Alzheimer's disease (AD) is the commonest form of cognitive decline in the elderly(Reference Whitehouse, Sciulli and Mason1) and there is not yet an effective treatment for AD. Therefore, development of nutritional strategies such as the consumption of fish and seafood which appear to be protective against cognitive decline are essential(Reference Boudrault, Bazinet and Ma2–Reference Plourde, Fortier and Vandal5). Recently, better cognition has been correlated with higher n-3 fatty acid content in erythrocytes(Reference Whalley, Fox and Wahle6), the latter usually being a direct reflection of n-3 fatty acid intake from fish and seafood(Reference Arterburn, Hall and Oken7, Reference Vidgren, Agren and Schwab8). Intake of EPA and DHA, the two major n-3 fatty acids composing fish, is well recognised to lower TAG and increase levels of HDL-cholesterol(Reference Balk, Lichtenstein and Chung9). Some studies have suggested that EPA and DHA concentrations in the plasma of those with AD are lower, but, as a whole, the available literature shows no difference in plasma DHA in those with AD compared with age-matched controls(Reference Cunnane, Plourde and Pifferi3, Reference Plourde, Fortier and Vandal5). The disconnect between apparently low DHA intake but normal plasma DHA in AD possibly results from genetic factors affecting fatty acid metabolism(Reference Cunnane, Plourde and Pifferi3, Reference Plourde, Fortier and Vandal5).
Two such genetic factors are the L162V polymorphism of PPAR-α (L162V) and the ɛ4 allele of apoE (E4). Carriers of either one of these genetic polymorphisms have an altered plasma lipid profile, thereby possibly enhancing their risk of cognitive decline(Reference Panza, D'Introno and Colacicco10, Reference Tai, Corella and Demissie11). Indeed, L162V is a potential risk factor for AD(Reference Brune, Kolsch and Ptok12) whereas E4 is the most important identified genetic risk factor for AD(Reference Coon, Myers and Craig13). Fish and seafood seem not to protect E4 carriers against AD(Reference Huang, Zandi and Tucker14–Reference Barberger-Gateau, Raffaitin and Letenneur16) and, in contrast to non-carriers of E4, there was no link in E4 carriers between cognitive performance and total n-3 fatty acids in erythrocytes(Reference Whalley, Deary and Starr15). To our knowledge, no studies have reported the influence of the L162V on the link between n-3 fatty acids and cognition in the elderly.
We hypothesised that the n-3 fatty acid metabolism may be altered by either one or both the L162V or E4. The objective of the present study was therefore to assess plasma fatty acid profile in carriers and non-carriers of the L162V and/or E4 before and after an n-3 fatty acid supplement.
Methods
Subjects and study design
The present study was an add-on project to a previous study on the impact of L162V on the response of CVD risk factors to n-3 fatty acid supplementation in men where a complete description of the study design is given(Reference Caron-Dorval, Paquet and Paradis17). Twenty-eight men were selected based on whether they were carriers of L162V (fourteen carriers; one homozygote, and fourteen non-carriers). Whether the subjects were also carriers of E4 was only determined after selection for L162V. There were eight carriers of E4 (one homozygote) and twenty non-carriers of E4. None of the subjects was homozygous for both L162V and E4. Subjects consumed 1·9 g EPA and 1·1 g DHA/d for 6 weeks (Ocean Nutrition, Dartmouth, NS, Canada). Capsules were provided to the subjects in a single bottle with an excess of capsules blinded to the subjects so as to evaluate compliance to the treatment. No subject was excluded on the basis of poor compliance.
Fatty acid analysis
Before (baseline, after the run-in phase) and after n-3 fatty acid supplementation, 12 h overnight fast blood sampling was done. Plasma was separated by centrifugation and stored at − 80°C until further analysis. Plasma total lipids were extracted with 2:1 chloroform–methanol and separated by TLC to obtain phospholipids, TAG, cholesteryl esters and NEFA. Fatty acids were transmethylated using 14 % methanolic boron trifluoride, and analysed using GC as previously described(Reference Plourde, Tremblay-Mercier and Fortier18).
DNA analysis
Genetic analyses were performed on genomic DNA isolated from human leucocytes. The L162V polymorphism was determined as previously described(Reference Vohl, Lepage and Gaudet19). The three commonest alleles of ApoE (ɛ2/ɛ3/ɛ4) were analysed using the method described by Hixson & Vernier(Reference Hixson and Vernier20).
Statistics
EPA and DHA data are shown as percentage composition (relative percentage to other fatty acids) and as the change (Δ: after – before the supplement × 100) in percentage composition (mean values and standard deviations). A repeated-measures ANOVA using a general linear model was applied to compare means before and after the n-3 supplementation (SPSS software, version 12.0; SPSS Inc., Chicago, IL, USA) and a multivariate ANOVA was used to determine gene × diet interactions. Baseline or after the n-3 fatty acid supplement statistical significance between carriers and non-carriers of E4 was evaluated by independent t tests. Statistical significance was defined as P ≤ 0·05.
The present study was conducted according to the guidelines laid down in the Declaration of Helsinki and all procedure involving human subjects were approved by the ethics committees of Université Laval Hospital Research Centre and Université Laval. Written informed consent was obtained from all subjects.
Results
Subjects heterozygous or homozygous for either L162V or E4 were grouped together. Based only on L162V, there was no significant gene × diet interaction for EPA or DHA in plasma lipid classes (data not shown). We therefore extended our analysis to evaluate whether E4 may alter the incorporation of EPA and DHA in plasma lipids. To assess the effect of E4 on plasma fatty acids, the subjects were divided as follows: (i) non-carriers of L162V or E4 (n 11); (ii) non-carriers of L162V but carriers of E4 (n 3); (iii) carriers of L162V but non-carriers of E4 (n 9); (iv) carriers of both L162V and E4 (n 5). There was no significant gene × diet interaction for EPA and DHA in plasma lipids (data not shown). However, the change in EPA and DHA in NEFA or TAG appeared to be lower in the two groups carrying E4. We then separated the groups according to the presence of E4 without regard for L162V. E4 carriers at baseline had 67 % higher EPA and 60 % higher DHA in plasma TAG (P = 0·018 and P = 0·032 respectively; Fig. 1). There were significant gene × diet interactions for EPA in NEFA and DHA in TAG (P = 0·043 and P = 0·029, respectively; Fig. 1) since, in the E4 carriers, the changes (represented by Δ) were significantly lower compared with the non-carriers. The number of subjects upon which the present study is based appears appropriate, since sample-size calculations based on means and standard deviations of the carriers and non-carriers of E4 for changes of EPA in NEFA or DHA in TAG and using an α of 0·05 and a power of 0·8 revealed that six subjects per group are needed; our two groups were composed of eight and twenty subjects for the carriers and the non-carriers of E4 respectively.
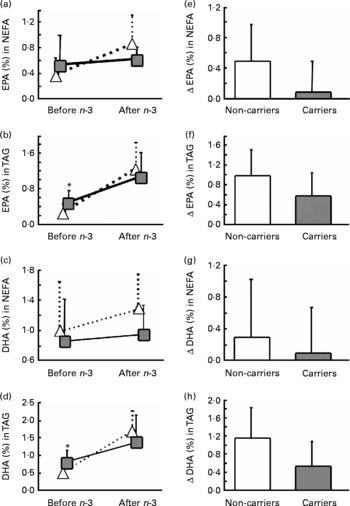
Fig. 1 Average percentage (a–d) and change (Δ) (e–h) in fasting plasma EPA (a, b, e, f)and DHA (c, d, g, h) in NEFA (a, c, e, g) or TAG (b, d, f, h) of the carriers (,
) and non-carriers (
, □) of the apoE ɛ4 allele (E4) before and 6 weeks after an n-3 fatty acid supplement. Values are means, with standard deviations represented by vertical bars. *Mean value was significantly different from that of the non-carriers of E4 at baseline (P ≤ 0·05). The gene × diet interaction for Δ EPA in NEFA (e) was significant (P = 0·043); the gene × diet interaction for Δ EPA in TAG (f) was NS (P = 0·070); the gene × diet interaction for Δ DHA in NEFA (g) was NS (P = 0·477); the gene × diet interaction for Δ DHA in TAG (h) was significant (P = 0·029).
Discussion
We report here that after an n-3 fatty acid supplement, incorporation of EPA and DHA into plasma NEFA and TAG is decreased by the presence of E4, but not by L162V. Since there was no significant gene × diet interaction when separated according to the four genotypes, E4 genotype appears to have a more significant effect on EPA and DHA metabolism than L162V.
Most importantly, EPA did not increase in plasma NEFA of E4 carriers even after consuming the relatively high dose of 1·9 g EPA/d for 6 weeks. Plasma NEFA are reported to be the preferred fatty acid form for transport into the brain and also the preferred form to initiate signalling pathways for the synthesis of eicosanoids(Reference Tassoni, Kaur and Weisinger21). Our data show that E4 may alter n-3 fatty acid transit into or out of NEFA. E4 also reduced DHA incorporation into plasma TAG after the supplement, which indirectly supports the previous suggestion that TAG may be an important storage or transport pool of DHA(Reference Kasim-Karakas22).
As yet we have no mechanism for this interaction between E4 and EPA and DHA metabolism, but we will undertake further studies using the [13C]DHA tracer to determine whether β-oxidation of DHA is higher in E4 carriers than non-carriers and whether its incorporation into plasma lipids is delayed compared with non-carriers. Other possible explanations may relate to the capacity to incorporate and utilise EPA and DHA upon E4 genotype. Retroconversion of DHA to EPA may be another possible contributor to explain higher EPA but since it is apparently very low (about 1·9 %) in humans, its contribution is unlikely to explain the present results(Reference Brossard, Croset and Pachiaudi23). However, whether the rate of retroconversion of DHA to EPA is affected in E4 carriers is unknown. Since the isoforms of ApoE are thought to bind differently to receptors, including the LDL receptor-related protein, thereby impacting on lipid transport, especially of TAG and cholesterol(Reference Anil24), using [13C]DHA could be informative about how E4 affects n-3 fatty acid metabolism.
Previous studies in E4 carriers and non-carriers reported similar EPA and DHA in plasma and erythrocyte phospholipids(Reference Whalley, Deary and Starr15, Reference Laurin, Verreault and Lindsay25, Reference Caslake, Miles and Kofler26) and in plasma cholesteryl esters(Reference Erkkila, Sarkkinen and Lindi27), a result that we confirmed in the present study for plasma phospholipids and cholesteryl esters since we had no gene × diet interaction and the increase was similar to what is reported in the literature(Reference Zuijdgeest-van Leeuwen, Dagnelie and Rietveld28). However, contrary to what we anticipated, carriers of E4 had significantly higher baseline EPA and DHA in plasma TAG (Fig. 1); this is despite similar EPA and DHA intakes before n-3 fatty acid supplementation as reported by FFQ and similar baseline anthropometric and blood biochemistry analysis.
The present study had one major limitation – it was an add-on to the original project which was not specifically designed to study variations in the plasma fatty acid response of E4 carriers and non-carriers to an n-3 fatty acid supplement. However, based on our calculations, we had sufficient number of subjects per group to evaluate this gene × diet interaction. Since the participants were originally selected based on their L162V genotype and although we report here no significant gene × diet interaction when the groups were separated based on L162V, we can not exclude the possibility that L162V interacts with E4. Nevertheless, as previously suggested(Reference Plourde, Fortier and Vandal5), these preliminary results give important support to the idea that E4 affects the link between fish and seafood intake and possibly the risk for cognitive decline because it somehow modulates n-3 fatty acid metabolism. Our data also suggest that the apparent lack of protection against cognitive decline by n-3 fatty acids and/or fish and seafood intake in E4 carriers(Reference Huang, Zandi and Tucker14, Reference Whalley, Deary and Starr15) could well involve altered n-3 fatty acid incorporation into plasma, a possibility that now needs to be evaluated in an independent well-controlled clinical trial.
Acknowledgements
Funding for this project was provided by grant no. MOP-200609 and grant no. 151293 from the Canadian Institutes of Health Research (CIHR), by grant no. 009480 from the Natural Sciences and Engineering Research Council of Canada (NSERC), by grant no. 201796 from the Canada Foundation for Innovation (CFI), by grant no. 008033 from the Canada Research Chairs Secretariat (CRC), the Department of Medicine, Université de Sherbrooke and the Fonds de Recherche en Santé du Québec (FRSQ) for a postdoctoral fellowship to M. P., and the Research Centre on Aging.
M. P. was the principal investigator for the add-on project, has full access to the fatty acid profile of the plasma, interpreted the data and wrote the manuscript. M.-C. V., P. C. and S. L. were the principal investigators of the original study and revised the data and the manuscript, M. V. did the fatty acid analysis of the plasma lipid classes and contributed to the manuscript, S. C. C. participated in the interpretation of the data and in drafting and editing the manuscript.
There are no conflicts of interest.