Preterm delivery (PTD; delivery before completing 37 weeks of gestation) affects 5–13 % of all pregnancies worldwide, though the incidence varies a lot even between high-income countries(Reference Morken, Vogel and Kallen1–Reference Chang, Larson and Blencowe3). It is the leading cause of neonatal morbidity and mortality. There is no way of predicting or preventing spontaneous PTD, but it is urgent to identify modifiable factors influencing the prevalence of PTD and to understand the pathways that regulate the timing of birth.
Our research group recently performed a genome-wide association study regarding gestational length and PTD in a discovery cohort of 43 568 women of European ancestry(Reference Zhang, Feenstra and Bacelis4). Four loci achieved genome-wide significance: the eukaryotic elongation factor, selenocysteine transfer RNA-specific (EEFSEC) gene was significant for both gestational length and risk of PTD. These associations were confirmed in three Nordic cohorts, among others the Norwegian Mother and Child Cohort Study (MoBa).
The EEFSEC gene codes for the protein EEFSEC, which is an elongation factor necessary for the co-translational incorporation of Se into the amino acid selenocysteine and thus selenoproteins. Se is an essential trace element. Its biological function is transferred via some twenty-five selenoproteins, and Se deprivation limits the syntheses of these proteins. Selenoproteins, such as the glutathione peroxidases and the thioredoxin reductases, have important cellular homeostatic functions in maintaining redox status and antioxidant defence as well as in modulating inflammatory responses(Reference Labunskyy, Hatfield and Gladyshev5). The Se-containing 5’deiodinases, which are required for thyroid hormone activity, are important in the regulation of growth and energy metabolism(Reference Mullur, Liu and Brent6). Redox status, inflammation and regulation of energy metabolism have been linked to the parturition process and risk of PTD(Reference Burnum, Hirota and Baker7–Reference Chen, Chen and Lin10). The identification of the selenocysteine pathway in PTD suggests that maternal Se status may be important. The fact that Se deficiency is prevalent in Malawi, the country with the highest frequency of PTD(11,Reference Hurst, Siyame and Young12) , further supports the hypothesis. The few existing studies on Se metabolism with regard to gestational length present contradicting results. Data from the National Birth Defects Prevention Study on 5738 deliveries (471 PTD cases) found no relationship between Se intake before pregnancy, measured in retrospect, and the risk of PTD (Reference Carmichael, Yang and Shaw13). Similarly, a small observational study (n 233) in the USA found no association between Se concentration in plasma and PTD(Reference Bogden, Kemp and Chen14). However, the study found that women delivering preterm (n 107) had higher estimated Se intake during early pregnancy compared with women delivering at term (n 126)(Reference Bogden, Kemp and Chen14). A third observational study found that women who delivered preterm (n 60) on the contrary had lower serum Se concentration at gestational week 12 compared with those who delivered at term (n 1069)(Reference Rayman, Wijnen and Vader15).
The aim of the present study is to examine the association between self-reported maternal dietary intake of Se during the first half of pregnancy and maternal Se status as indicated by the whole-blood concentration of Se at week 17 and two outcomes, gestational length and spontaneous PTD, in the large, prospective population-based MoBa.
Subjects and methods
Study population
The present study is based on MoBa, an ongoing prospective population-based pregnancy cohort administered by the Norwegian Institute of Public Health(Reference Magnus, Birke and Vejrup16). Participants were recruited from all over Norway from 1999 to 2008, with a participation rate of 41 %. The cohort now includes 114 500 children, 95 200 mothers and 75 200 fathers(Reference Magnus, Birke and Vejrup16).
Pregnant women were invited by postal invitation in connection with the routine ultrasound screening offered free of charge to all women in gestational weeks 17–19. They were asked to answer three questionnaires during their pregnancies and to donate blood and urine samples at the time of ultrasound screening. Participants were followed up regularly with questionnaires after delivery. The present study is based on information from the first questionnaire (Q1) about general health status and lifestyle, which was filled out around gestational weeks 15–17, and the semi-quantitative FFQ filled out around gestational week 22. Pregnancy and birth records from the Medical Birth Registry of Norway (MBRN) were linked to the MoBa database(Reference Magnus, Birke and Vejrup16). MBRN is a national health registry containing information about all births in Norway. The present study is based on version 8 of the quality-assured data files released for research in 2014. Only women with singleton live births after gestational week 22+0 and women with valid estimates of total energy intake (between 4·5 and 20 MJ) were included. Women who participated in the cohort with more than one pregnancy were included only with their first enrolled pregnancy. Fig. 1 outlines the selection of the study population.

Fig. 1. Flow chart over the study population. * The present FFQ was not used before 2002, explaining the drop of numbers from boxes 3 to 4. † Selenium was measured in n 2982 women. MoBa, Norwegian Mother, Father and Child Cohort Study.
Selenium intake
Intake of Se (µg/d) from food and dietary supplements was estimated based on self-reported food and supplement intake in the MoBa (FFQ). The MoBa FFQ is a semi-quantitative instrument designed to record dietary habits and intakes of dietary supplements during the first 4–5 months of pregnancy, that is, from the start of pregnancy until around gestational week 22(Reference Meltzer, Brantsaeter and Ydersbond17). The FFQ includes questions about the intake of 255 food items or dishes. The FFQ has been validated in an MoBa subpopulation (n 119) using a 4-d weighed food diary and biological markers in blood and urine as reference measures(Reference Brantsaeter, Haugen and Thomassen18, Reference Brantsaeter, Haugen and Alexander19), showing that the MoBa FFQ is a valid tool for assessing dietary intake of energy, nutrients and food in the first 4–5 months of pregnancy.
The present version of the FFQ was used from March 2002 throughout the recruitment period. Completed questionnaires were optically read, and the consumption frequencies were converted into food amounts (g/d) using standard Norwegian portion sizes(Reference Meltzer, Brantsaeter and Ydersbond17). Intake of Se and other nutrients from food was based on FoodCalc(Reference Lauritsen20) and the Norwegian food composition table(Reference Rimestad, Borgejordet and Vesterhus21). Se occurring naturally in foods is thought to be mostly selenomethionine.
The participants were asked to report the use of dietary supplements by writing name and brand as well as the frequency and amount. To calculate nutrient intake from supplements, a database of more than 1000 dietary supplements was created based on the producers’ declared nutrient content information(Reference Haugen, Brantsaeter and Alexander22, Reference Brantsaeter, Haugen and Hagve23). Available Se supplements contained one or more forms of Se, including inorganic selenite or selenate, selenomethionine, Se-methylselenocysteine or selenised yeast(Reference Niedzielski, Rudnicka and Wachelka24). These forms differ in their impact on tissue Se concentration(Reference Sigrist, Brusa and Campagnoli25). The supplementary Se intake was estimated and analysed separately for inorganic and organic supplements.
Selenium status
A subgroup of the MoBa participants (n 2999) was also included in the Norwegian Environmental Biobank, a new project established by the Norwegian Institute of Public Health(Reference Paltiel, Haugan and Skjerden26, Reference Caspersen, Thomsen and Haug27). Inclusion criteria for the Norwegian Environmental Biobank were available whole blood, urine and plasma samples, available genetic data and available data from MoBa questionnaires 1–6 and the father questionnaire(Reference Paltiel, Haugan and Skjerden26, Reference Caspersen, Thomsen and Haug27). Whole blood was collected in heparin tubes in gestational weeks 17–18 and shipped by ordinary mail (unrefrigerated) in a Vacutainer for long-term freezing at a central bio-repository(Reference Ronningen, Paltiel and Meltzer28, Reference Paltiel, Anita and Skjerden29). Storage temperature for whole blood was –20°C(Reference Caspersen, Thomsen and Haug27). Se analyses were conducted at Lund University, Sweden, by inductive coupled plasma MS (iCAP Q; Thermo Fisher Scientific, Bremen, GmbH) equipped with a collision cell having kinetic energy discrimination and helium as collision gas. The detection limit was 3·2 µg/l, and the CV was 1·5 %. The analytical accuracy was verified using certified reference materials, Seronorm Trace elements whole blood L-1 and L-2 (SERO AS, Billingstad, Norway). The results obtained were represented as mean (standard deviation (sd)) were for L-1 (Lot 1103128, N 205) 56·1 (5·7) μg/l v. recommended 59 (35–83) μg/l and for L-2 (Lot 1103129, N 205) 116 (1·5) μg/l v. recommended 112 (66–158) μg/l(Reference Caspersen, Thomsen and Haug27). Blood Se reflects both status and uptake while plasma/serum Se only reflects short-term status(Reference Thomson30).
Se measurements were available for 2637 of the 72 025 women included in the present study.
Outcome variables
Gestational length in days was determined in second trimester using ultrasound in 98·2 % of the pregnancies and based on last menstrual period in the remaining pregnancies. PTD was defined as birth between week 22+0 and 36+6. Early PTD was defined as delivery between week 22+0 and 33+6, late PTD as week 34+0 and 36+6, early term as 37+0 and 38+6 and late term as deliveries after week 39+0. Spontaneous PTD was defined as birth after preterm labour or preterm pre-labour rupture of membranes.
Covariates
All multivariate models with Se intake (from food or from supplements) as exposure variable were adjusted for the following pre-defined covariates: maternal age, parity, maternal smoking habits during pregnancy, alcohol consumption, maternal education, pre-pregnancy BMI, iodine intake, protein intake, fibre intake, n-3 intake and total energy intake. Further, models were mutually adjusted for the different Se sources (dietary intake, organic supplements, inorganic supplements). Multivariate models with Se status as exposure variable were adjusted for maternal age, parity, maternal smoking habits during pregnancy, alcohol consumption, maternal education and pre-pregnancy BMI.
Information regarding maternal age at delivery was obtained from MBRN and used as a continuous variable in the regression models. Information on parity was based on the data from both MBRN and the first MoBa questionnaire (Q1) that were distributed in pregnancy week 15–17 and divided into categories based on the number of previous pregnancies of ≥22+0 weeks of gestation: 0, 1 or >1 previous births. All other covariates were obtained from the MoBa questionnaire Q1. Maternal education was categorised as <13, 13–16, >16 years or missing. Smoking during pregnancy was categorised as non-smoker, occasional or daily smoker. Consumption of alcohol-containing beverages was self-reported in the FFQ (glasses per d, week or month) and dichotomised (yes or no) in the analyses. Pre-pregnancy BMI was based on the self-reported pre-pregnancy height and weight. BMI was grouped according to WHO classification as underweight (<18·5 kg/m2), normal-weight (18·5–24·9 kg/m2), overweight (25·0–29·9 kg/m2) and obese (≥30·0 kg/m2). Dietary fibre was included as a proxy for a healthy diet. We have previously reported that the ‘healthy/prudent dietary pattern’ correlated with fibre intake (r 0·57)(Reference Englund-Ögge, Brantsæter and Sengpiel31). The study population has been shown to be moderately iodine deficient(Reference Abel, Korevaar and Erlund32, Reference Abel, Ystrom and Caspersen33), and iodine has also been found to be associated with PTD(Reference Charoenratana, Leelapat and Traisrisilp34). As dietary iodine and Se intake correlated with r 0·57, and both are important for thyroid hormone production, iodine intake ranked into quintiles was included in the models. Models were also adjusted for dietary n-3 intake since Se and n-3 are both abundant in marine foods and n-3 intake also has been found to decrease the risk of PTD and increase pregnancy length(Reference Middleton, Gomersall and Gould35, Reference Vinding, Stokholm and Sevelsted36). All models were adjusted for total energy intake in kilo Joules (kJ) as well as for total protein intake since many Se sources are protein rich such as meat, poultry and fish. All variables extracted from the MoBa questionnaires were used as categorical variables in the regression models, with missing data as a category of its own.
Statistical methods
All statistical analyses were performed using SPSS Statistics version 25.0 (International Business Machines Corp., IBM). All P values were two sided and values <0·05 were considered significant. Spearman’s correlation test was used to evaluate the correlation between Se intake and blood Se concentration (Table 1). Differences in Se intake and Se status according to maternal characteristics were studied with the Kruskal–Wallis test (Table 2). Multiple linear regression analysis (using the UNIANOVA command in SPSS) was used to analyse the association between Se intake or Se status and gestational length as a continuous variable (Tables 3 and 5). The association between Se intake/status and PTD was estimated as a hazard ratio (HR) with a 95 % CI using multivariable Cox regression (Tables 4 and 6). All analyses were performed with and without adjustment for the confounders listed previously. A sub-analysis was performed in order to study the association between Se intake and different categories of PTD (early preterm, late preterm and early term) (online Supplementary Table S1). In order to study the possible threshold effects between Se intake and PTD, Se intake from diet was analysed in deciles (online Supplementary Table S2). The category closest to the recommended daily intake (RDI; 60 µg/d) was used as the reference. Regarding power, in the analysis based on dietary Se intake, the group sizes are very large (3618 PTD v. 68 407 term deliveries) and hence even small differences can be detected. For Se status based on the subgroup with blood samples, the groups sizes are smaller (80 v. 2558). Here, a difference between PTD and term deliveries of 0·32 Cohen’s d can be detected with 80 % power at a significant level of 0·05.
Table 1. Correlation between selenium intake and whole-blood concentration of selenium
(Numbers of subjects; medians and interquartile ranges (IQR); correlations and 95 % confidence intervals)

* Spearman correlation.
Table 2. Maternal dietary selenium intake and maternal blood selenium concentration according to maternal characteristics†
(Numbers of subjects and percentages; medians and interquartile ranges (IQR))
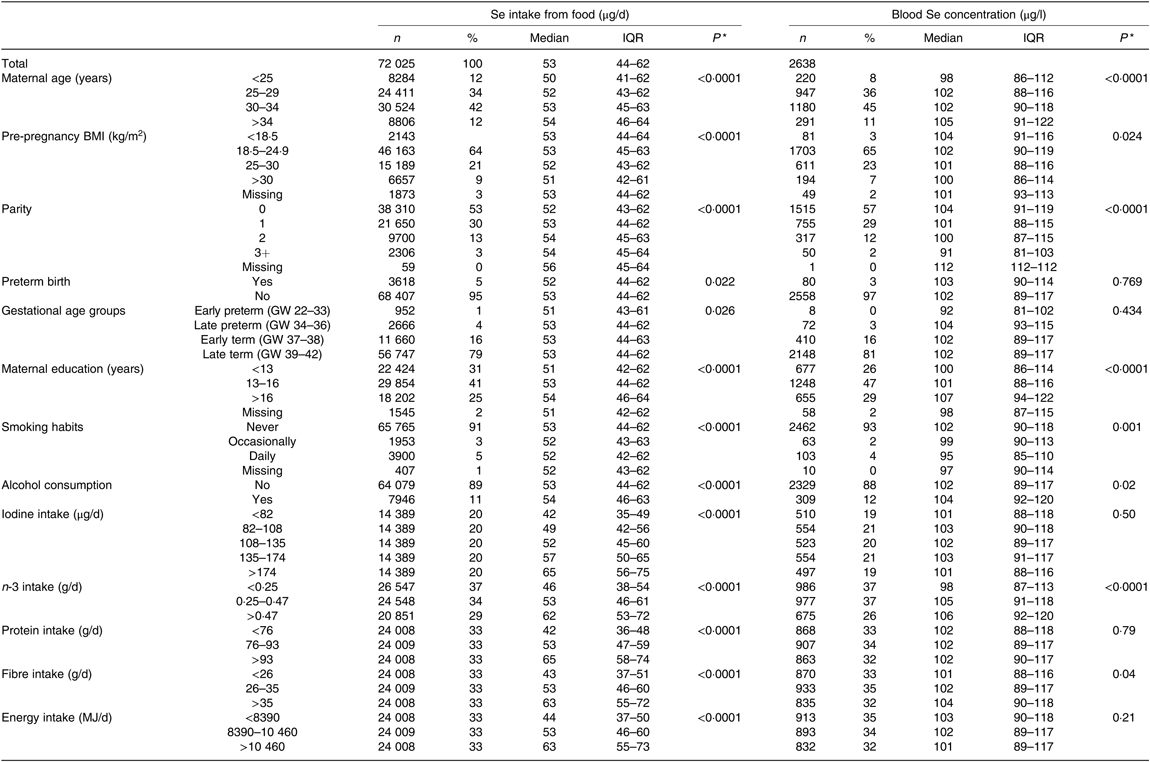
GW, gestational week.
* P value obtained with the Mann–Whitney U test for two groups and the Kruskal–Wallis test for more than two groups.
† Amount of daily Se intake from food (FFQ data) and concentration of Se in blood in mid-pregnancy, according to maternal characteristics, from 72 025 participants in the Norwegian Mother and Child Cohort Study. Se intake from food was assessed with an FFQ in gestational week 22. Blood Se concentration was measured in whole blood collected in GW 17–18 in a subsample of 2638 of the 72 025 participating mothers.
Table 3. Association between maternal dietary selenium intake and selenium intake from supplements and gestational length*
(β-Coefficients and 95 % confidence intervals)

RDI, recommended daily intake.
* Multiple linear regression analysis of Se intake from food and from supplements in relation to gestational length in days for 72 025 participants in the Norwegian Mother and Child Cohort Study. Se intake from food and supplements was assessed with an FFQ in gestational week 22.
† Adjusted for maternal age, parity, smoking habits, alcohol consumption during pregnancy, maternal education, BMI, iodine intake in five categories, fibre intake, protein intake, n-3 intake and total energy intake. Analyses for the different Se sources are also mutually adjusted in the adjusted model.
‡ β per standard deviation of Se intake. Standard deviation for Se intake from food is 14·6 μg/d, from inorganic supplements 32·6 μg/d and from organic supplements 10·0 μg/d.
§ Measured in μg/d.
‖ RDI of Se for pregnant women is 60 μg/d.
Table 4. Association between maternal dietary selenium intake and selenium intake from supplements and risk of preterm delivery*
(Hazard ratios (HR) and 95 % confidence intervals)

* Daily intake of Se from food and from supplements and HR for preterm delivery (22+0–36+6 weeks) in all subjects and in spontaneous deliveries only analysed with Cox regression. Number of preterm deliveries: 3618 of all 72 025 subjects and 2100 of the 57 098 spontaneous deliveries. Se intake from food and supplements was assessed with an FFQ in gestational week 22.
† Adjusted for: maternal age, parity, smoking habits, alcohol consumption during pregnancy, maternal education, BMI, iodine intake in five categories, fibre intake, n-3 intake and total energy intake. Analyses for the different Se sources are also mutually adjusted in the adjusted model.
‡ HR per standard deviation of Se intake. Standard deviation for Se intake from food is 14·6 μg/d, from inorganic supplements 32·6 μg/d and from organic supplements 10·0 μg/d.
§ Measured in μg/d.
‖ Iatrogenic deliveries have been censored in the regression model.
Table 5. Association between maternal selenium blood concentration in mid-pregnancy and gestational length*
(β-Coefficients and 95 % confidence intervals)

* Multiple linear regression analysis of blood Se concentrations in relation to gestational length in days for 2638 participants in the Norwegian Mother and Child Cohort Study. Blood Se concentration was measured in whole blood collected in gestational week 17−18 in a subsample of 2638 of the 72 025 participating mothers.
† Adjusted for: maternal age, parity, smoking habits, alcohol consumption during pregnancy, maternal education and BMI.
‡ β per standard deviation (23·4 μg/l) of blood Se concentration.
§ Measured in μg/l.
Table 6. Association between maternal selenium blood concentration in mid-pregnancy and risk of preterm delivery*
(Hazard ratios (HR) and 95 % confidence intervals)

* Maternal blood Se concentrations (μg/l) and HR for preterm delivery (22+0–36+6 weeks) in all subjects with Se blood measurements (n 2638) and in spontaneous deliveries only (n 2177) analysed with Cox regression. Number of preterm cases: 80 of all 2638 subjects and 55 of the 2177 spontaneous deliveries. Blood Se concentrations were measured in whole blood collected in gestational week 17–18.
† Adjusted for maternal age, parity, smoking habits, alcohol consumption during pregnancy, maternal education and BMI.
‡ HR per standard deviation (23·4 μg/l) of blood Se concentrations.
§ Measured in μg/l.
‖ Iatrogenic deliveries have been censored in the regression model.
Ethical approvals
The establishment of MoBa and initial data collection was based on a license from the Norwegian Data protection agency and approval from The Regional Committee for Medical Research Ethics. The MoBa cohort is currently regulated by the Norwegian Health Registry Act. The present study was approved by The Regional Committee for Medical Research Ethics (2015/2425/Rek sør-øst A).
Results
Selenium intake and selenium status in the study population
The median intake of Se from the diet was 53 (interquartile range (IQR) 44–62) µg/d for all women (n 72 025, Table 1). Fig. 2 shows how much the different food groups contributed to dietary Se intake. Seafood, bread, meat and pasta accounted for 24, 19, 19 and 10 % of the Se in the diet, respectively. Other important sources included dairy products, fruits and vegetables, eggs and cheese (Fig. 2). In Norway, no food items are fortified with Se.

Fig. 2. Contribution (%) to dietary selenium intake by different food sources. , Seafood;
, bread;
, meat, all types;
, pasta;
, dairy products;
, fruit and vegetables;
, eggs;
, cheese;
, rice, millet, couscous;
, waffles, pancakes;
, pizza, tacos;
, cereals;
, snacks and candy;
, biscuits and cookies;
, nuts;
, rice porridge.
Median Se intake from supplements was 50 (IQR 30–75) µg/d for the 23 409 (33 %) Se supplement users (Table 1). Most of these women (n 20 812) consumed Se supplements containing only inorganic selenate or selenite, which provided a median supplemental intake of 50 (IQR 35–75) µg/d (Table 1). A smaller number (n 3283) took supplements containing organic selenomethionine or selenised yeast, which provided a median supplemental intake of 30 (IQR 21–43) µg/d (Table 1). The RDI of Se for pregnant women is 60 µg/d. About a third of the cohort (n 21 073) met the recommended dietary intake from their diet alone, while half (n 36 135) did so through the use of Se-containing supplements. The women with a dietary Se intake above RDI were those more likely to consume Se supplements compared with women whose Se intake is below RDI (33 v. 32 %, P = 0·005).
Median whole-blood Se concentration (Se status) was 102 (IQR 89–117) µg/l. This parameter correlated weakly with the estimated dietary Se intake (Spearman’s ρ = 0·135, P < 0·001); while it was not correlated with estimated Se intake from supplements (Table 1). The distribution of Se intake and whole-blood Se concentration is shown in Fig. 3.

Fig. 3. Distribution of dietary selenium intake and selenium intake from supplements (organic and inorganic forms combined). Histograms showing the intake of selenium from food (a) and from supplements (b). Only supplement users (n 23 409) are included in (b). RDI, recommended daily intake. For pregnant women, the RDI for selenium is 60 µg/d.
Maternal characteristics in relation to selenium intake and selenium status
Dietary Se intake differed by most of the maternal characteristics studied (Table 2). Dietary Se intake was positively associated with age, education, household income, parity, gestational length, alcohol consumption during pregnancy, iodine intake, protein intake, n-3 intake, fibre intake and total energy intake, while it was negatively associated with smoking and BMI (Table 2).
Se status was positively associated with age, education, alcohol consumption, n-3 intake and fibre intake and negatively associated with maternal BMI, parity and smoking (Table 2).
Maternal selenium intake and gestational length
Dietary Se intake was positively associated with the length of gestation; β per sd = 0·25, adjusted model, that is, an increase in intake of Se by 1 sd, 14·6 μg/d, increased gestation by 6 h (Table 3). Regression analysis for spontaneous deliveries (n 57 098) only showed a positive association between maternal dietary Se intake and length of gestation (Table 3).
Among all 72 025 deliveries, 3618 (5 %) women delivered preterm, 952 (1 %) women delivered early preterm, 2666 (4 %) late preterm, 11 660 (16 %) early term and 56 747 (79 %) delivered late term. Among the 57 098 spontaneous deliveries, 2100 (4 %) were preterm, 482 (1 %) early preterm, 1618 (3 %) late preterm, 7873 (14 %) early term and 47 125 (82 %) late term.
Higher maternal dietary Se intake was associated with decreased risk of PTD in all subjects (HR per SD: 0·92, 95 % CI, 0·87, 0·98) as well as cases of spontaneous deliveries only (HR per sd: 0·88, 95 % CI, 0·81, 0·96) (Table 4).
No associations were found between intake of inorganic (selenite/selenate) or organic Se supplements (selenomethionine/selenised yeast) and gestational length or PTD (Tables 3 and 4).
Analyses of intake of dietary Se and Se-containing supplements in relation to the risk of early preterm, late preterm and early term deliveries showed one significant association, that is, Se intake from food was associated with a decreased risk of late PTD (adjusted HR per sd: 0·90, 95 % CI, 0·84, 0·97) (online Supplementary Table S1).
Threshold analysis
Possible threshold effects of dietary Se intake were studied by entering Se categorised into deciles into the Cox regression models (online Supplementary Table S2). Women in four of the seven lowest intake categories had an increased risk of PTD compared with the reference category. No significant association was found for higher intake categories compared with the reference category (online Supplementary Table S2).
Maternal selenium status, gestational length and preterm delivery
Maternal Se status at gestational week 17 was associated neither with gestational length nor with PTD (Tables 5 and 6). The subgroup with available whole-blood Se measurements was relatively small (n 2638) and included only eighty women who delivered preterm, fifty-five of these with spontaneous deliveries, limiting the statistical power.
Discussion
Higher maternal dietary Se intake in the first half of pregnancy was associated with increased gestational length and reduced risk of PTD. The results were more pronounced when the analyses were confined to women with spontaneous deliveries only. Threshold analyses suggest that the association is driven by Se intake below the RDI and that Se intake above the RDI does not affect PTD risk. While a 6-h increase in gestational length might not seem clinically relevant, this can be expected due to the intake of a single nutrient at the population level. The corresponding findings for a decreased PTD risk underline the clinical relevance of the results. No associations were found between the intake of Se-containing supplements or Se status and gestational length or risk of PTD.
Most of the dietary Se are in proteins. The bioavailability of dietary Se depends on the digestibility of the proteins. The protein-bound forms (selenocysteine and selenomethionine) need to be converted to single amino acids and short polypeptides that can be absorbed( Reference Combs, Combs, Combs and Combs37 ). Se in animal products tends to be better utilised compared with Se from plant tissues( Reference Combs, Combs, Combs and Combs37 , Reference Cantor, Moorhead and Musser38 ) due to the better digestibility. Fish can be an exception( Reference Cantor, Scott and Noguchi39 ) if it is high in heavy metals that bind Se in poorly digestible adducts( Reference Shamberger40 ). Dietary supplements typically contain Se in the form of Se -enriched brewers’ yeast or sodium selenite or selenate. Se in yeast needs to be digested to free Se from the yeast proteins; while the inorganic forms (sodium selenite or selenate) can be absorbed directly. Hence, bioavailability probably does not explain the different results for dietary Se being associated with gestational length, while there was no association with Se intake from supplements. Rather, the results suggest that most of the women in the cohort have dietary Se intake near or above the level associated with optimal selenoprotein expression, especially the third of women who also consumed Se-containing supplements who are more likely to have Se intake from food more than the RDI. Thus, intake of Se-containing supplements would not further improve selenoprotein expression affecting metabolic pathways related to birth outcome, for example, inflammation or redox status.
Se in whole blood circulates either bound to plasma-selenoproteins glutathione peroxidase 3 and selenprotein 1 or in the form of glutathione peroxidase 1 in the erythrocytes and lymphocytes. Also other proteins that contain Se non-specifically incorporated in lieu of methionine are found in both compartments. The relative expression level of these three selenoproteins is thought to reflect the expression of other selenoproteins in the body. The expression of selenoproteins is dependent not only on dietary Se intake but also on genetic variation in genes involved in the Se metabolism such as dimethylglycine dehydrogenase( Reference Evans, Zhu and Dy41 ), selenoprotein P( Reference Meplan, Crosley and Nicol42 ), the glutathione peroxidases, cytosolic glutathione peroxidase and phospholipid glutathione peroxidase( Reference Meplan, Crosley and Nicol43 ). Based on the findings from our recent genome-wide association study, where we reported genome-wide significance for one of the genes involved in the Se metabolism, the EEFSEC gene, we hypothesised that Se intake and/or Se status during pregnancy would be correlated with gestational length and PTD. The epidemiological findings in the present study, that dietary Se intake in the first half of pregnancy was associated with increased gestational length and reduced risk of PTD, further support this hypothesis. As a next step, we plan to perform gene–environment interaction analyses to gain further knowledge in how Se influences the length of gestation and the risk of PTD and to examine whether EEFSEC polymorphisms interfere with the association between dietary Se intake and whole blood Se concentrations.
Only a few other studies evaluated Se intake in relation to gestational length and/or PTD. One small nested case–control study in New Jersey, USA, including 107 women who delivered preterm and 126 control women, used a 24-h recall dietary assessment to estimate Se intake at entry to prenatal care. The results showed that women who delivered preterm had a higher Se intake in the first trimester compared with controls (115 (9) v. 93 (5) µg/d, P < 0·03)( Reference Bogden, Kemp and Chen14 ), hence opposite results to what was found in the present study. However, the New Jersey study compared only 107 cases with 126 controls, while the present study compared 3618 cases with 68 407 controls. Further, the FFQ used in the MoBa cohort has been extensively validated( Reference Brantsaeter, Haugen and Thomassen18 , Reference Brantsaeter, Haugen and Alexander19 ). A larger multicentre study in the USA, including 5738 deliveries (471 PTD cases) from the National Birth Defects Prevention Study found no association between Se intake and PTD. Se intake during the year before pregnancy was estimated retrospectively by telephone interview 6–24 months after delivery using a short FFQ of 58 food items, impairing the validity of the results( Reference Carmichael, Yang and Shaw13 ). For the MoBa cohort, dietary data were collected prospectively with an extensively validated instrument in a more than 10 times as large study population. The USA study reported a higher intake compared with that reported in Norway in the MoBa cohort( Reference Carmichael, Yang and Shaw13 ).
For 2638 of the 75 025 women included in the present study, Se status measured in gestational weeks 17–18 was available. No associations were found between Se status and either of the outcomes, gestational length and PTD. We are not aware of other studies that evaluated Se in whole blood in the first half of pregnancy in relation to gestational length and PTD. However, in accordance with the present findings, Bogden et al.( Reference Bogden, Kemp and Chen14 ) observed no differences in plasma Se concentration at gestational week 15 for women who delivered preterm (n 107) compared with those who delivered at term (n 126) (1·37 (0·02) v. 1·34 (0·02) µmol/l). On the other hand, Rayman et al.( Reference Rayman, Wijnen and Vader15 ) found that women in the Netherlands who delivered preterm (n 60) had lower serum Se concentration at gestational week 12 than those who delivered at term (0·96 (0·14) v. 1·02 (0·13) μmol/l, P = 0·001). We have reported Se concentration in whole blood in µg while Rayman et al. and Bogden et al. reported the Se concentrations in serum in µmol. The median Se of 102 µg/l in whole blood found in our study population corresponds to 1·3 µmolar/l, which is comparable with the concentration measured in serum and plasma in the other studies.
Se from diet and supplements containing organic Se (selenomethionine or selenised yeast) was weakly correlated with Se concentration in blood, while no association was found for inorganic Se. Selenomethionine, the dominant form in foods, is incorporated specifically into selenoproteins as well as non-specifically into other proteins, for example, albumin. It is likely that women consuming less than 60 µg of the RDI had suboptimal expression of selenoenzymes, and they would be expected to show increased selenoprotein expression in response to all absorbable forms of Se.
An interesting aspect of our findings is that blood Se concentrations in Norwegians have dropped quite dramatically in the last 30 years, from being among the highest in Europe, on average approximately 150 µg/l( Reference Meltzer, Norheim and Bibow44 ) whole blood (corresponding to 120 µg/l serum/plasma) to approximately 100 µg/l in the present study. This has brought Norwegians down to similar blood Se concentration as found among Swedes and Danes. This change may relate to decreasing consumption of trans-Atlantic flour from Se-rich areas in Canada and the USA, in favour of increased use of North-European grains, which are typically of lower Se content. The finding in the present study that supplementary Se had little influence on the blood concentration might be due to the fact that the third of women who chose to consume Se-containing supplements were those who were more likely to meet the RDI by their dietary Se intake.
Strengths and limitations
This is by far the largest study investigating the association between Se intake and gestational length and PTD. The prospective design and the detailed information about maternal diet, demography, socioeconomic factors and pregnancy outcomes are further strengths. The study is population based, including women from all over Norway, representing women living in urban and rural areas, coastland and inland regions, different socioeconomic groups and women with diverse dietary habits. Due to the large sample size, even subgroups of PTD could be studied.
Observational studies cannot establish causality as there is always a risk of residual confounding. However, the comprehensive data set allowed us to account for a large number of possible confounders, including maternal age and education, parity, smoking and energy intake.
The dietary intake was estimated before delivery using an FFQ specifically developed and validated for use in this cohort( Reference Meltzer, Brantsaeter and Ydersbond17 , Reference Brantsaeter, Haugen and Alexander19 ). Se intake estimated using this instrument was significantly correlated with Se intake estimated using a 4-d food diary in the validation study (r 0·28 for Se in food)( Reference Brantsaeter, Haugen and Alexander19 ). However, the use of an FFQ has limitations and is more suitable for ranking participants according to high and low intake than for precise intake calculations. Since the Se content varies according to the Se concentration of the soil where crops are grown or the animals graze, some uncertainty is associated with estimating Se intake from food composition databases. When testing whether the Se content in blood varied depending on the year of inclusion into MoBa, significant differences were found, with concentrations ranging from a median of 87 to 114 µg/l between years 2003 and 2009 (results not shown). Women in the present study reported the intake of food and dietary supplements. Food habits are often stable over time, while intake of supplements fluctuate, especially during such a sensitive period as pregnancy, suggesting that the long-term intake of Se, and hence storage of Se in the body, is better captured in the questions about food than about supplement use.
Participants in MoBa were recruited between 10 and 20 years ago and dietary trends change over time. This may limit the translation of the findings. However, Se is found in many different food items and most of them are staple foods such as wheat, meat and fish. We have little reason to believe that major changes were observed in the intake pattern of Se-rich food items during the past decade, though the dishes they are part of may have changed.
The generalisability of the results from the Se measurements in whole blood may be limited due to selection bias. Since one inclusion criterion for participation in the Norwegian Environmental Biobank Study was to have answered all of the first six MoBa questionnaires, the subgroup of women who have Se measurements do not represent the whole MoBa population( Reference Caspersen, Thomsen and Haug27 ). Women in the subsample included a lower proportion with missing information on baseline characteristics and a higher proportion of non-smokers and highly educated compared with those in the whole MoBa( Reference Caspersen, Thomsen and Haug27 ).
Conclusions
Higher Se intake from food but not from supplements was associated with a small increase in gestational length and decreased risk of PTD in 72 075 women participating in MoBa, strengthening the results from the first genome-wide association study on gestational length and PTD( Reference Zhang, Feenstra and Bacelis4 ). The reason for the different findings for Se in different sources, that is, from supplements and from food, may be due to the fact that about half of the women had a dietary Se intake more than the RDI, which is thought to guarantee an optimal selenoenzyme expression. Further studies, preferably in the form of randomised controlled trials and gene–environment interaction analyses, are needed before considering to change the present dietary guidelines regarding Se intake by pregnant women.
Acknowledgements
The Norwegian Mother, Father and Child Cohort Study is supported by the Norwegian Ministry of Health and Care Services and the Ministry of Education and Research. We are grateful to all the participating families in Norway, who take part in this on-going cohort study.
The Norwegian Mother, Father and Child Cohort Study is supported by the Norwegian Ministry of Health and Care Services and the Ministry of Education and Research, NIH/NINDS (grant no. 1 UO1 NS 047537-01 and grant no. 2 UO1 NS 047537-06A1). Payment for access to data and article-processing charges for this publication was covered by grants from the Wilhelm and Martina Lundgren Foundation (grant no. 2017-1788 to M. B.) and The Royal Society of Arts and Sciences in Gothenburg (grant no. 2017-054 to M. B.). The Bill & Melinda Gates Foundation provided grant no. OPP1175128 to L. J. M. Agreement concerning research and education of doctors (ALFGBG-717501), Sahlgrenska University Hospital, Sahlgrenska Academy, Gothenburg, Sweden, to B. J. March of Dimes (no. 21-FY16-121) to B. J. The Health & Medical Care Committee of the Regional Executive Board, Region Västra Götaland, Sweden (VGFOUREG-658361) to B. J.
All co-authors have contributed significantly to the planning of the analyses and interpretation of the data and have provided essential intellectual input. M. B., S. N. and V. S. did the statistical analyses. M. B. wrote the first draft of the manuscript. All authors approved the final version of the manuscript. The principal investigator, V. S., had full access to the data and had final responsibility for the decision to submit for publication.
The authors declare no conflicts of interest regarding the content of this paper.
Supplementary material
For supplementary material/s referred to in this article, please visit https://doi.org/10.1017/S0007114519002113