The COVID-19 epidemic
As epidemiological data on the dramatic COVID-19 pandemic are disseminated, obesity has emerged as a major risk factor for severe disease necessitating intensive care unit (ICU) admission(Reference Richardson, Hirsch and Narasimhan1–Reference Lemyze, Courageux and Maladobry5). Obese patients <60 years old have a 2·2-fold increased rate of hospitalisation with coronavirus disease 2019 (COVID-19)(Reference Lighter, Phillips and Hochman2), while a 7·36 increased risk of mechanical ventilation has been reported in patients with BMI 35 kg/m2 or greater(Reference Simonnet, Chetboun and Poissy4). The observation of worse outcomes in obese patients was also seen during the 2009 H1N1 influenza pandemic, with 61 % of fatalities occurring in obese adults(Reference Louie, Acosta and Samuel6). The apparent increased severity of viral pneumonia in this population is critically important given the increasing prevalence of obesity globally(7). Multiple potential explanations for this association have been proposed, including altered pulmonary physiology in the obese patient, predisposition to thromboembolic complications which are observed with increased frequency in COVID-19 patients and increased incidence of co-morbidities that may impact upon outcome and render ICU management more complex and challenging(Reference Lemyze, Courageux and Maladobry5,Reference Sattar, McInnes and McMurray8,Reference Chiappetta, Sharma and Bottino9) . However, a growing body of evidence implicates direct obesity-induced immune dysregulation. Increased lethality of H1N1 influenza observed in obese mice has been linked to impaired T-cell responses(Reference Milner, Sheridan and Karlsson10), while failed influenza vaccine response is correlated with impaired antibody generation(Reference Karlsson, Hertz and Johnson11). Likewise, human studies have demonstrated failure of influenza vaccine response in obese adults(Reference Sheridan, Paich and Handy12). Similarly, increased susceptibility of obese patients to severe COVID-19 infection is hypothesised to be linked to impaired successful immune responses against the virus(Reference Kim and Nam13), particularly through diet-derived reconfigurations of protective immunometabolic responses.
Obesity and immune dysfunction
While obesity has been linked to dysregulated adaptive immunity and failure of antibody generation following infection or vaccination, severe acute respiratory syndrome coronavirus-2 (SARS-CoV-2) is a novel virus to which no one has an established adaptive immune response upon first exposure. Initial host defence against a novel virus relies entirely upon the rapid, non-specific innate immune response(Reference Chen, Liu and Goraya14). Thus, the impact of obesity upon innate immunity likely plays a crucial role in the severity of COVID-19 disease. Obesity creates a state of chronic inflammation, termed ‘meta-flammation’, characterised by sustained pro-inflammatory innate immune responses mediated through activation of the NLR family pyrin domain-containing 3 (NLRP3) inflammasome/IL-1 axis(Reference Ghanim, Aljada and Hofmeyer15–Reference Larsen, Faulenbach and Vaag19). Adipose tissue directly secretes pro-inflammatory TNFα, IL-1 and IL-6, with downstream activation of the transcription factor NF-κB, driving a state of oxidative stress(Reference Divella, De Luca and Abbate20). This meta-flammation underpins the pathogenesis of several obesity-related conditions, including type 2 diabetes mellitus, CVD and non-alcoholic fatty liver disease(Reference Wen, Gris and Lei21,Reference Wree, McGeough and Peña22) . Similarly, the establishment of ‘trained immunity’, a non-specific priming of progenitor cells of the innate immune system which alters their functional responses, is influenced by meta-flammation associated with these conditions(Reference Netea, Joosten and Latz23,Reference Christ, Gunther and Lauterbach24) .
Despite increased basal levels of pro-inflammatory mediators, obesity-associated meta-flammation is paradoxically associated with attenuated innate immune response to both bacterial and viral infections(Reference Honce and Schultz-Cherry25,Reference Huttunen and Syrjanen26) . Diet-induced obese mice demonstrate a substantially higher mortality rate than lean mice following influenza infection, with reduced expression of pro-inflammatory cytokines TNFα, IL-6, IL-1β and interferon-1 in the pulmonary compartment early post-infection(Reference Smith, Sheridan and Harp27,Reference Easterbrook, Dunfee and Schwartzman28) . Interestingly, however, by day 6 post-infection, the lungs of obese mice contained higher expression of TNFα and IL-1β, with similar expression of anti-inflammatory IL-10 to that of lean mice, suggesting a ‘skewing’ towards a pro-inflammatory state that results in lung tissue damage(Reference Smith, Sheridan and Harp27). One possible explanation for this observation is failure of early macrophage responses in the obese mouse resulting in insufficient viral control by the innate immune system, with later T-cell infiltration causing increased inflammation and tissue damage that contributes to pathogenesis. Lipopolysaccharide-induced obese mice demonstrated impaired macrophage production of TNFα, IL-1β and IL-6 when challenged, with more severe lung pathology following infection with H1N1 influenza(Reference Ahn, Sohn and Lee29). This mirrors the pathogenesis observed in severe COVID-19 disease, where early localised host responses fail to control viral replication, with later surges in inflammation – the ‘cytokine storm’, particularly driven by IL-6, which is associated with increased mortality(Reference Wu, Chen and Cai30) – causing overt tissue damage that ultimately leads to respiratory failure. Elevated leptin levels characteristic of obesity may also contribute, with a murine model of 2009 H1N1 influenza infection demonstrating reduced IL-1 and IL-6 levels following administration of anti-leptin antibody to obese mice, resulting in improved survival(Reference Milner, Sheridan and Karlsson10). Interestingly, though attenuated levels of pro-inflammatory cytokines were evident in the pulmonary compartment early post-infection in obese mice, systemic levels of TNFα, IL-1 and IL-10 were elevated at early time points similar to that of lean mice(Reference Easterbrook, Dunfee and Schwartzman28).
Macrophage metabolism and bioenergetics
We have previously described a similar state of baseline hyper-inflammation but paradoxical failure to mount an adequate innate immune response to infection in smokers’ pulmonary macrophages compared with those of non-smokers’(Reference O’Leary, Coleman and Chew31). Upon stimulation, healthy human pulmonary macrophages undergo a dramatic shift in central carbon metabolism, termed glycolytic reprogramming, with increased reliance on glycolytic metabolism rather than oxidative phosphorylation to generate energy(Reference Gleeson, Sheedy and Palsson-McDermott32), resulting in activation of the NLRP3/IL-1 axis through consequent impact on transcription factor expression, diversion of metabolic intermediates into biosynthetic pathways and perturbations of the electron transport chain(Reference Gleeson and Sheedy33,Reference Mills, Kelly and Logan34) . Similarly, ‘trained immunity’, the protective effect of which is being examined in the context of COVID-19 due to Bacillus Calmette–Guérin vaccination(Reference Gursel and Gursel35), requires such glycolytic reprogramming in progenitor innate immune cells(Reference Netea, Joosten and Latz23).
Smokers’ macrophages, in contrast to non-smokers’, are skewed towards increased glycolytic activity at baseline(Reference O’Beirne, Kikkers and Oromendia36,Reference Gleeson, O’Leary and Ryan37) , with elevated IL-1 production, similar to the chronic hyper-inflammation observed in innate immune cells from obese patients. Despite this, however, smokers’ macrophages are unable to ramp up glycolytic activity and consequent pro-inflammatory and anti-microbial functions in response to pathogenic challenge, due to a state of ‘bioenergetic exhaustion’ induced by this chronic baseline activity(Reference Gleeson, O’Leary and Ryan37). Others have also demonstrated increased ‘polarisation’ of macrophages from smokers lungs at baseline(Reference Bazzan, Turato and Tinè38), notably describing increased proportions of M1-skewed macrophages, known to characteristically favour glycolytic metabolism. Interestingly, analyses of bronchoalveolar lavage fluid from SARS-CoV-2-infected patients have similarly identified higher proportions of recruited M1-like macrophages and depletion of lung-native tissue resident alveolar macrophages (which preferentially use oxidative phosphorylation to generate energy) in those with more severe disease(Reference Salomé and Magen39). Though baseline BMI was not reported in this study, strong epidemiological evidence suggests severe disease to be more prevalent in obese patients(Reference Richardson, Hirsch and Narasimhan1,Reference Lighter, Phillips and Hochman2) and existing studies have shown that obesity-associated metabolic signals alter tissue resident macrophage phenotype away from the classical anti-microbial programme(Reference Kratz, Coats and Hisert40).
The impact of obesity on innate immune responses
Although many features of obesity can exhaust immune function through hyper-stimulation, ranging from hyperglycaemia, insulin resistance to hyperlipidaemia, we must consider the differential effect these factors have at various levels of innate immune function. Exhaustion of anti-microbial responses in resident lung macrophages could be coupled with hyper-stimulation or training of the immune system at the level of the bone marrow and circulating monocytes, which traffic to the lung after infection and trigger excessive inflammatory responses. Blood glucose control has been implicated in COVID-19 severity, with hyperglycaemic patients experiencing worse disease(Reference Zhu, She and Cheng41). Thus, the effect of this increased glucose on immunometabolic functions is being examined. Ongoing and unpublished work examining the importance of glycolysis in circulating monocytes after SARS-CoV-2 infection indicates that excessive glycolysis may actually facilitate viral replication and drive excessive disease inflammation once recruited to the lung(Reference Cavounidis and Mann42). At the same time, recent murine studies of resident lung macrophages, however, suggest these cells preferentially use fatty acid oxidation rather than glycolysis to adapt to the lipid-rich and glucose-deprived environment in the lung(Reference Svedberg, Brown and Krauss43,Reference Woods, Kimmig and Meliton44) . Strategies which boost glycolysis and pro-inflammatory function specifically in these cells could be used to improve early anti-viral containment. Additionally, obesity has also been shown to alter the expression of the receptor for SARS-CoV-2 entry on respiratory epithelia, with retrospective analysis of angiotensin-converting enzyme-2 (ACE2) mRNA demonstrating increased expression in lung epithelia in obese high-fat diet-fed mice(Reference Heialy, Hachim and Senok45). Thus, obese patients could suffer from a trifecta of immunometabolic defects at level of lung epithelium (primary infected cell), the alveolar macrophage (the primary responding cell) and circulating monocytes (the primary recruited cell), which increase their susceptibility to disease (Fig. 1).
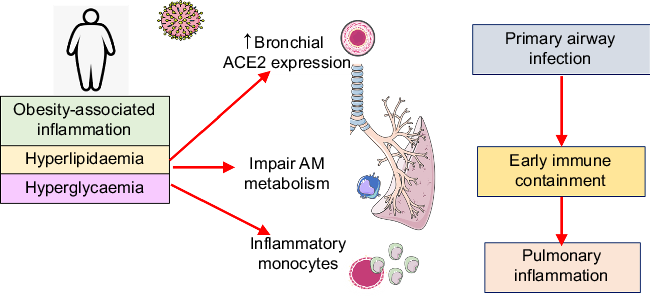
Fig. 1. The trifecta of immunometabolic defects in the innate immune response to coronavirus disease 2019 (COVID-19) in obese people. Increased expression of angiotensin-converting enzyme-2 (ACE2), the receptor facilitating severe acute respiratory syndrome coronavirus-2 (SARS-CoV-2) infection, has been reported in the respiratory tract and lung of obese mice. Alveolar macrophage (AM) metabolism is altered by the lung environment and can be exhausted by baseline chronic inflammation affecting early microbial containment. Circulating bone marrow-derived monocytes can be affected by coincidental adverse obesity-related metabolic stressors, for example, poor blood glucose control, hyperlipidaemia. Resultant immune training in the bone marrow monocyte lineage leads to a hyperinflammatory phenotype that could facilitate up-regulation of disease-causing inflammation in the lung. This figure was created using Servier Medical ART templates, which are licensed under a Creative Commons Attribution 3.0 Unported License; https://smart.servier.com
Obesity-associated inflammation and dietary quality fat intake
Obese phenotypes vary greatly, which may be partly attributable to diet quality and/or physical activity(Reference Yubero-Serrano, Delgado-Lista and Tierney46). There is clear evidence that NLRP3 inflammasome-mediated IL-1β inflammation influenced by obesity has been outlined above. However, it is possible that this paradigm is more complex and linked to the quality/composition of the obesogenic diet. For example, the nature of different fatty acids and/or their metabolites may play a role. Excessive dietary fat presented as SFA greatly accentuates obesity-induced metabolic inflammation(Reference Murphy, Smith and Murphy47,Reference Finucane, Lyons and Murphy48) . In contrast, feeding excessive MUFA or PUFA do not accentuate NLRP3 activity or IL-1β inflammation to same extent, possibly via re-configuration of key metabolic hubs including AMP-activated protein kinase(Reference Finucane, Lyons and Murphy48,Reference Ralston, Nguyen-Tu and Lyons49) . We posit that certain dietary elements, such as SFA, fructose-induced endogenous SFA and/or their metabolites including ceramides may be particularly deleterious within the context of obesity-related immunometabolic dysfunction. And whilst hypothetical, this may in turn determine not only the nature of the innate immune response in COVID-19 but also the efficacy of vaccination at the population level(Reference Karlsson, Hertz and Johnson11,Reference Sheridan, Paich and Handy12) .
There are significant gaps in knowledge with respect to the impact of different dietary elements on COVID-19-related immunity (as recently reviewed(Reference Calder50)) and within the context of obesity. To this end, we need to be cautious and proceed with sound nutritional evidence. One case in point being the recent controversy wherein some observational studies linked low vitamin D status, which often coexists with obesity, to adverse COVID-19 outcomes. It is probable that vitamin D, alongside other important nutrients, plays an important role in immune cell metabolism and related immune functionality, as most cells can convert the vitamin D precursor into its active form. This does not imply that vitamin D supplementation in high-risk obese subjects can reduce risk. There are too many confounders underlying associations, not least of which vitamin D status is simply a good biomarker for several micronutrient deficiencies, contributing to malnutrition despite obesity.
Future perspectives
The innate immune response to SARS-CoV-2 infection is likely crucial in determining the clinical course of COVID-19 disease, and obesity-associated dysregulation of this response is critically important given the high prevalence of this co-morbidity worldwide. While understanding the direct impact of obesity upon COVID-19 pathogenesis is important, from the diet and lifestyle perspective, there may be additional elements we could better understand which impact innate immune function at multiple levels. Based on parallels that can be drawn with our findings in smokers’ pulmonary macrophages, metabolic characteristics of innate immune response in the obese patient potentially represent a key feature of the unique susceptibility of this population to severe disease. As we struggle to develop therapies and vaccines to combat this new global health threat, immunometabolism of the innate immune system warrants attention from the global scientific community.
Acknowledgements
L. E. G. is an acting consultant in respiratory and general internal medicine at St James’ Hospital. H. M. R. is funded by the Irish Department of Agriculture, Food and the Marine (14/F/828 “ImmunoMet” Programme) and Precision Oncology Ireland (18/SPP/3522) which is part funded by the Science Foundation Ireland Strategic Partnership Programme. F. J. S. and H. M. R. are supported by the Science Foundation Ireland Frontiers Award Programme (SFI 19/FFP/6625).