In the contemporary ageing society, ageing and related diseases have become the most serious issues that need to be urgently addressed. The incidence and mortality of Alzheimer’s disease, Parkinson’s disease and other major diseases that endanger the health and life of the elderly also increase with age(Reference Hou, Dan and Babbar1), placing a heavy burden on society and families. Almost all ageing brains exhibit characteristic changes associated with neurodegeneration(Reference Wyss-Coray2). Therefore, the prevention and improvement of various potential risk factors brought about by ageing via non-pharmacological means have become a research hotspot. Studies have demonstrated that supplementation of natural products with high antioxidant activity can increase antioxidant enzyme activities in vivo, reduce oxidative protein damage(Reference Chauhan and Chauhan3), reverse memory impairment(Reference Unno, Ishikawa and Takabayashi4) and delay brain ageing in the ageing mice(Reference Liu, Zhang and Xiao5).
The annual production of peanuts in China currently ranks first worldwide, with the planting area and peanut production growing every year. More than 55 % of peanuts are used for oil production, and a large number of peanut meal by-products are produced during the pressing and refining process(Reference Jiang, Liu and Liu6). It is found that peanut meal is rich in a variety of nutrients, such as protein, fiber, minerals, vitamins, polyphenols and so on(Reference Mei, Zhou and Hu7), of which the content of protein is as high as 47–55 %(Reference Reddy, Chen and Yang8). However, due to the imperfect existing peanut oil processing technology, the protein denaturation in peanut meal after high temperature squeezing, the essential amino acid content is unbalanced and easy to be contaminated by aflatoxin(Reference White, Oakes and Shi9), thus peanut meal is mainly utilised as animal feed or even fertilizer, with low added value and massive waste of resources. In reaction to the phenomenon, many new processing technologies have emerged to improve the utilisation rate of peanut meal. Among them, the fermentation technology can take advantage of the abundant protein and soluble sugar in peanut meal to provide nutrition for microorganisms and produces plenty of proteins, amino acids small molecular peptides(Reference Couto and Sanromán10) and polyphenols that are more easily to be absorbed and utilised by the human body through fermentation decomposition(Reference Xiong, Zhou and Wang11), which has been proved to be a low-cost and high-benefit processing technology.
Bacillus natto (B. natto) is a subspecies of Bacillus subtilis. It belongs to Gram-positive aerobic species, with long rod-shaped cells, acid and heat resistance and strong pathogenic bacteria inhibition ability(Reference Li, Wang and Li12,Reference Song, Yu and Song13) and is one of the forty probiotics announced by the USA FDA(Reference Wu, Chang and Shih14). B. natto can secrete enzymes such as protease, amylase, lipase and phytase and can decompose macromolecules including protein, fat and carbohydrates into small peptides, amino acids, organic acids, oligosaccharides and other components that are easily absorbed by the human body(Reference Dong, An and Wang15), thereby improving the utilisation rate of fermentation products. More importantly, the food source of nattokinase has the advantages of convenient oral administration and excellent gastrointestinal stability compared with other commercial drugs(Reference Amadou, Le and Shi16,Reference Weng, Yao and Sparks17) and has been widely used in the fields of medicine, health food, agriculture and animal husbandry so far(Reference Kimura and Yokoyama18). It has been shown that the fermentation products of B. natto have high antioxidant activity(Reference Chu, Zhao and Lu19), which can help eliminate the excessive free radicals in the body(Reference Chen, Gao and Wei20), and have the ability to improve or enhance cognitive function(Reference Jeong, Ryu and Yang21). Therefore, using B. natto to ferment peanut meal can make reasonable utilisation of oil production waste and achieve improvements of nutritional composition.
Our previous studies have shown that the supplementation of the peanut meal extract fermented by B. natto remarkably improved the cognitive function of the mice during growth and development(Reference Jiang, Ding and Liu22) and can effectively improve hyperlipidaemia caused by a high-fat diet in mice(Reference Lu, Ding and Jiang23), but it is still unclear whether it has beneficial effects on cognitive function in ageing rats. Herein, based on the aforementioned beneficial potentialities of B. natto and fermented peanut meal, the d-galactose was used to establish ageing models, and the rats were fed with the extracts prepared from peanut meal fermented by B. natto. By employing the behavioural and histological methods, from the aspects of hippocampal tissue structure, oxidative stress homoeostasis and learning and memory abilities, the anti-ageing effect of the extract on the rats have been studied, aiming to provide theoretical and experimental basis for the development of functional foods based on the rational utilisation of peanut by-product resources.
Materials and methods
Preparation of peanut meal extracts fermented with Bacillus natto
Peanut meal (Qingdao Kerry Vegetable Oils Co., Ltd., Qingdao, China) – soyabean meal – bran (7:2:1) was utilised as the fermentation medium, and then the seed liquid of B. natto (preserved in Food Engineering Laboratory, Qingdao University, Qingdao, China) was inoculated. The mixture was cultured at 37°C for 38 h, and subsequently, the ferments were centrifuged, filtered and freeze-dried for reservation.
Determination of the main components of fermentation extract by Bacillus natto
The Lowry–Folin phenol method was implemented to measure the Natto kinase activity(Reference Lu, Lin and Wu24), free amino acids were determined via the ninhydrin method(Reference Jones, Owen and Farrar25), peptides were detected by the biuret method(Reference Xiao, Ren and Zhang26), total flavonoids were tested by the NaNO2-Al(NO3)3-NaOH chromogenic method(Reference Hu, Ding and Wei27), polysaccharide was measured by the phenol-sulfuric acid method(Reference Cuesta, Suarez and Bessio28) and the number of B. natto was calculated by the plate counting method(Reference Massa, Caruso and Trovatelli29).
Animals and materials
All the animal experiment protocols and procedures were approved by the Ethics Committee of Qingdao University (QYFY WZLL 25879), and the experiments followed the National Guidelines for the Care and Use of Laboratory Animals and adhered to the ARRIVE Guidelines for reporting animal research(Reference Gao, Zhang and Li30). Seventy-two specific pathogen free (SPF) Sprague-Dawley (sd) rats (female, 4–5 months old, about 240 g) were purchased from Experimental Animal Center of Shandong Lukang (license number SCXK Lu 20130001). The rats were housed in the laboratory animal facilities (School of Public Health, Qingdao University, Qingdao, China), placed under a 12-h light/dark cycle, controlled at 22 ± 2°C and allowed free access to food and water. After 1 week of adaptation, rats were divided into six groups by the random number table according to the experimental instructions(Reference Festing and Altman31) as following: normal control group (N), ageing model group (M), FE low-dose group (FL), FE medium-dose group (FM), FE high-dose group (FH) and vitamin E positive control group (Y), each with 12 animals. Except for the group N (treated with the same dose of normal saline), other groups were injected intraperitoneally with 400 mg/ (kg/d) of d-galactose every day for 8 weeks to induce an ageing rat model(Reference Ho, Liu and Wu32). Besides the d-galactose treatment, different ingredients were given by gavage: groups N and M with 0·2 ml/10 (g/d) of normal saline, group Y with 100 mg/ (kg/d) of vitamin E, groups FL, FM and FH with FE at doses of 60 mg/ (kg/d), 300 mg/ (kg/d) and 1500 mg/ (kg/d), respectively. The experimental design is depicted in Fig. 1. Lastly, after intervention for 8 weeks, rats were sacrificed after pentobarbital anaesthesia, the blood samples were taken from abdominal aorta and centrifuged at 644 g for 10 min to obtain the serum, while organs of each tissue were taken and placed at –80°C for storage. The viscera indices were calculated according to the following formula: Index = weight of viscera (mg)/body weight (g).

Fig. 1. Experimental protocol and design.
Morris water maze tests
On the eighth week of intervention, learning and memory skills were measured by the Morris water maze (MWM) tests(Reference Tomas Pereira and Burwell33). The experiment was performed as previously described(Reference Li, Men and Zhu34,Reference Subash, Essa and Braidy35) . The rats were trained for 5 d, daily training was consisted of four stages in which the rats were put into the water from four different quadrants of the tank and the time from entering the water to climbing the platform was recorded, namely, the escape latency. If the rats did not find the platform within 60 s, it was induced to the platform with a rod and kept for 10 s. In this case, the escape latency was recorded as 60 s. After 5 d, the platform was removed, and the rats were put into the water in the opposite side of the original platform quadrant. Then, the time ratio (the ratio of total swimming time in the target quadrant), distance ratio (the ratio of total swimming distance in target quadrant), swimming speeds and numbers of crossing over the platform were recorded by an MWM image analysis system (Mobile Datum).
Determination of antioxidant indexes of brain tissue
The brain tissues of the rats were finely minced and homogenised in 50 mM normal saline and centrifuged at 10 000 g , 4°C for 15 min in a Beckman Type 5430 refrigerated ultracentrifuge (Beckman Instruments). Afterwards, the supernatants were used for the antioxidant indexes assays.
The superoxide dismutase (SOD) activity was assessed by the total superoxide dismutase (T-SOD) assay kit (Hydroxylamine method, A001–1–2, Nanjing Jiancheng Bioengineering Institute), assayed using the xanthine/xanthine oxidase method based on the production of O2- anions. The developed blue colour was measured at 550 nm. The activities of T-SOD were expressed as units per milligrams of protein (U/mgprot).
Malondialdehyde (MDA) content was determined by the MDA assay kit (TBA method, A003–1–2, Nanjing Jiancheng Bioengineering Institute). The optical density at 532 nm was measured via a spectrophotometer (UV-2000, Unico Instrument, Shanghai, China). 1,1,3,3-Tetramethoxypropane was employed as an external standard. The level of MDA was expressed as nanomoles per milligram protein (U/mgprot).
Histological analysis of hippocampus
Cerebral hemispheres of the rats were taken out and fixed with 4 % formaldehyde, embedded with paraffin, subjected to sectioning on microtome (CUT 5062, SLEE medical, Mainz, Germany) and the coronal sections with thickness of 6 mm were obtained. The serial sections (six sections per hippocampus) at an interval of 200 mm between 1·8 and 3·2 mm from bregma were collected. The mounted sections were rinsed with distilled water and stained with alum haematoxylin followed by differentiating with 0·3 % acid alcohol. After rinsing in distilled water, the sections were stained with eosin for 2 min and dehydrated for mounting, which were observed under a microscope (BX53, Olympus).
Expression detection of hippocampus γ-aminobutyric acid receptor 1 and N-methyl-d-aspartic acid 2B receptor
Expressions of GABABR1 and N-methyl-d-aspartic acid 2B receptor (NMDAR2B) were detected by the immunohistochemical techniques. The hippocampus sections were deparaffinised to water, washed with PBS for three times, sealed by dropping serum and placed at room temperature for 20 min. Mouse anti-mouse GABABR1 (Abcam) antibody and rabbit anti-mouse NMDAR2B (Abcam) antibody were added dropwise, placed overnight at 4°C and washed with PBS for three times; Cy3-Goat anti-mouse (Boster) and FITC-Goat anti-rabbit IgG (Boster) were added dropwise, incubated at 37°C for 1 h and rinsed with PBS for three times, while the staining was performed with diaminobenzidine. After the reaction was terminated, the film was dried, mounted and observed by a microscope with the IPP6·0 analysis system (BX53; Olympus Corp.), and the average optical density of positive cells was used as the quantitative expression result.
Statistical methods
All data were expressed as mean ± standard deviation (sd). The data sets were first assessed for equal variance via Bartlett’s test and for normality using the Kolmogorov–Smirnov test. The group means were compared by ANOVA followed by Fisher’s least significant difference post hoc test. The body weight was compared by mixed model analysis. The difference was recognised as statistically significant when P < 0·05. Data analysis was performed by the SPSS 26·0 software (IBM Corp.) and Origin Pro 9.1 (OriginLab Corp.).
Results
Main components of fermentation extract by Bacillus natto
The tested results of the main composition of FE are listed in Table 1.
Table 1. Determined main composition in FE

Evaluation of the ageing model
It can be observed that the rats in group N were in good physical condition, with white and shiny hair, elastic skin, normal diet and rapid movement, while the rats in group M exhibited prominent signs of ageing: emaciation, yellow and dull coat colour, skin laxity, slow movement and listlessness.
Effects of fermentation extract by Bacillus natto on growth performance in the ageing rats
The changes in average body weight of each group during the trial period are shown in Fig. 2(a). As seen, the body weights of the rats in group N were increased gradually from 241·67 g to 275·42 g in the whole experiment period, and the average body weight of the rats in group M showed a rising trend in the first 2 weeks as well, while from the third week onwards, the body weights were decreased gradually with the extension of modelling time which were declined from 240·33 to 235·73 g. The mean body weight of the rats in group FL was not notably changed, while those of group FM, group FH, and group Y were increased gradually by 7·99 %, 12·18 % and 12·75 %, respectively.

Fig. 2. (a) Weight gain of rats in each group; (b) liver index of rats in each group; (c) spleen index of rats in each group; (d) thymus index of rats in each group. N: normal group; M: model group; FL: fermented peanut meal extracts (FE) low-dose group; FM: FE medium-dose group; FH: FE high-dose group; Y: positive control group (VE).
There was no significant difference can be observed in liver index among all groups (P > 0·05). Compared with group N, the spleen index of group M was decreased from 2·35 % to 2·11 %, which was markedly decreased by 10·21 % (P < 0·05), while that of group FM was increased by 9·19 % (P < 0·05), in comparison with group M. The thymus index of group M was declined from 1·71 % to 1·41 %, compared with group N, which was remarkably decreased by 17·54 % (P < 0·05), while those of groups FL, FM and FH were increased by 10·29 %, 16·18 % and 16·67 %, respectively, compared with group M (P < 0·05). It can be inferred from the growth and organ indexes of rats that FE has no harmful effects on the health of the experimental animals.
Effects of fermentation extract by Bacillus natto on cognitive function in the ageing rats
Morris water maze test
The MWM was performed to evaluate the effects of FE on the learning and memory skills of rats. The swimming trajectory of each experimental group during probe tracking is shown in Fig. 3(a), and the last day of the trajectory is displayed in Fig. 3(b). Escape latencies, percentage of time and distance in target quadrant, numbers of crossing over the platform and average swimming speeds (Fig. 3(c)–(g)) were also observed.

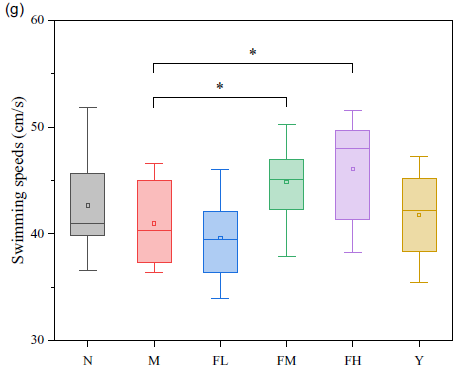
Fig. 3. (a) Swimming trajectory of the rats from each group on the first day; (b) swimming trajectory of the rats in each group on the last day; (c) escape latencies of each group in 5 d training trials; (d) percentage of time in target quadrant on the last day; (e) percentage of distance in target quadrant on the last day; (f) numbers of crossing over the platform on the last day; (g) average swimming speeds on the last day. N: normal group; M: model group; FL: fermented peanut meal extracts (FE) low-dose group; FM: FE medium-dose group; FH: FE high-dose group; Y: positive control group (VE).
As can be seen from Fig. 3(a) and (b), there were no notable differences in the swimming trajectories between all groups on the first day of training, while group M showed a significantly higher proportion of figure ‘8’, large circling movements and longer times than those of the other groups on the last day, indicating that the rats in group M retained a less accurate spatial memory. However, the rats in group FL, FM and FH could quickly find the platform position and exhibited strong spatial memory ability.
To remove the baseline difference, the slope change of escape latency during training of each rat was selected to evaluate the difference between each group, as seen in Fig. 3(c). Compared with group N, the escape latency of the rats in group M was prominently extended by 28·59 %, while those of groups FM and FH were significantly shortened by 66·06 % and 33·46 %, compared with group M (P < 0·05). In terms of time ratio, distance ratio and times of crossing over the platform in the target quadrant, the rats in group M showed markedly different degrees of decline, compared with the rats in group N, which were 52·79, 55·07 and 75·82 %, respectively (P < 0·05). Compared with group M, the time ratios of the rats in groups FL, FM and FH were remarkably risen by 35·43, 85·27 and 123·10 %, respectively (P < 0·05), and the distance ratios of the rats in groups FL, FM and FH were notably elevated by 28·10, 94·48 and 106·19 %, respectively (P < 0·05). As for crossing over the platform, groups FL, FM and FH were significantly increased by 75·19, 288·72 and 363·91 %, respectively, compared with group M (P < 0·05). The swimming speed of the rats in groups FM and FH was significantly ascended by 9·52 and 12·45 %, compared with that in group M (P < 0·05).
Effect of fermentation extract by Bacillus natto on antioxidant activity of the brain tissue in the ageing rats
As shown in Fig. 4, the SOD activity in group M was significantly decreased by 30·22 % from 137·19 U/mgprot to 95·73 U/mgprot, compared with that of group N (P < 0·05), while the activity was increased by 46·25 % and 63·54 % in groups FM and FH, respectively, compared with group M (P < 0·05). The MDA content in group M was markedly raised by 32·76 % from 4·06 U/mgprot to 5·39 U/mgprot (P < 0·05), in comparison with group N. However, compared with group M, the MDA content in groups FM and FH was remarkably declined by 20·39 % and 28·04 %, respectively (P < 0·05).

Fig. 4. Superoxide dismutase (SOD) (a) and malondialdehyde (MDA) (b) of ageing rats brain tissue. N: normal group; M: model group; FL: fermented peanut meal extracts (FE) low-dose group; FM: FE medium-dose group; FH: FE high-dose group; Y: positive control group (VE).
Histomorphological observation of hippocampus
It can be observed from Fig. 5 that, in group N, the hippocampal neurons exhibited a clearly hierarchical and tight array, with large and round light staining nuclei, in which the nucleoli were clear. Comparatively, in group M, the neurons showed a disorganised pattern while its number was reduced, and the outline of nuclei was blurred with a large number of pyknotic degenerated cells, which were shuttle-shaped or triangle-shaped and deeply stained purple blue. In contrast with group M, groups FL, FM and FH displayed a more regular neuronal arrangement, clearer nuclear structure and a reduced number of nuclear pyknotic cells, which was most pronounced in group FH. These results indicate that FE can inhibit d-galactose-induced neuronal degeneration, thereby reducing neuronal damage, and helping to improve the learning and memory ability of the ageing model rats.

Fig. 5. Histological features of H&E staining in hippocampus (original magnifications, ×400). N: normal group; M: model group; FL: fermented peanut meal extracts (FE) low-dose group; FM: FE medium-dose group; FH: FE high-dose group; Y: positive control group (VE).
Expressions of γ-aminobutyric acid receptor 1 and N-methyl-d-aspartic acid 2B receptor in the hippocampus
As seen in Fig. 6(a) and (b), positive expressions of GABABR1 and NMDAR2B can be observed from the results of immunohistochemistry and image analysis, and the cell membrane was stained in yellow.

Fig. 6. (a) Immunohistochemical staining of γ-aminobutyric acid receptor 1 (GABABR1) in hippocampus (×400); (b) Immunohistochemical staining of N-methyl-d-aspartic acid 2B receptor (NMDAR2B) in hippocampus (×400); (c) expression of GABABR1; (d) expression of NMDAR2B. N: normal group; M: model group; FL: fermented peanut meal extracts; (FE) low-dose group; FM: FE medium-dose group; FH: FE high-dose group; Y: positive control group (VE).
It can be noticed from Fig. 6(c) and (d) that the expression of GABABR1 was elevated from 157·40 to 173·00 in group M, in comparison with group N, which was significantly increased by 9·91 % (P < 0·05). And in group FH, the expression of GABABR1 was remarkably descended by 7·98 %, compared with group M (P < 0·05). In contrast with group N, the expression of NMDAR2B was prominently declined by 10·31 %, which was from 157·20 to 141·00 in group M (P < 0·05), and the expression in group FM was markedly raised by 3·83 %, compared with group M (P < 0·05).
Discussion
Ageing is a progressive process in which the function of tissues and organs declines. Its physiological manifestations are mainly reflected in three aspects: morphological changes, such as decrease of cell number and changes in overall appearance; decline of physiological function and function reduction of major sensory organs(Reference Khan, Singer and Vaughan36,Reference Hernandez-Segura, Nehme and Demaria37) . Therefore, the evaluation of ageing is multidimensional. Among the hypotheses of ageing theory, the metabolic theory and free radical theory are generally accepted(Reference Balaban, Nemoto and Finkel38). These two mechanisms are involved in the ageing model prepared by d-galactose, which accumulate in animals by continuous injection of d-galactose and increase intracellular osmotic pressure, eventually causing cell swelling, metabolic disorders and functional disorders(Reference Azman and Zakaria39). Meanwhile, a large number of superoxide radicals can be generated during d-galactose metabolism, resulting in oxidative damage, reducing the immune function of the body, causing degenerative changes in the central nervous system of animals and better simulating the natural ageing situation(Reference Sadigh-Eteghad, Majdi and McCann40). Thus, it is widely used in the pharmacological study of neurodegenerative diseases(Reference Shwe, Pratchayasakul and Chattipakorn41). In this study, the female rats were given subcutaneously 400 mg/(kg/d) of d-galactose daily for eight consecutive weeks. The rats in group M showed obvious signs of ageing, including mental fatigue, hair loss, weight loss and significant decrease of thymus index. Oxidative stress injury was observed in the brain, and the SOD activity was markedly dropped (P < 0·05), while the MDA content, which can reflect the degree of peroxidation, was significantly raised (P < 0·05). The results of MWM experiment show that the spatial learning and memory were declined, and neuronal damage occurred from the HE staining in hippocampal tissue. The immunohistochemical results reveal that the expression of GABABR1 in the hippocampus was remarkably increased (P < 0·05), while the expression of NMDAR2B was notably decreased (P < 0·05). It can be inferred from the above results that the ageing rat model prepared with d-galactose in this experiment was successfully replicated.
The immune system plays an important role in maintaining the stability of the internal environment of the body, ageing is one of the important triggers for reduced function of this system(Reference Müller and Pawelec42). With the growth of age, the immune function declines gradually, which is mainly manifested as the atrophy of immune organs, and the reduction of immune factor level(Reference Rezzani, Franco and Hardeland43), etc. Thymus is the main immune organ of the body, and calculation of thymus index can reflect the immune status to a certain extent(Reference Wang, Lin and Jin44). In this study, the thymus index of the rats in group M was significantly lower than that in group N, indicating that d-galactose could cause degenerative changes in the thymus of the ageing model rats, which was consistent with the literature reports(Reference Sun, Yang and Zhao45,Reference Li, Wei and Guo46) . According to the experimental data, the thymus index of groups FM and FH was notably higher than that of group M. It can be seen that a certain dose of FE can delay the ageing-induced thymic atrophy and exert a certain protective effect on the decline of immune function.
Ageing is an important risk factor for the development of many diseases, and neuroageing is a neurodegenerative disease closely related to age. Neuronal atrophy and neurotransmitter reduction occur during brain ageing, in turn leading to sensory and unresponsiveness, cognitive decline and impaired brain function in the elderly, among which cognitive function is most severely affected by brain ageing(Reference Li, Wang and Li47). Studies have shown that impaired cognitive function due to brain ageing is found to be positively correlated with functional changes in brain regions of the hippocampus and prefrontal cortex by electron tomography(Reference Persson, Sylvester and Nelson48). The main function of hippocampal brain regions is to regulate episodic memory and spatial memory, which plays an important role in memory consolidation. However, it is very fragile, and the structural and functional changes caused by ageing occurred earlier than other brain regions(Reference Bartsch and Wulff49). Previous studies have shown that the hippocampal-related spatial learning and memory function are impaired with ageing(Reference Voss, Soto and Yoo50). The MWM test is a classic behavioural experiment to examine the spatial memory ability of animals(Reference Vorhees and Williams51). In this study, the spatial association and recall exploration ability of the rats in group M were significantly weakened, while the escape latency was markedly prolonged (P < 0·05), indicating that ageing could blur the spatial orientation of the rats and reduce their learning ability, which was similar to the results reported in related literature(Reference Lee, Sur and Han52). At the same time, the swimming ability of the rats supplemented with FE was also compared. The escape latency of group FM and FH was remarkably shortened (P < 0·05), and the crossing times, time and distance ratio were notably increased (P < 0·05) compared with group M, indicating that the medium and high dose of FE can improve the learning and memory ability of the d-galactose-induced ageing rats. Moreover, the HE staining of the hippocampus showed that the nerve cells of the ageing rats supplemented with FE exhibited a tendency of recovery, and improved the expression changes of GABABR1 and NMDAR2B in the hippocampus. These results suggest that FE can exert a protective effect on d-galactose-induced neuronal injury in the rat hippocampus, which in turn enhances its learning and memory ability.
The brain has the highest oxygen demand in body and a weak antioxidant mechanism; therefore, it is highly susceptible to oxidative stress. Excessive reducing sugars can be converted into aldose and hydrogen peroxide in the body, producing reactive oxygen species and thus inducing oxidative stress(Reference Cobley, Fiorello and Bailey53). Normally, the body can ensure homeostasis by maintaining the generation of reactive oxygen species and the balance of antioxidant enzymes such as SOD; however, the decrease in the activity of antioxidant enzymes in the brain and the generation of excessive oxidative products may lead to cell tissue damage and physiological dysfunction of the brain, thus affecting learning and memory function(Reference Haider, Liaquat and Shahzad54,Reference Li, Yu and Zhang55) . Oxidative stress plays a key role in the ageing process of tissues and organs, thus the detection of oxidative stress markers in vivo can indirectly reflect the ageing degree of the body(Reference Moloney and Cotter56). SOD is the most important antioxidant enzyme in organisms, which has the effects of scavenging superoxide anion-free radical, anti-inflammatory and anti-ageing, etc., and the level of SOD activity can directly reflect the antioxidant capacity of tissues(Reference Tu, Chang and Chou57). When the activity of SOD decreases, the ability of body to eliminate oxygen-free radicals is weakened, which can induce the damage of DNA, protein and enzyme, thereby accelerating the ageing of various tissues and organs. Excessive reactive oxygen species production by d-galactose can also accelerate the decomposition of biological tissues, leading to the increase of MDA concentration in the body. MDA is also an important marker of oxidative damage(Reference Zhang, Xu and Wang58), and the measurement of MDA in tissues can objectively reflect the content of lipid peroxide in the body. Excess MDA accumulation has been shown to cause impaired cognitive function(Reference Liu, Liu and Tu59). Therefore, in this study, SOD and MDA, the biomarkers most closely related to oxidative stress, were detected to evaluate the anti-ageing effect of B. natto fermented peanut meal on the d-galactose-induced ageing model rats. The results showed that the antioxidant capacity of the rats in group M was declined. However, the SOD activity was elevated, while the MDA content was decreased in the brains of the rats supplemented with FE, indicating that FE could enhance the activity of antioxidant enzymes in the brains of the ageing model rats, and slow down the damage of free radicals to the brain cells, showing the anti-ageing effect. Natural antioxidant substances can raise the SOD activity and enhance the oxygen-free radical scavenging and antioxidant damage ability. It can be speculated from the experimental data that FE can slow down the damage of free radicals to the brain cells by enhancing the SOD activity in the brain tissues of the ageing rats, the mechanism of which may be lied in the polyphenols, peptides and polysaccharides contained in FE.
In summary, FE can delay the ageing process of the d-galactose-induced female ageing model rats, which may be related to two aspects: on the one hand, it can improve the body’s immunity and reduce the atrophy of the immune organs; on the other hand, it can enhance the antioxidant capacity of the body. By suppressing the oxidative stress response caused by the d-galactose intervention, FE enhances the antioxidant level of the body and reduces the damage of the brain tissue, which may be a key factor in preventing cognitive decline in the rats. Nonetheless, in-depth study still should be done to clarify the exact components of FE that can delay ageing and improve the learning and memory abilities in vivo and further explore their possible mechanisms of action.
Conclusion
Based on the above experimental results, we can infer that FE helps to improve the growth performance of the ageing rats, enhance the learning and memory ability and delay ageing. Its mechanism of action may be due to the delay of brain ageing by reducing the expression of GABABR1, increasing of the antioxidant activity for the brain, prevention of neuronal degeneration and protection of the hippocampus. This study provides evidence for the anti-ageing effect of FE and a reference for the study of the potential nutritional value of by-products of peanut processing industry.
Acknowledgements
The authors wish to thank Dr. Zhantao Liu from the school of pharmacy in Qingdao University for the use of some instruments and assistance with the measurements.
This work was financially supported by the Qingdao Science and Technology Project (20-3-4-33-nsh) and Shandong Provincial Natural Science Foundation (ZR2019PH020).
Conception and design of the study: Z. L. and A. M.; experiments: H. D., Q. L., Y. Z. and Y. W.; collection of data: H. D., Q. L. and Y. Z.; analysis and interpretation of data: H. D., Q. L. and Y. Z.; drafting of the manuscript: H. D. and Y. H. and critical revision of the manuscript for important intellectual content: A. M., Z. L. and Y. H.
The authors declare that there are no conflicts of interest.