Higher or lower fetal growth has been implicated in a number of childhood and adult-onset chronic diseases and conditions including type 2 diabetes, hypertension, obesity and CVD(Reference Whincup, Kaye and Owen1–Reference Boney, Verma and Tucker6). Epigenetic processes, particularly DNA methylation, regulate fetal programming and development by influencing gene expression(Reference Smith and Meissner7). Evidence of both animal models and human study has shown that offspring DNA methylation can be modified by maternal intake of methyl nutrients (e.g. folate, choline, betaine and methionine) involved in one-carbon metabolism(Reference Sinclair, Allegrucci and Singh8–Reference Pauwels, Ghosh and Duca11). Previous research has extensively studied the potential role of maternal folate intake rather than other methyl donors in influencing fetal growth, and meta-analyses have demonstrated that higher maternal folate intake is associated with an increase in birth weight or with a lower risk of small-for-gestational-age (SGA)(Reference Hodgetts, Morris and Francis12–Reference Zhang, Wang and Xin14).
Similar to folate, choline and its related metabolites also play an important role in one-carbon metabolism. Choline can be irreversibly oxidised to betaine, which further provides a methyl group to homocysteine to form methionine and eventually the universal methyl donor S-adenosylmethionine(Reference Ueland15). This pathway is paralleled to the 5-methyltetrahydrofolate-dependent route in re-methylation of homocysteine. Choline is also necessary for maintaining the integrity of cell membrane and serves as the precursor for the neurotransmitter acetylcholine(Reference Zeisel and da Costa16). Another role of betaine is that it functions as an intracellular osmolyte to protect cells under stress(Reference Ueland, Holm and Hustad17). Dimethylglycine (DMG) is formed after betaine providing methyl group to homocysteine. Methionine, also an essential amino acid, is particularly important during fetal development because of high demands for protein synthesis(Reference Rees, Wilson and Maloney18). To date, only three epidemiologic studies have explored the association of maternal circulating choline and betaine with birth weight, and the results have been inconsistent(Reference van Lee, Tint and Aris19–Reference Hogeveen, den Heijer and Semmekrot21). While a large mother–offspring cohort reported an inverse association between maternal plasma betaine and birth characteristics included, that is, birth weight or birth length or abdominal fat mass(Reference van Lee, Tint and Aris19), other studies reported null association between either biomarkers and birth weight(Reference Ivorra, Garcia-Vicent and Chaves20, Reference Hogeveen, den Heijer and Semmekrot21). Besides, we are aware of only one epidemiological study that has explored amniotic fluid methionine status and offspring birth weight(Reference Monsen, Schneede and Ueland22); this study showed a positive association. Various factors, such as sample size, differences in gestation age underwent a venipuncture and confounders controlled, could contribute to these inconsistent results.
In this study, we aimed to investigate the association of maternal plasma choline and its related nutrients with birth weight, birth length, SGA and large-for-gestational-age (LGA) by utilising a hospital-based cohort study. Stratified analyses by infant sex were performed because it has been found that restriction of maternal methyl donors intake was associated with offspring’s methylation changes of some genes in a sex-specific manner(Reference Sinclair, Allegrucci and Singh8). Homocysteine is closely interrelated with methyl donors in one-carbon metabolism, and a causal role for maternal homocysteine in offspring birth weight was observed(Reference Yajnik, Chandak and Joglekar23). We thus also evaluated whether the association between assayed biomarkers and birth weight was modified by maternal homocysteine status.
Methods
Subjects
A hospital-based cohort study was conducted in Peking University Third Hospital in Beijing, China, between March 2017 and January 2018. Singleton pregnant women aged 18–45 years, conceived naturally and planned to delivery at the participating hospital. Ineligibility was due to an intention to deliver in other hospitals or conceived through assisted reproduction techniques. Eligible women were recruited from the antenatal clinics when they first presented at this hospital. Among 260 eligible women, 176 were willing to participate in our study and agreed to donate a blood sample during the routine blood sampling used for clinical care. Further, sixty-one participants were excluded based on the characteristics of offspring: birth weight lower than 1500 g (n 17), early preterm (gestational age ≤34 weeks) (n 25), had congenital disease (n 9), malformation (n 7) or chromosomal abnormality (n 3). Finally, a total of 115 mother–infant pairs were included in the present study (Fig. 1). The study was approved by the Peking University Third Hospital Medical Ethics Committee (IRB00006761-2016145), and informed consent was obtained from the participants.
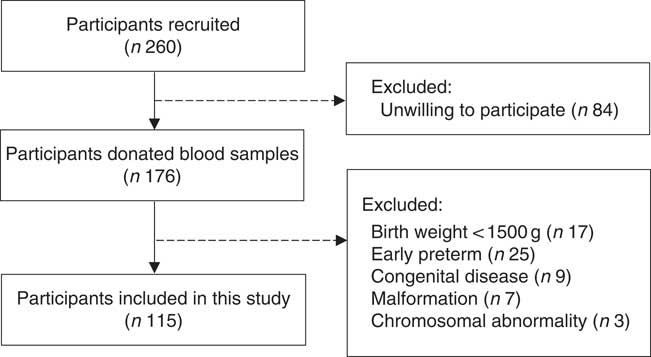
Fig. 1 Flow diagram of participants included in the present study.
Data on main outcomes and covariates
Information on birth weight and birth length was obtained from the hospital delivery records. Neonates, whose birth weight was <10th percentile or ≥90th percentile of growth curves based on gestational age and sex(Reference Mikolajczyk, Zhang and Betran24), were categorised as SGA or LGA, respectively. Gestational age at birth was calculated using the last menstrual period and was subsequently confirmed or revised by early ultrasound. Gestational age based on ultrasound findings was used when there was a discrepancy larger than 10 d. Low birth weight (n 5) and high birth weight (n 8) were not used as interested outcomes due to the small numbers.
Data on maternal characteristics like parity, the age of mother at delivery, medical history, marital status and ethnic group were abstracted from medical records. Weight and height of participants were measured by nurses after admission. Drinking, smoking and weight gain during pregnancy were self-reported by participants at the time of enrolment. Maternal folic acid intake during pregnancy was recalled by mothers after delivery. Pre-pregnancy weight was calculated as the difference between measured weight and self-reported weight gain. Pre-pregnancy BMI was determined by dividing calculated weight (kg) by height squared (m2). Women were categorised into three groups according to smoking: non-smoker, a former smoker and current smoker. Alcohol drinking during pregnancy was also asked (yes or no). Data were separately abstracted by two trained staff, and the consistency was verified.
Sample collection and processing
Early morning fasting blood samples were collected in EDTA-treated tubes, immediately stored in a 4°C refrigerator (storage time was within a maximum of 20 h) and were subsequently transported on ice to the laboratory (within 0·5 h) for processing. Blood drawing was concurrent with the routine blood sampling. Samples were centrifuged at 3000 rpm for 10 min at 4°C, and the aliquoted supernatants were stored at –80°C refrigerator until needed for the analysis.
Measurement of plasma biomarkers
Concentrations of choline, betaine, DMG, methionine and homocysteine were analysed by HPLC (Shimadzu)-tandem MS (API 4000; MDS Sciex) based on the method of Midttun et al. (Reference Midttun, Kvalheim and Ueland25) with modifications. Briefly, after a 30 µl plasma mixed with 5 µl internal standard solution followed by a vortex, 150 µl of methanol was added to precipitate proteins. The mixture was further vortexed for 30 s and centrifuged at 13 000 g for 20 min at 4°C. A 150 µl supernatant was transferred to an Eppendorf tube and 2 h of freeze-drying was done to remove methanol. Dried samples were then re-dissolved in 150 µl of 50% acetonitrile, and 10 µl of supernatant was removed and injected into the HPLC-MS/MS system. Choline-d9, DMG-d6 and methionine-d3 were used as internal standards. Cysteamine hydrochloride was used as the internal standard for homocysteine. Biomarkers of interest were separated by an XBridge BEH Phenyl column (150×4·6 mm, 3·5 µm) from Waters. Mobile phases consist of phase A (0·1 % formic acid in water) and phase B (0·1 % formic acid in acetonitrile). We used a column flow rate of 0·5 ml/min, a sample injection volume of 5 µl and the column temperature of 40°C for the analysis. De-clustering potentials and collision energies were optimised for each biomarker and internal standard. Duplicate control samples were interspersed in each analytical batch to assess laboratory precision. The between-day CV are as follows: 2·4 % for choline, 6·7 % for betaine, 1·6 % for methionine, 3·4 % for DMG and 1·3 % for homocysteine. All assays were conducted by laboratory technicians, and all of them were blinded to the clinical information of participants.
Statistical analysis
Biomarker concentrations are not distributed normally and therefore are reported as medians (25th–75th percentiles). Differences in concentrations across groups regarding characteristics about mother and child were examined by the Mann–Whitney U test or Kruskal–Wallis test. For each of the biomarkers, we log transformed and then created a standard deviation score before further analyses. Multiple linear regression models were constructed to assess the effects of maternal biomarkers on continuous outcomes (e.g. birth weight and birth length). The effect size is interpreted as changes in outcomes per 1sd change in the log-transformed exposure. The association between dichotomous outcomes (SGA and LGA) and maternal blood biomarker was estimated as OR using logistic regression models. We used restricted cubic splines to examine whether non-linear associations between biomarkers with birth weight, birth length, SGA and LGA exist (rms-package in R statistical software), and the result showed that all P non-linearity>0·1. In the present study, all participants were married, were non-smokers and were not drinking during pregnancy. Besides, 96·2 % of our participants were Han Chinese. We, thus, did not adjust marriage status, smoking, drinking and ethnicity in multivariate models. Effect values were obtained from models with adjustment for gestational age, gestational age at venipuncture (in 2-week intervals) and fetal sex (model 1), from models additionally controlled for pre-pregnancy BMI (<18·5, 18·5–24·9, ≥25 kg/m2), maternal age (<35, ≥35 years), parity, gestational diabetes (yes/no), plasma homocysteine status (<90 percentile, ≥90 percentile) (model 2), as well as from model 3 adjusting for confounders in model 2, plus mutually adjusted four plasma biomarkers. Models for SGA and LGA were not adjusted for newborn’s sex and gestational age.
Stratified analyses by infant sex or maternal plasma homocysteine status (<75 percentile, ≥75 percentile) were performed. Covariates adjusted in stratified analyses were selected due to the limited sample size. Covariates that were considered clinically relevant (plasma biomarkers) or with a P value <0·2 on univariate analysis with response variable were finally included in a multivariate model. To assess the robustness of our results, we performed sensitivity analyses after excluding mothers having gestational diabetes. Comparisons on several key variables (e.g. maternal age, gestational age and birth weight) between study participants and randomly selected 400 women visited this hospital during the study period were performed to check for the representative. In this study, significance was defined as P<0·05, and all statistical tests were two tailed. Statistical analyses were conducted by SPSS software (version 24.0) and R statistical software (version 3.4.0).
Results
As shown in Table 1, the majority of the included mothers were aged <35 years (86·1 %), were nulliparous (82·6 %) and had pre-pregnancy BMI range from 18·5 to 24·9 kg/m2 (78·3 %). Notably, 20 % of women suffered gestational diabetes. The newborns, of whom sixty-two were boys (53·9 %), 88·7 % had a birth weight within a normal range (2500–3999 g), and 95·7 % had a gestational age ≥37 weeks. All plasma biomarker concentrations did not differ significantly across groups for each characteristics. Mean for maternal age and pre-pregnancy BMI was 31·5 (sd 3·1) years and 21·7 (sd 2·7) kg/m2, respectively.
Table 1 Maternal biomarker concentrations (µmol/l) according to maternal and infant characteristicsFootnote * (Numbers and percentages; medians and 25th, 75th percentiles)

* P values are obtained from the Mann–Whitney U test or Kruskal–Wallis test.
The association of maternal plasma choline, betaine and DMG and methionine with birth weight and birth length is shown in Table 2. Higher maternal plasma betaine concentrations were associated with lower birth weight in all three adjusted models (model 3: β=–130·3 g per 1sd increment; 95 % CI –244·8, –15·9 g). A significant inverse association was also seen for choline with birth weight in model 1, but this association weakened sharply and became not statistically significant after inclusion of plasma betaine, DMG and methionine in model 3. None of the biomarkers was associated with birth length in our study.
Table 2 Associations of maternal biomarker concentrations (µmol/l) with birth weight and birth lengthFootnote * (β-Coefficients and 95 % confidence intervals)

* Model 1: adjusted for gestational age, gestational age at venipuncture (in 2-week intervals) and fetal sex. Model 2: adjusted for confounders in model 1 in addition to pre-pregnancy BMI (<18·5, 18·5–24·9, ≥25 kg/m2), maternal age (<35, ≥35 years), parity, gestational diabetes, plasma homocysteine status (<90 percentile, ≥90 percentile). Model 3: model 2 and mutually adjusted for plasma choline, betaine, dimethylglycine and methionine.
Table 3 shows the association of maternal plasma biomarkers with SGA and LGA risk. A strong inverse relationship between maternal methionine status and LGA risk was observed in either crude or adjusted models. The adjusted OR was 0·44 (95 % CI 0·21, 0·89) for every 1sd increment of methionine concentrations. Plasma choline and betaine was also inversely associated with LGA risk and crude OR was 0·51 (95 % CI 0·27, 0·96) and 0·48 (95 % CI 0·26, 0·88), respectively, but these associations did not survived after further controlling for covariates. Of note, both maternal betaine and methionine status were not associated with the increased risk of SGA.
Table 3 Risks of small-for-gestational-age (SGA)/large-for-gestational-age (LGA) according to each maternal biomarker concentrations (µmol/l)Footnote * (Odds ratios and 95 % confidence intervals)

* Model 1: crude models. Model 2: adjusted for gestational age at venipuncture, gestational diabetes, maternal age, pre-pregnancy BMI (<18·5, 18·5–24·9, ≥25 kg/m2), parity, plasma homocysteine status (<90 percentile, ≥90 percentile). Model 3: covariates in model 2 and mutually adjusted for plasma choline, betaine, dimethylglycine and methionine.
Stratified analysis based on infant sex showed that reduction in birth weight related to maternal betaine status only limited to male infants (β: –156·0 g per 1sd increment, 95 % CI –305·1, –6·9; P=0·041) (Table 4). Besides, the inverse association between maternal betaine status with birth weight were mainly significant among women with higher homocysteine status (≥5·1 µmol/l) (β: –206·3 g per 1sd increment, 95 % CI –402·5, –10·0; P=0·040) (Table 5). The participants in the present study did not significantly differ from randomly selected 400 women concerning key variables as follows: maternal age (P=0·966), gestational age (P=0·164), ethnicity (P=0·255), gestational diabetes (P=0·127) and birth weight (P=0·455). Sensitivity analyses for main results yielded similar results, but the association was slightly weaker (data not shown).
Table 4 Association between maternal biomarker concentrations (µmol/l) with birth weight according to infant sexFootnote * (β-Coefficients and 95 % confidence intervals)

* Adjusted for gestational age, gestational diabetes and mutually adjusted for plasma choline, betaine, dimethylglycine and methionine.
Table 5 Association between maternal biomarker concentrations (µmol/l) with birth weight according to maternal plasma homocysteine statusFootnote * (β-Coefficients and 95 % confidence intervals)

* Stratification is based on 75th percentile of maternal homocysteine concentrations in this study.
† Adjusted for gestational age, infant sex, gestational age at venipuncture (in 2-week intervals), gestational diabetes, and mutually adjusted for plasma choline, betaine, dimethylglycine and methionine.
Discussion
To our knowledge, this is the first study to explore the role of maternal choline and its related metabolites in one-carbon metabolism in infant growth in a Chinese population. This study shows that high maternal plasma betaine concentrations were associated with the lower birth weight of infants. This association seemed to be stronger in male infants, or those had higher maternal homocysteine status. Higher maternal plasma methionine was associated with lower risk of LGA.
Our finding that maternal plasma betaine concentrations were negatively associated with birth weight is strengthened by results from Growing Up in Singapore Towards Healthy Outcomes (GUSTO) cohort study( Reference van Lee, Tint and Aris19 ), the largest mother–offspring cohort to date explored such an association. The GUSTO study also showed that higher maternal plasma betaine was associated with less abdominal fat mass, shorter birth length and increased risk of SGA( Reference van Lee, Tint and Aris19 ). However, another study conducted in the Netherlands found a null association, while an inverse association was observed for umbilical cords betaine status with birth weight( Reference Hogeveen, den Heijer and Semmekrot21 ). Both the GUSTO study and our study collected fasting blood samples. The difference in study population might partly explain these inconsistent findings because the dietary pattern has been found to have a profound effect on methyl-donor biomarker status( Reference Dominguez-Salas, Moore and Cole26 ). The significant positive association between maternal betaine status and SGA risk, showed in GUSTO, was not found in our study; this can be explained by the limited power of the analysis resulted from the small number of SGA infants. Besides, a recent study showed that maternal betaine supplementation prevented fetal and placental overgrowth in high-fat-fed mice( Reference Joselit, Nanobashvili and Jack-Roberts27 ). Maternal plasma betaine have been found to remain constant from twenty-two gestational weeks to the rest of pregnancy( Reference Velzing-Aarts, Holm and Fokkema28 , Reference Fernandez-Roig, Cavalle-Busquets and Fernandez-Ballart29 ), a stage covering the period of our blood sample collection. A strong correlation between maternal betaine status and cord blood betaine status was observed( Reference Visentin, Masih and Plumptre30 ).
Evidence from animal and human adult betaine supplementation trials as well as the GUSTO study suggested that the possible mechanisms of the observed decrease in birth weight related to betaine may involve adiposity reductions in infants(Reference van Lee, Tint and Aris19, Reference Eklund, Bauer and Wamatu31, Reference Cholewa, Guimaraes-Ferreira and Zanchi32). Betaine supplementation has been shown to promote muscular fatty acid uptake and oxidation via up-regulation of related genes in pigs(Reference Li, Wang and Wang33). Increased betaine-dependent remethylation of homocysteine may reduce the availability of acetyl-coenzyme A, a substrate required for fatty acid synthesis and fat deposition(Reference Lawrence, Schinckel and Adeola34). Betaine also promotes the biosynthesis of carnitine by providing a methyl group. Carnitine is necessary for the oxidation of long-chain fatty acids and has been associated with reduced carcass fat content in pigs(31).
In the stratified analyses, we found that significant association between betaine and birth weight was mainly among male infants. In animal models, epigenetic changes and phenotypic differences in the offspring caused by restriction of peri-conceptional methyl donors intake were most pronounced in males(Reference Sinclair, Allegrucci and Singh8). Previous studies conducted among adults also demonstrated that favourable body composition (e.g. lower weight and lower body fat) associated with higher serum betaine was mainly seen for male participants(Reference Gao, Randell and Zhou35, Reference Chen, Liu and Liu36). This sexual dimorphism might involve the differential gene methylation alterations in response to betaine between males and females. It was observed that higher maternal intake of betaine is associated with lower cord blood methylation in males only(Reference Boeke, Baccarelli and Kleinman10). A randomised controlled trial of peri-conceptional nutrient supplementation, which used supplements containing nutrients involved in one-carbon metabolism such as folic acid and vitamin B12, showed little overlap between males and females in the gene that underwent methylation alterations associated with supplementation(Reference Khulan, Cooper and Skinner37).
We noted a substantial reduction in birth weight related to the higher maternal betaine and homocysteine concentrations. Maternal betaine was inversely associated with homocysteine only when folate status was low(Reference Fernandez-Roig, Cavalle-Busquets and Fernandez-Ballart29, Reference Holm, Hustad and Ueland38). However, a small but significant positive association between betaine and homocysteine was observed in our study (Spearman correlation coefficient was 0·20, P=0·03). We speculated that folate status, B vitamins and genetic variants might explain the inconsistent correlation. We assumed that our participants were in a relatively folate-replete condition because 98, 89, 60, 24 % of our participants reported that they took folic acid before pregnancy, during the first, the second and third trimester of pregnancy, respectively. As for the adherence, 84 % of mothers reported that they took folic acid in ≥8 out of 10 d. In addition, some other important predictors of homocysteine status such as methylenetetrahydrofolate reductase 677 C→T polymorphism and B vitamins (B2, B6 and B12), all of which were not determined in our study, modulate the homocysteine–betaine relationship(Reference Holm, Hustad and Ueland38). The effect of betaine and homocysteine on birth weight cannot be differentiated because of their correlation, and our observed birth weight reduction might reflect the effect of both biomarkers. The inverse association between homocysteine and birth weight has been well illustrated in previous studies(Reference Yajnik, Chandak and Joglekar23, Reference Hogeveen, Blom and den Heijer39), and the mechanisms involve the impaired placental perfusion and function, resulting from the damaging effect on endothelial cell(Reference Gaiday, Tussupkaliyev and Bermagambetova40). Further studies are needed to clarify the biological mechanisms of these results. Given the limited sample size, results based on stratified analyses might be a chance finding.
The demand for choline is high during pregnancy due to the accelerated one-carbon metabolism and the formation of new membranes during cellular division(Reference Caudill41). However, previous studies(Reference Ivorra, Garcia-Vicent and Chaves20, Reference Hogeveen, den Heijer and Semmekrot21) together with ours failed to find association between maternal choline and birth weight. It has been shown that plasma choline increased progressively from fifteen gestational weeks throughout the rest of pregnancy(Reference Fernandez-Roig, Cavalle-Busquets and Fernandez-Ballart29), mainly due to the mobilisation of maternal hepatic choline stores and endogenous synthesis enhanced by oestrogen(Reference Yan, Jiang and West42). Associations between choline status with outcomes might be masked because we collected blood samples in a pregnancy stage during which plasma choline is increasing. Furthermore, it should also be noted that maternal plasma choline at delivery was not associated with cord plasma choline(Reference Visentin, Masih and Plumptre30).
Although maternal methionine was unrelated to birth weight, a 56 % decreased risk of LGA per 1sd increment of maternal plasma methionine was observed in our study. LGA is related to prolonged delivery, excessive maternal haemorrhage and caesarean sections(Reference Ng, Olog and Spinks43), and an increased risk of obesity, diabetes and CVD later in life(Reference Taal, Vd Heijden and Steegers5, Reference Boney, Verma and Tucker6). Recently a study in sows has demonstrated that methionine supplementation during late gestation lowered the TAG levels(Reference Bin, Azad and Liu44). Furthermore, the third-trimester maternal TAG status has been considered as an independent risk predictor of macrosomia(Reference Di Cianni, Miccoli and Volpe45, Reference Mossayebi, Arab and Rahmaniyan46). It is possible that TAG status might help in interpreting this result. This interpretation is highly putative due to the limited evidence. This intriguing finding warrants further confirmation.
Key strengths of the present study are our direct measurements of methyl donor nutrition and its longitudinal design. Several limitations of our study should also be noted. First, the small number of participants limits the power of our study. Second, maternal mental health conditions that are considered to have an effect on infant growth(Reference Alder, Fink and Bitzer47) were unfortunately not assessed in our study. Third, a single measurement may not directly reflect the methyl donor nutrition during the whole pregnancy because of the variations in concentrations of some plasma one-carbon biomarkers such as choline and DMG throughout gestation(Reference Fernandez-Roig, Cavalle-Busquets and Fernandez-Ballart29). Blood sample collection during different stages of pregnancy from each study subject should be considered in future studies. Moreover, recruitment conducted in a tertiary referral hospital reduced the external validity of our results. However, our study was restricted to an assumingly healthy population, and thus findings can be extrapolated to the general population to some extent. Of note, 70 % of participants in our study were white-collar workers, representing a relatively high socio-economic status of our participants.
In conclusion, our findings showed that maternal plasma betaine showed a strong inverse relationship to birth weight. Maternal methionine status showed a protective effect on LGA risk. Maternal betaine and methionine may have a role in preventing fetal overgrowth. Given the implications of inappropriate fetal growth for later-life chronic disease risk, more investigations exploring the effect of maternal one-carbon biomarkers on fetal growth are warranted. Our results require further validation in larger mother–infant cohorts.
Acknowledgements
We gratefully acknowledge the cooperation of the study participants.
This work is supported by the National Key Research and Development Program of Reproductive Health and Major Birth Defects Control and Prevention (2016YFC1000400).
Y.-F. D. performed the statistical analyses and interpreted the results and wrote the paper; Y. W., J. Y. and Z.-Y. C. participated in the participant’s recruitment, data collection, blood sample collection and study design; Y.-F. D. and X.-F. Z. abstracted data from medical records; T.-C. W. and H.-F. S. offered help in the assay of biomarkers. X.-L. W. designed the research, supervised and contributed to manuscript writing. All authors revised the manuscript and read and approved the final manuscript.
The authors declare that they have no conflicts of interest.