Ageing is the most important risk factor for cognitive decline, dementia and Alzheimer's disease. Besides ageing, there are several other behavioural factors such as diet, obesity, smoking and physical activity that affect cognitive function(Reference Lee, Back and Kim1). There is considerable evidence that one-carbon metabolites are essential in neurodevelopment and brain function. High plasma concentrations of total homocysteine (tHcy) and low concentrations of folate and/or vitamin B12 have been associated with cognitive deficit and Alzheimer's disease(Reference Smith2). Betaine and choline are quaternary ammonium compounds metabolically linked to both lipid and folate-dependent one-carbon metabolism(Reference Ueland3). Choline lowers plasma tHcy concentrations, even when dietary consumption of folate and other B vitamins is adequate(Reference da Costa, Gaffney and Fischer4, Reference Lee, Jacques and Dougherty5), and high doses of betaine, alone or in combination with other B vitamins, are used in the treatment of homocystinuria(Reference Ueland3).
Betaine serves as a methyl donor in a reaction converting homocysteine to methionine, catalysed by the hepatic enzyme betaine-homocysteine methyltransferase(Reference Ueland, Holm and Hustad6). It is not known whether betaine plays a role within the brain, although a betaine/γ-aminobutyric acid (GABA) transporter has been identified in astrocytes(Reference Madsen, White and Schousboe7). There is only one published report of a positive association between plasma concentrations of betaine and cognition in human subjects(Reference Eussen, Ueland and Clarke8).
Choline, an essential dietary constituent(Reference Zeisel and da Costa9), is required for the synthesis of acetylcholine, phospholipids and betaine(Reference Ueland3). Because acetylcholine is a neurotransmitter involved in attention, learning and memory, choline may be important in many cognitive processes and in brain development(Reference Zeisel10). Choline, as a component of phosphatidylcholine, also plays a role in membrane structure and in membrane-mediated cell signalling(Reference Zeisel11). In animal studies, rats in impoverished environmental conditions (no toys or other playing opportunities) fed cytidine 5'-diphosphocholine (CDP)-choline were protected from memory impairment(Reference Teather and Wurtman12). Moreover, in rats, choline supplementation during the embryonic period improves memory performance later in life(Reference McCann, Hudes and Ames13). In contrast, one study found no correlation between human maternal and cord blood choline concentrations and subsequent child intelligence quotient scores at 5 years of age(Reference Signore, Ueland and Troendle14). In a folate-fortified human population, low serum concentrations of total choline were associated with an elevated risk of neural tube defects(Reference Shaw, Finnell and Blom15). In another study(Reference Innis, Davidson and Bay16), a positive relationship between plasma choline concentration and acetylcholine concentration was observed in children with cystic fibrosis who had low choline status, but not in healthy children. Whether plasma choline concentrations are associated with acetylcholine concentration in older adults is not known, and controlled clinical trials have not shown any clinical value of choline and phosphatidylcholine in the treatment of cognitive dysfunction in Alzheimer's disease(Reference Amenta and Tayebati17, Reference Higgins and Flicker18). On the other hand, in a recent study in a large, non-demented community-based cohort, higher concurrent choline intake was associated with better cognitive performance(Reference Poly, Massaro and Seshadri19).
Although choline plays an important role in the development as well as functioning of the central nervous system, few studies have investigated the association between plasma concentrations of choline and cognitive function. We have examined the associations between plasma concentrations of free choline, betaine and cognitive functions monitored in an elderly subsample of the Hordaland Health Study. We also investigated possible interactions with other one-carbon metabolites, because choline, betaine and folate are interchangeable sources of one-carbon units, and, together with vitamin B12, determinants of tHcy(Reference Ueland3), which is a strong marker of future cognitive decline(Reference Smith2).
Subjects and methods
Study population
The Hordaland Health Study was conducted from 1997 to 1999 as a collaborative effort between the University of Bergen, University of Oslo, local health services and the National Health Screening Service (now the Norwegian Institute of Public Health). Details of the study and of recruitment to the cognitive sub-study have been described elsewhere(Reference Refsum, Nurk and Smith20, 21). Briefly, the cognitive sub-study was confined to all those living in the city of Bergen and who were born between 1925 and 1927. A total of 2841 elderly subjects attended both the baseline (1992–3) and the follow-up (1997–9) studies and were in the latter study invited, independent of their cognitive status, to participate in cognitive tests; 2197 (77·3 %) of these subjects agreed to participate. In the present study, we have restricted the cross-sectional analyses to 2195 individuals for whom plasma concentrations of free choline and betaine(Reference Konstantinova, Tell and Vollset22) and cognitive function measurements were available. The present study was conducted according to the guidelines laid down in the Declaration of Helsinki and all procedures involving human subjects were approved by the Regional Committee for Medical Research Ethics of Western Norway. Written informed consent was obtained from all subjects.
Data collection
Cognitive testing was performed at the study location by trained nurses after the standard cardiovascular examinations of the National Health Screening Service(Reference Bjartveit, Foss and Gjervig23) were completed. The cognitive test battery included six tests(Reference Nurk, Drevon and Refsum24): the Kendrick Object Learning Test (KOLT, episodic memory)(Reference Kendrick25); the Trail Making Test, part A (TMT-A, sensorimotor speed)(Reference Reitan26); a modified version of the Digit Symbol Test (m-DST, perceptual speed and executive function)(Reference Wechsler27); a modified form of the Block Design (m-BD) (visuospatial skills)(Reference Wechsler27); a modified version of the Mini-Mental State Examination (m-MMSE, global cognition)(Reference Braekhus, Laake and Engedal28); an abridged version of the Controlled Oral Word Association Test (COWAT), also called ‘S-task’ (semantic memory)(Reference Benton and Hamsher29). For all cognitive tests, the higher scores indicate better performance, except for the TMT-A where the speed of fulfilment is important, i.e. the shorter the time used, the better the results.
Non-fasting blood samples used for the preparation of plasma were collected into evacuated tubes containing EDTA, and stored at − 80°C. Plasma concentrations of free choline, betaine, tHcy and creatinine were measured by normal-phase liquid chromatography–tandem MS detection(Reference Holm, Ueland and Kvalheim30). The within- and between-day imprecision (CV) for plasma free choline and betaine varied between 2·1 and 8·8 %(Reference Holm, Ueland and Kvalheim30). Plasma concentrations of folate and vitamin B12 were measured by Lactobacillus casei (Reference Molloy and Scott31) and L. leichmannii microbiological assays(Reference Kelleher and Broin32). Plasma methylmalonic acid (MMA) concentration was measured by a modified GC–MS method based on ethylchloroformate derivatisation(Reference Husek33). Methylenetetrahydrofolate reductase (MTHFR) 677C → T and apoE ɛ4 genotypes were determined in the packed cell fraction of blood samples using the real-time and one-stage PCR techniques, respectively(Reference Ulvik and Ueland34, Reference Wenham, Price and Blandell35). Details on self-reported information on the history of CVD, education, smoking status and depression have been reported previously(Reference Nurk, Refsum and Drevon36).
Statistical analyses
Because the distributions of blood measurements showed a markedly skewed distribution, log-transformed data were used in all analyses. Pearson's correlation coefficients were determined between choline, betaine and different covariates. Cut-off values for low plasma concentrations of free choline and betaine were set at the 20th percentile of the study population values: ≤ 8·4 μmol/l for choline and ≤ 31·1 μmol/l for betaine. For a comparison between groups, Pearson's χ2, independent sample t tests and ANOVA were applied. Preliminary analyses to identify covariates showed that cognitive performance was associated with education, plasma folate concentration and depression (all test scores were significantly associated). Of the six cognitive test scores, five were significantly associated with tHcy and four were associated with apoE ɛ4 allele and CVD history. Sex, smoking and plasma concentration of vitamin B12 were significantly associated with two out of six cognitive test scores, whereas plasma concentrations of MMA and creatinine, and the MTHFR 677C → T T allele were significantly associated with only one cognitive test score. Plasma concentrations of free choline or betaine were significantly associated with sex, apoE ɛ4 allele, the history of CVD, education, smoking status, plasma concentrations of folate, MMA, tHcy and creatinine. Inclusion of plasma concentrations of vitamin B12, MMA or tHcy as covariates did not alter the results significantly and are therefore omitted from final statistical models to avoid potential over-adjustment. Thus, the final adjusted models included the following variables: sex, education, apoE ɛ4 allele, CVD history, smoking status, plasma concentrations of folate and creatinine, and MTHFR 677C → T genotype. Although the depression score was highly correlated with cognitive performance, it was excluded from statistical models due to a large proportion of missing values. However, the effect of depression is reported separately when it affected otherwise significant results. Given the narrow age range, adjustment for age did not change the results and has not been included. In multivariate analyses, subjects with missing data in one or more variables were excluded. Gaussian generalised additive regression models, as implemented in S-PLUS 6.2 for Windows (Insightful Corporation), were used to generate graphic representations of the dose–response relationships, using a sex-adjusted model. Multiple linear regression analyses were used to examine significant associations between the cognitive test scores and plasma concentrations of free choline and betaine using both a sex-adjusted model and a model adjusted for the variables referred to the final model above. Potential interaction between plasma free choline or betaine and other one-carbon metabolites (plasma concentrations of folate, vitamin B12, MMA and tHcy) on poor cognitive performance (cut-off points for poor cognitive test scores were set at about the 10th percentile of the cognitive test score, except for the TMT-A, for which the 90th percentile was used) was assessed by multiple logistic regression analyses including an interaction term where plasma free choline and betaine concentrations were dichotomous variables both as a main effect and an interaction term. Similarly, other one-carbon metabolites were present in interaction models as dichotomous variables. Cut-off values for low or high concentrations were set either at the 20th or 80th percentile: low folate ≤ 5·06 nmol/l; low vitamin B12 ≤ 257 pmol/l; high tHcy ≥ 14·3 μmol/l; high MMA ≥ 3·95 μmol/l. All interaction models were adjusted for the same covariates as mentioned for the final models above, except for the models where plasma concentration of folate was studied as an effect modifier.
All statistical analyses, except for generalised additive models, were performed using the SPSS version 16.0 for Windows (SPSS, Inc.). A two-sided P value less than 0·05 was considered significant.
Results
The characteristics of the study population, including cognitive scores, are presented in Table 1. Of the 1992 participants who completed all cognitive tests, 231 (less than 12 %) performed poorly in two or more tests, including four individuals who performed poorly in all six tests, nine in five tests, thirty in four tests, sixty-five in three tests and 123 in two tests.
Table 1 Characteristics of the study population (Number of participants and percentages; mean values and 95 % confidence intervals)

MTHFR, methylenetetrahydrofolate reductase; HADS, Hospital Anxiety and Depression Scale; KOLT, Kendrick Object Learning Test (episodic memory); TMT-A, part A of the Trail Making Test (sensorimotor speed); m-DST, modified version of the Digit Symbol Test (perceptual speed and executive function); m-BD, modified version of the Block Design (visuospatial skills); m-MMSE, modified version of the Mini-Mental State Examination (global cognition); COWAT, S-task from the Controlled Oral Word Association Test (semantic memory).
* Geometric means for blood parameters.
The correlation coefficients between choline, betaine and other plasma values, genetic factors, lifestyle variables and cognitive performance are presented in Table 2. The strongest correlation was between plasma concentrations of choline and betaine, but also several other blood parameters, notably plasma creatinine, correlated with choline and betaine. CVD history and education were correlated with choline as well as betaine, and betaine was also correlated with the number of apoE ɛ4 alleles. Episodic memory was negatively and sensorimotor speed positively correlated with plasma concentrations of choline as well as betaine, although these correlations were weak. Global cognition was positively correlated with plasma choline concentration, and perceptual speed, executive function and semantic memory were positively correlated with plasma betaine concentration.
Table 2 Pearson's correlations between plasma concentrations of free choline, betaine and different covariates (Number of participants and correlation coefficients)

MTHFR, methylenetetrahydrofolate reductase; KOLT, Kendrick Object Learning Test (episodic memory); TMT-A, part A of the Trail Making Test (sensorimotor speed); m-DST, modified version of the Digit Symbol Test (perceptual speed and executive function); m-BD, modified version of the Block Design (visuospatial skills); m-MMSE, modified version of the Mini-Mental State Examination (global cognition); COWAT, S-task from the Controlled Oral Word Association Test (semantic memory).
Men had significantly higher mean plasma concentrations of both choline and betaine than women: 10·8 (95 % CI 10·6, 10·9) v. 9·6 (95 % CI 9·5, 9·7) μmol/l (P< 0·001) for choline and 43·9 (95 % CI 43·2, 44·6) v. 35·9 (95 % CI 35·3, 36·4) μmol/l (P< 0·001) for betaine. Participants with low plasma concentrations of choline compared with those with high concentrations had lower concentrations of betaine (mean 33·0 (95 % CI 32·1, 33·9) v. 41·0 (95 % CI 40·5, 41·5) μmol/l, P< 0·001), MMA (mean 0·19 (95 % CI 0·18, 0·20) v. 0·20 (95 % CI 0·20, 0·21) μmol/l, P< 0·001) and creatinine (mean 87 (95 % CI 85, 88) v. 93 (95 % CI 92, 94) μmol/l, P< 0·001). Compared with high betaine status, subjects with low plasma concentrations of betaine had lower concentrations of choline (mean 8·7 (95 % CI 8·5, 8·9) v. 10·5 (95 % CI 10·4, 10·6) μmol/l, P< 0·001), folate (mean 6·8 (95 % CI 6·5, 7·2) v. 7·7 (95 % CI 7·6, 8·0) μmol/l, P< 0·001), MMA (mean 0·19 (95 % CI 0·19, 0·20) v. 0·20 (95 % CI 0·20, 0·21) μmol/l, P= 0·025) and creatinine (mean 88 (95 % CI 87, 89) v. 93 (95 % CI 92, 93) μmol/l, P< 0·001), but higher concentrations of tHcy (mean 12·4 (95 % CI 12·0, 12·8) v. 11·4 (95 % CI 11·2, 11·5) μmol/l, P< 0·001).
Participants with low plasma choline concentrations had poorer cognitive performance in sensorimotor speed, perceptual speed and executive function than subjects with high plasma free choline concentrations (Table 3); the results became more significant for sensorimotor speed, perceptual speed and executive function, and global cognition after multiple adjustments for sex, education, apoE ɛ4 allele, CVD history, smoking status, plasma concentrations of folate and creatinine, and MTHFR 677C → T genotype. Participants with low plasma betaine concentrations performed significantly better than those with high betaine concentrations in episodic memory, whereas performance related to sensorimotor speed was worse (Table 4). After multiple adjustments, the betaine associations were no longer significant.
Table 3 Cognitive test performance by status of plasma concentration of free choline* (Mean values and 95 % confidence intervals, number of participants and percentages)
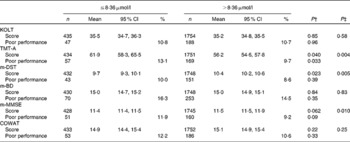
KOLT, Kendrick Object Learning Test (episodic memory); TMT-A, part A of the Trail Making Test (sensorimotor speed); m-DST, modified version of the Digit Symbol Test (perceptual speed and executive function); m-BD, modified version of the Block Design (visuospatial skills); m-MMSE, modified version of the Mini-Mental State Examination (global cognition); COWAT, S-task from the Controlled Oral Word Association Test (semantic memory).
* Cut-off value for the low plasma concentration of free choline was set at the 20th percentile of the study population values. Cut-off points for poor cognitive test performance were set at about the 10th percentile of the cognitive test score, except for the TMT-A, for which the 90th percentile was used: KOLT ≤ 25; TMT-A ≥ 111; m-DST ≤ 5; m-BD ≤ 12; m-MMSE ≤ 10; COWAT ≤ 8.
† Independent sample t test or Pearson's χ2.
‡ Univariate ANOVA, adjusted for sex, education, apoE ɛ4 allele, CVD history, smoking status, plasma concentrations of folate and creatinine and methylenetetrahydrofolate reductase 677C → T genotype.
Table 4 Cognitive test performance by status of plasma concentration of betaine* (Mean values and 95 % confidence intervals, number of participants and percentages)

KOLT, Kendrick Object Learning Test (episodic memory); TMT-A, part A of the Trail Making Test (sensorimotor speed); m-DST, modified version of the Digit Symbol Test (perceptual speed and executive function); m-BD, modified version of the Block Design (visuospatial skills); m-MMSE, modified version of the Mini-Mental State Examination (global cognition); COWAT, S-task from the Controlled Oral Word Association Test (semantic memory).
* Cut-off value for the low plasma concentration of betaine was set at the 20th percentile of the study population values. Cut-off points for poor cognitive test performance were set at about the 10th percentile of the cognitive test score, except for the TMT-A, for which the 90th percentile was used: KOLT ≤ 25; TMT-A ≥ 111; m-DST ≤ 5; m-BD ≤ 12; m-MMSE ≤ 10; COWAT ≤ 8.
† Independent sample t test or Pearson's χ2.
‡ Univariate ANOVA, adjusted for sex, education, apoE ɛ4 allele, CVD history, smoking status, plasma concentrations of folate and creatinine and methylenetetrahydrofolate reductase 677C → T genotype.
There was a positive dose–response relationship between global cognition (m-MMSE score) and plasma choline concentrations (Fig. 1), and the association remained significant in the linear regression model after adjustments for sex, education, apoE ɛ4 allele, CVD history, smoking status, plasma concentrations of folate and creatinine, and MTHFR 677C → T genotype (P= 0·012). Plasma betaine concentration was positively and linearly associated with sensorimotor speed (TMT-A score) and semantic memory (COWAT score), and there were also borderline significant linear associations with global cognition (m-MMSE score), perceptual speed and executive function (m-DST score). However, none of the linear associations between plasma concentrations of betaine and cognitive test performances remained significant after multiple adjustments.

Fig. 1 Associations between different cognitive test scores and plasma concentrations of choline and betaine obtained by Gaussian generalised additive regression models. On the vertical axis, the model generates a reference value of zero that approximately corresponds to the value of cognitive test score associated with the mean of plasma concentrations of choline and betaine for all subjects. Solid lines are the estimated dose–response curves; shaded areas represent 95 % CI. P values adjusted for sex are from corresponding multiple linear regression analyses. The data for the lowest and highest 1 percentile of plasma concentrations are not included. KOLT, Kendrick Object Learning Test; TMT-A, part A of the Trail Making Test; m-DST, modified version of the Digit Symbol Test; m-BD, modified version of the Block Design; m-MMSE, modified version of the Mini-Mental State Examination; COWAT, abridged version of the Controlled Oral Word Association Test (S-task).
The interaction analyses based on cross-sectional data showed that low plasma free choline concentrations combined either with low vitamin B12 or high plasma MMA concentration increased the risk ratio (RR) for poor performance by 2·6–3·1-fold in episodic memory, global cognition, semantic memory and visuospatial skills (Table 5). Similarly, low plasma concentrations of betaine combined with high plasma concentrations of MMA increased the RR for poor performance in episodic memory 2·5 times (Table 5). In addition, we found that low plasma concentrations of free choline and betaine together more than doubled the RR for poor visuospatial skills, the RR being 2·01 (95 % CI 0·98, 4·12, P= 0·056). There were no significant interactions between low plasma concentrations of free choline or betaine and low folate or high tHcy concentrations on cognitive functions (data not shown).
Table 5 Interaction between low plasma concentrations of free choline or betaine and different covariates on poor cognitive performance* (Risk ratios and 95 % confidence intervals)

KOLT, Kendrick Object Learning Test (episodic memory); MMA, methylmalonic acid; m-BD, modified version of the Block Design (visuospatial skills); m-MMSE, modified version of the Mini-Mental State Examination (global cognition); COWAT, S-task from the Controlled Oral Word Association Test (semantic memory).
* Cut-off values for low choline and low or high concentrations of other variables were set either at 20th or 80th percentile: low choline ≤ 8·36 μmol/l; low betaine ≤ 31·1 μmol/l; low vitamin B12 ≤ 257 pmol/l; high MMA ≥ 3·95 μmol/l. Cut-off points for poor cognitive test scores were set at about the 10th percentile of the cognitive test score: KOLT ≤ 25; m-BD ≤ 12; m-MMSE ≤ 10; COWAT ≤ 8.
† The reference group includes subjects with normal concentrations of both plasma free choline or betaine and vitamin B12 or MMA.
‡ Adjusted for sex, education, apoE ɛ4 allele, history of CVD, smoking status, plasma folate, creatinine and methylenetetrahydrofolate reductase 677C → T genotype.
Because inclusion of the depression score as a covariate in the interaction models significantly reduced the number of participants due to missing data, it was excluded from the final models. However, although most of the interactions maintained their strength and remained significant after adjusting for depression (data not shown), the RR were no longer significant for combinations of low choline and low vitamin B12 on the KOLT (RR 2·30, 95 % CI 0·88, 6·03) and m-MMSE scores (RR 2·41, 95 % CI 0·90, 6·46).
Discussion
In a population-based elderly cohort of 2195 individuals, we have shown that low plasma concentrations of free choline were cross-sectionally associated with poor performance in global cognition, sensorimotor speed, perceptual speed and executive function, after adjusting for other factors known to influence cognition. The associations between plasma betaine concentrations and cognitive function were no longer significant after controlling for these other risk factors. There were significant interactions between low plasma concentrations of free choline or betaine and markers of vitamin B12 status (plasma vitamin B12 and MMA) on cognitive performance.
The associations of plasma or serum concentrations of choline and betaine with cognition in human subjects have rarely been studied. Among Dutch elderly people, plasma betaine concentrations were positively associated with the domains of construction, sensorimotor speed and executive function(Reference Eussen, Ueland and Clarke8). In addition, there was a tendency that participants with the largest increase in betaine concentrations showed a larger increase in memory performance when compared with participants with the smallest increase in betaine concentrations after 24 weeks of supplementation with folate and vitaminie: B12(Reference Eussen, Ueland and Clarke8). In line with those results, we found positive cross-sectional associations between plasma concentrations of betaine and sensorimotor speed, executive function, perceptual speed and semantic memory. However, the associations disappeared after multiple adjustments. Eussen et al. (Reference Eussen, Ueland and Clarke8) did not find significant associations between cognition and plasma choline concentration. In contrast, we observed that choline was positively associated with sensorimotor speed, perceptual speed, executive function and global cognition. Moreover, we also observed a significant dose–response relationship between plasma concentrations of choline and global cognition. The reasons for the differences in observations between the present findings and those of Eussen et al. (Reference Eussen, Ueland and Clarke8) are unknown, but may be partly due to different study designs and sample sizes, as the Dutch study was a randomised, double-blind, placebo-controlled trial with 195 participants.
Surprisingly, episodic memory was inversely correlated with plasma concentrations of choline and betaine in our dataset. The mechanisms behind these associations are unclear, or as the associations were relatively weak and there was no dose–response effect, these associations may appear by chance.
It has been suggested that plasma concentration of free choline represents only a minor fraction of the total choline pool, and thus may be a poor marker of choline status and metabolism in the brain(Reference Eussen, Ueland and Clarke8). Moreover, even if administration of free choline increases brain choline availability, it does not increase acetylcholine synthesis or release, which may explain its ineffectiveness in relieving the cognitive symptoms of Alzheimer's disease(Reference Amenta and Tayebati17). In animal studies, betaine concentration among different tissues was lowest in the brain and was about 25 % of that in the plasma; and there was no relationship between brain and plasma betaine concentrations(Reference Slow, Lever and Chambers37). These findings may explain why the associations between cognition and plasma concentrations of free choline or betaine in different studies are inconsistent and indicate that plasma free choline and betaine themselves are inadequate predictors of status of these nutrients in tissues.
The effects of betaine and choline on one-carbon metabolism are often present only in subgroups, for example, among subjects with folate deficiency or with low plasma concentrations of other B vitamins (B2, B6 and B12) in combination with the TT genotype of the MTHFR 677C → T polymorphism(Reference Ueland3). This reflects the convergence of both betaine-homocysteine methyltransferase and vitamin B12-dependent methionine synthase on methionine formation, whereby folate, choline and betaine become fungible sources of one-carbon units(Reference Ueland3). These interrelationships may explain why combined abnormal concentrations of one-carbon metabolites have a stronger effect on cognition than each metabolite alone. In the present study, neither low concentrations of vitamin B12, nor high concentrations of MMA alone, were associated with poor cognitive performance, but low vitamin B12 concentration in combination with low plasma concentration of choline nearly tripled the RR for poor cognitive performance related to episodic memory, global cognition and semantic memory. Similarly, high MMA concentration combined with low betaine or low plasma free choline concentrations more than doubled the RR for poor performance in episodic memory and visuospatial skills, respectively.
The strengths of the present study include a large population-based sample with six different cognitive tests. A major limitation of the study is the cross-sectional design as cognition in the elderly results from long-term exposures(Reference Launer38, Reference Whalley, Dick and McNeill39) and subjects with impaired cognition may have altered their diet as a consequence of a change in their cognitive function. However, because the cognition of participants in the present study was not seriously impaired, we do not believe that the present findings are related to reversed causality. There is also a risk for type I error as the significant associations in the present study are often weak and partly contrasting. Last but not least, as 77·3 % of the 2841 study attendees volunteered for cognitive testing, the possibility of recruitment bias should be considered.
In conclusion, the overall associations between cognition and plasma concentrations of free choline and betaine in human subjects seem to be modest and further investigations are needed, particularly in relation to interactions with other risk factors, and with genetic polymorphisms that may affect choline(Reference Zeisel40).
Acknowledgements
The present study was supported by the Charles Wolfson Charitable Trust, Alzheimer's Research (UK), the Advanced Research Programme of Norway, the Johan Throne Holst Foundation for Nutrition Research, the Freia Medical Foundation, University of Oslo, and the Foundation to Promote Research into Functional Vitamin B12 Deficiency (Norway). We are grateful to Elfrid Blomdal (University of Bergen, Norway) for her excellent support with the literature. H. R., G. S. T., P. M. U. and S. E. V. participated in the study design and the organisation of the data collection. K. E., H. A. N. and A. D. S. assisted with the design and organisation of the cognitive sub-study. E. N. conducted the statistical analyses and wrote the first draft of the manuscript. All co-authors interpreted the results, contributed to the study design and participated in critically revising the manuscript. None of the authors had any conflict of interest.