosa against irritant-induced lesions through the suppression of mucosal cell apoptosis.
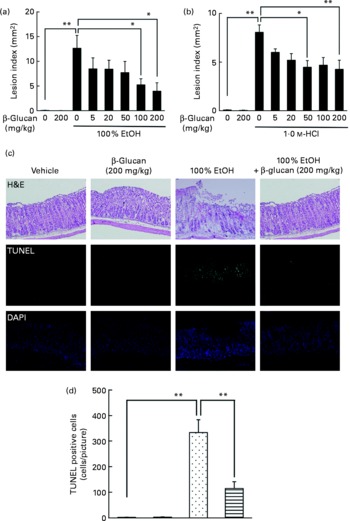
Fig. 1 Effect of low-molecular-weight β-glucan on irritant-induced gastric lesions. Mice were orally administered the indicated doses of β-glucan (mg/kg) or vehicle (□, PBS), 1 h after which they were orally administered (a, c) 100 % ethanol (EtOH) (), (b) 1·0 m-HCl (5 ml/kg) or vehicle (water). (a, b) After 4 h, the stomach was removed and scored for haemorrhagic damage. (c) Sections of gastric tissue were prepared after 4 h and subjected to histopathological examination (haematoxylin and eosin (H&E) staining), terminal deoxynucleotidyl transferase dUTP nick-end labelling (TUNEL) assay and 4,6-diamino-2-phenylindole (DAPI) staining. (d) TUNEL-positive cells in the three sections were counted. Values are means, with their standard errors represented by vertical bars (n 3–17). Mean values were significantly different: * P < 0·05, ** P < 0·01. ■, β-Glucan (200 mg/kg);
, 100 % EtOH+β-glucan (200 mg/kg).
Inflammatory responses, such as the infiltration of leucocytes, play an important role in the production of irritant-induced gastric lesions. In the present study, we examined the effect of LMW β-glucan on the EtOH-induced gastric inflammatory response by measuring gastric MPO activity, an indicator of inflammatory infiltration of neutrophils. As shown in Fig. 2(a), MPO activity increased in response to the administration of EtOH, and this increase was suppressed by pre-administration of LMW β-glucan. The administration of LMW β-glucan alone did not significantly affect the MPO activity (Fig. 2(a)).
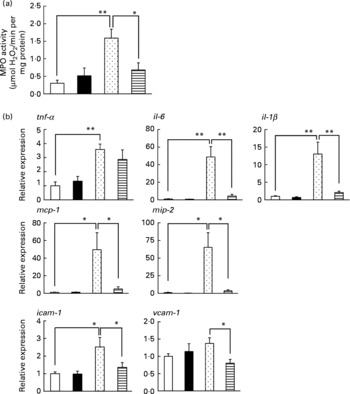
Fig. 2 Effect of low-molecular-weight β-glucan on the ethanol (EtOH)-induced gastric inflammatory response. Mice were orally administered β-glucan (■, 200 mg/kg) or vehicle (□, PBS), 1 h after which they were orally administered 100 % EtOH (, 5 ml/kg) or vehicle (water). (a, b) After 4 h, the stomach was removed. (a) Gastric myeloperoxidase (MPO) activity was determined as described in the Materials and methods. Total RNA was extracted and subjected to real-time RT-PCR using a specific primer set for each gene. (b) Values were normalised to the gapdh gene and expressed relative to the control sample. Values are means, with their standard errors represented by vertical bars (n 9–14). Mean values were significantly different: * P < 0·05, ** P < 0·01.
, 100 % EtOH+β-glucan (200 mg/kg). mcp-1, Monocyte chemoattractant protein-1; mip-2, macrophage inflammatory protein-2; icam-1, intercellular adhesion molecule-1; vcam-1, vascular cell adhesion molecule-1.
We also examined the effects of LMW β-glucan and/or EtOH on the mRNA expression of pro-inflammatory cytokines (IL-6, IL-1β and TNF-α), chemokines (MCP-1 and MIP-2) and CAM (ICAM-1 and VCAM-1) by real-time RT-PCR analysis. As shown in Fig. 2(b), mRNA expression of all these genes except vcam-1 was induced by EtOH administration, and this induction of the expression of all these genes except tnf-α was suppressed by pre-administration of LMW β-glucan. These results suggest that LMW β-glucan suppresses gastric inflammatory responses by suppressing the expression of pro-inflammatory cytokines, chemokines and CAM.
Effect of low-molecular-weight β-(1,3→1,6)-d-glucan on defensive factors for the gastric mucosa
In order to understand the molecular mechanism governing the protective effect of LMW β-glucan on the gastric mucosa, we examined its effect on various gastric mucosal defensive factors, such as HSP70, mucin proteins and PGE2 in vivo. First, we examined the effect of LMW β-glucan and/or EtOH on HSP70 expression at the gastric mucosa by immunoblotting analysis. As shown in Fig. 3(a) and (b), either LMW β-glucan or EtOH significantly induced the expression of HSP70. Immunohistochemical analysis supported the notions that either LMW β-glucan or EtOH induced the expression of HSP70 (Fig. 3(c)).
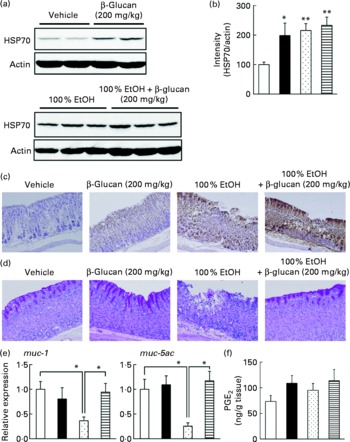
Fig. 3 Effect of low-molecular-weight β-glucan on the defensive factors for the gastric mucosa. Administration of β-glucan (■, 200 mg/kg) and 100 % ethanol (EtOH, ) was performed as described in the legend of Fig. 2. After 4 h, the stomach was removed. (a) Total proteins were analysed by immunoblotting with an antibody against heat shock protein (HSP) 70 or actin. (b) The relative intensity of the HSP70 band to the actin band is shown (one of the gels is shown in (a)). Sections of gastric tissue were prepared and subjected to immunohistochemical analysis with an antibody against (c) HSP70 or (d) periodic acid Schiff staining. (e) The expression of mucin (muc)-1 or muc-5ac mRNA was monitored by real-time RT-PCR, as described in the legend of Fig. 2. (f) The gastric PGE2 level was determined by ELISA. Values are means, with their standard errors represented by vertical bars (n 3–9). Mean values were significantly different: * P < 0·05, ** P < 0·01. □, Vehicle;
, 100 % EtOH+β-glucan (200 mg/kg).
We also examined by periodic acid Schiff staining the effect of LMW β-glucan and/or EtOH on the level of gastric mucin. As shown in Fig. 3(d), the level of gastric mucin was reduced by the administration of EtOH, and pre-administration of LMW β-glucan restored the level. We also examined the mRNA expression of representative gastric mucin genes (muc-1 and muc-5ac)(Reference Corfield, Myerscough and Longman35) by real-time RT-PCR. Muc1 is a membrane-bound protein and Muc-5ac is a secreted protein(Reference Byrd and Bresalier36). As shown in Fig. 3(e), the expression of muc-1 and muc-5ac mRNA was suppressed by the administration of EtOH and pre-administration of LMW β-glucan restored the expression.
The effect of LMW β-glucan and/or EtOH on gastric PGE2 levels was also studied. As shown in Fig. 3(f), neither LMW β-glucan nor EtOH administration affected the level of PGE2. The results in Fig. 3 suggest that LMW β-glucan protects the gastric mucosa against EtOH-induced lesions by increasing the levels of HSP70 and gastric mucin rather than that of PGE2.
Effect of low-molecular-weight β-(1,3→1,6)-d-glucan on the expression of genes in vitro
In order to test whether LMW β-glucan directly affects the expression of HSP70 and mucin proteins, we examined its effect on the expression of these factors in cultured human gastric carcinoma cells. Immunoblotting analysis revealed that treatment of cells with LMW β-glucan significantly induces the expression of HSP70 in a dose-dependent manner (Fig. 4(a) and (b)).
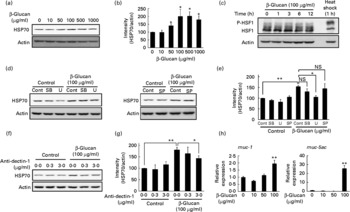
Fig. 4 Effect of low-molecular-weight (LMW) β-glucan on the expression of heat shock protein (HSP) 70 and mucin genes in vitro. (d, f) Human gastric carcinoma cells were pre-incubated for 1 h with or without 10 μm-SB203580 (SB), 10 μm-U0126 (U), 10 μm-SP600125 (SP) or indicated concentrations of an antibody against dectin-1. Cells were incubated with (a, h) the indicated concentrations or (c, d, f) 100 μg/ml of LMW β-glucan for (a, d, f, h) 24 h or (c) indicated periods. (c) Cells were cultured at 42°C for 1 h (heat shock). Total proteins were analysed by immunoblotting with an antibody against HSP70, heat shock factor 1 (HSF1) or actin ((a), (c), (d), (f)) (P-HSF1, phosphorylated form of HSF1). (b, e, g) The relative intensity of the HSP70 band to the actin band is shown (one of the gels is shown in (a, d, f)). The expression of mucin (muc)-1 or muc-5ac mRNA was monitored by real-time RT-PCR, as described in the legend of Fig. 2. (h) Values were normalised to the actin gene. Values are means, with their standard errors represented by vertical bars (n 3–6). Mean values were significantly different: * P < 0·05, ** P < 0·01. cont, Control.
We then examined the molecular mechanism for the LMW β-glucan-induced expression of HSP70. It is known that stressor-induced expression of HSP is mediated by the activation (phosphorylation) of HSF1. As shown in Fig. 4(c), as well as heat treatment, treatment of cells with LMW β-glucan increased the level of phosphorylated HSF1, suggesting that LMW β-glucan activates HSF1.
Previous studies have suggested that mitogen-activated protein kinases, such as p38, extracellular signal-regulated kinase and c-jun NH2-terminal kinase, are involved in the regulation of the expression of HSP(Reference Kim, Wang and Zhang37, Reference Wang, Khaleque and Zhao38). Therefore, we here examined the effect of inhibitors of mitogen-activated protein kinase on the LMW β-glucan-induced expression of HSP70. Pretreatment of cells with U0126 but not with SB203580 or SP600125 partially suppressed the LMW β-glucan-induced expression of HSP70 (Fig. 4(d) and (e)), suggesting that extracellular signal-regulated kinase is involved in the LMW β-glucan-induced expression of HSP70.
Dectin-1 was reported to be a receptor for β-glucan. In order to test the involvement of dectin-1, we examined the effect of addition of antibody against dectin-1 in the culture medium on the LMW β-glucan-induced expression of HSP70. As shown in Fig. 4(f) and (g), addition of antibody against dectin-1 in the culture medium partially suppressed the β-glucan-induced expression of HSP70, suggesting that dectin-1 is involved in the LMW β-glucan-induced expression of HSP70.
Real-time RT-PCR analysis revealed that treatment of cells with LMW β-glucan induces the expression of muc-1 and muc-5ac mRNA (Fig. 4(h)).
We also examined the effect of LMW β-glucan on the lipopolysaccharide-stimulated expression of pro-inflammatory cytokines, chemokines and CAM in peritoneal macrophages prepared from mice. As shown in Fig. 5, lipopolysaccharide stimulated the mRNA expression of all these factors, and the simultaneous treatment of cells with LMW β-glucan suppressed this lipopolysaccharide-stimulated expression. The results in Figs. 4 and 5 suggest that LMW β-glucan directly affects the expression of HSP70, mucin proteins, pro-inflammatory cytokines, chemokines and CAM.
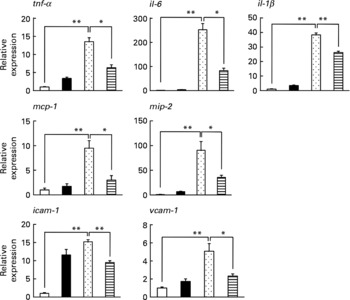
Fig. 5 Effect of low-molecular-weight β-glucan on the lipopolysaccharide (LPS)-stimulated expression of pro-inflammatory cytokines in cultured macrophages. Peritoneal macrophages were pre-incubated with β-glucan (■, 100 μg/ml) for 1 h and further incubated with LPS (, 1 μg/ml) for 3 h in the presence of β-glucan (100 μg/ml) as in the pre-incubation step. The expression of genes was monitored by real-time RT-PCR as described in the legend of Fig. 4. Values are means, with their standard errors represented by vertical bars (n 3–6). Mean values were significantly different: * P < 0·05; ** P < 0·01. □, Vehicle;
, LPS (1 μg/ml)+β-glucan (100 μg/ml). mcp-1, Monocyte chemoattractant protein-1; mip-2, macrophage inflammatory protein-2; icam-1, intercellular adhesion molecule-1; vcam-1, vascular cell adhesion molecule-1.
Effect of pullulan on gastric ulcerogenic response and the expression of heat shock protein 70
Since we used relatively high doses of LMW β-glucan, it may non-specifically interact with the irritants and protect the gastric mucosa. Thus, in order to test the specificity, we used another polysaccharide (α-(1,4 → 1,6)-d-glucan (pullulan)) with molecular weight similar to LMW β-glucan as the control. As shown in Fig. 6(a), oral pre-administration of pullulan (up to 200 mg/kg) did not protect the gastric mucosa against EtOH. We also examined the effect of pullulan on the expression of HSP70 both in vivo and in vitro. Oral administration of pullulan (200 mg/kg) did not increase the expression of HSP70 at the gastric mucosa with and without simultaneous administration of EtOH (Fig. 6(b) and (c)). Furthermore, treatment of human gastric carcinoma cells with pullulan (up to 1 mg/ml) did not affect the expression of HSP70 (Fig. 6(d)). Results in Fig. 6 suggest that LMW β-glucan specifically protects the gastric mucosa against irritants and induces the expression of HSP70.
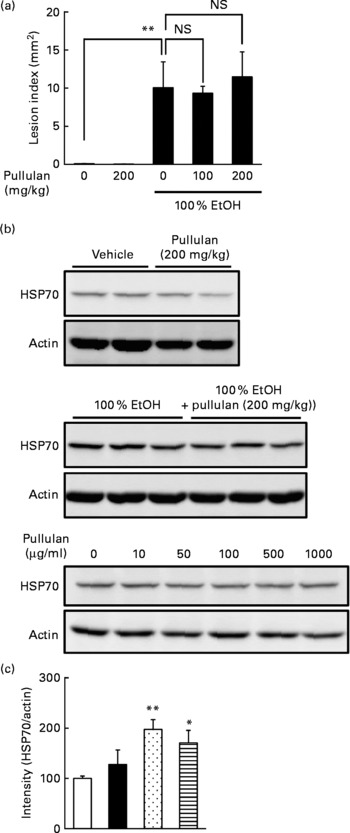
Fig. 6 Effect of pullulan on irritant-induced gastric lesions. The effect of the oral administration of pullulan on (a) ethanol (EtOH)-induced gastric lesions and (b, c) EtOH-induced expression of heat shock protein (HSP) 70 was examined as described in the legend of Figs. 1 and 3, respectively. (d) The effect of pullulan on the EtOH-induced expression of HSP70 in human gastric carcinoma cells was examined as described in the legend of Fig. 4. Values are means, with their standard errors represented by vertical bars (n 3–5). Mean values were significantly different: * P < 0·05, ** P < 0·01. □, Vehicle; ■, pullulan (200 mg/kg); , 100 % EtOH;
, 100 % EtOH+pullulan (200 mg/kg).
Discussion
β-Glucans have been reported to have various clinically beneficial effects, such as anti-tumour effects and protective effects against bacterial, viral, fungal and parasitic challenge(Reference Tsoni and Brown9, Reference Chen and Seviour10). Of the β-glucans, our preparation of β-(1,3)-d-glucan with β-(1,6) branches from A. pullulans (LMW β-glucan) is characterised by a LMW, high purity, high water solubility, low viscosity and a high level of β-(1-6) branching(Reference Kimura, Sumiyoshi and Suzuki14, Reference Kimura, Sumiyoshi and Suzuki25). It has also been shown to have various clinically beneficial effects such as suppression of the allergic response and anti-tumour and anti-metastatic actions(Reference Kimura, Sumiyoshi and Suzuki14, Reference Sumiyoshi, Suzuki and Kimura23, Reference Kimura, Sumiyoshi and Suzuki25, Reference Kimura, Sumiyoshi and Suzuki26). In the present study, we found another beneficial effect of LMW β-glucan, a protective effect against the formation of irritant-induced gastric lesions. Because β-glucans are known to have minimal toxic and adverse effects, we propose that β-glucans, especially LMW β-glucan, may be beneficial as health foods and supplements to prevent the formation of gastric ulcers. The findings in the present study are also important from the nutritional standpoint. Various foods, such as mushrooms, contain β-glucans. Results in the present study suggest that the intake of such foods spontaneously protects our gastric mucosa against the production of gastric lesions. Due to technical problem, we could not examine the pharmacokinetics of LMW β-glucan (such as digestion and absorption). As for other β-glucans, it has been reported that the bioavailability is from 0·5 to 5 % upon oral administration(Reference Rice, Adams and Ozment-Skelton39).
Both gastric mucosal apoptosis and inflammatory responses (such as infiltration of leucocytes) play an important role in the production of irritant-induced gastric lesions, and in the present study, we have shown that both these responses are suppressed by the administration of LMW β-glucan. The anti-apoptotic effect of β-glucans has also been reported elsewhere(Reference Sener, Eksioglu-Demiralp and Cetiner17, Reference Ha, Hua and Grant24). On the other hand, as described earlier, β-glucans have been reported to show opposing (positive or negative) effects on immunoreactions, including inflammation. Possible explanations for this discrepancy include differences in the administration route, average molecular weight, water solubility and purity of the β-glucans employed. Another explanation is that β-glucans may have positive or negative effects on immunoreactions depending on the experimental conditions. In previous reports and in the present study, β-glucans were shown to suppress immunoreactions when the reactions were initiated by other stimuli(Reference Sener, Eksioglu-Demiralp and Cetiner17–Reference Shah, Williams and Keshvara22). On the other hand, β-glucans activate immunoreactions in intact animals(Reference Tsoni and Brown9, Reference Chen and Seviour10, Reference Brown and Gordon11, Reference Engstad, Engstad and Olsen13). We consider that β-glucan is a unique immunomodulator, suppressing immunoreactions when they are abnormally activated, and activating immunoreactions under normal conditions, which is important for maintaining healthy conditions.
Of the three major defensive factors for the gastric mucosa (HSP70, gastric mucin and PGE2), we found that gastric levels of HSP70 and mucin were increased by LMW β-glucan, suggesting that these increases are responsible for the protective effect of LMW β-glucan against the formation of irritant-induced gastric lesions. We also found that EtOH-induced expression of pro-inflammatory cytokines, chemokines and CAM was suppressed by LMW β-glucan. In addition to its cytoprotective effects, the anti-inflammatory activity of HSP70 has recently been revealed; HSP70 inhibits NF-κB, which induces the expression of pro-inflammatory cytokines, chemokines and CAM(Reference Chen, Wu and Zhang40, Reference Weiss, Bromberg and Raj41). Thus, LMW β-glucan-induced expression of HSP70 may be involved in its inhibitory effects on gastric mucosal apoptosis and the inflammatory responses.
An ameliorative effect of HSP70 due to its cytoprotective, anti-inflammatory and molecular chaperone (quality control of proteins) properties has been reported for various diseases. For example, we have shown using transgenic mice expressing HSP70 that HSP70 protects against irritant-induced lesions in the stomach and small intestine, inflammatory bowel disease-related experimental colitis and UV-induced epidermal damage(Reference Tanaka, Tsutsumi and Arai7, Reference Suemasu, Tanaka and Namba8, Reference Tanaka, Namba and Arai30, Reference Asano, Tanaka and Yamakawa42, Reference Matsuda, Hoshino and Yamashita43). The potential therapeutic applicability of HSP70 for use in other diseases, such as neurodegenerative diseases, ischaemia–reperfusion damage and diabetes, has also been suggested(Reference Jana, Tanaka and Wang44–Reference Adachi, Katsuno and Minamiyama46). Interestingly, geranylgeranylacetone, a leading anti-ulcer drug on the Japanese market, has been reported to be an HSP inducer, up-regulating various HSP not only in cultured gastric mucosal cells but also in various tissues in vivo (Reference Hirakawa, Rokutan and Nikawa6). It has been reported that geranylgeranylacetone suppresses not only gastric lesions but also lesions of the small intestine, inflammatory bowel disease-related experimental colitis and neurodegenerative diseases(Reference Suemasu, Tanaka and Namba8, Reference Asano, Tanaka and Yamakawa42, Reference Ohkawara, Nishihira and Takeda47, Reference Katsuno, Sang and Adachi48). Therefore, the results of the present study suggest that LMW β-glucan may be beneficial not only for inhibiting the development of gastric lesions but also for the treatment of other diseases through the induction of HSP70 expression.
Acknowledgements
The present study was supported by Grants-in-Aid for Scientific Research from the Ministry of Health, Labour and Welfare of Japan, as well as the Japan Science and Technology Agency and Grants-in-Aid for Scientific Research from the Ministry of Education, Culture, Sports, Science and Technology, Japan. K.-i. T. and Y. T. did some of the experiments. T. S. prepared LMW β-glucan and T. M. is responsible for conducting the study. None of the authors has a financial relationship with a commercial entity that has an interest in the subject of the study.