Inflammatory diseases such as rheumatoid arthritis, asthma and hepatitis are the major causes of morbidity in humans. Today, it is well known that chronic inflammation also leads to the development of cancer, CVD and neurodegenerative diseases. Oedema formation in the paw is the result of a synergism between various inflammatory mediators that increase vascular permeability and/or the mediators that increase blood flow. Several experimental models of paw oedema have been described. Carrageenan (CAR)-induced paw oedema is widely used for determining the acute phase of inflammation. Histamine, 5-hydroxytryptamine and bradykinin are the first detectable mediators in the early phase of CAR-induced inflammation, whereas PG are detectable in the late phase of inflammation(Reference Albayrak, Uyanik and Odabasoglu1–Reference Odabasoglu, Halici and Cakir5). It is well known that the acute inflammatory process, in which vascular permeability increases and leucocyte migration occurs, involves inflammation mediators including neutrophil-derived active oxygen species and free radicals, such as H2O2 and superoxide, and the hydroxyl radicals, such as NO, PG and cytokines(Reference Tanas, Odabasoglu and Halici3–Reference Odabasoglu, Halici and Cakir5). Polymorphonuclear leucocytes that are the first cells to arrive at the inflammatory site in the body release free oxygen radicals and free hydroxyl radicals(Reference Tanas, Odabasoglu and Halici3–Reference Kumtepe, Odabasoglu and Karaca7). On the other hand, in many physiological functions of the body and the pathogenesis of inflammation, ion exchanges between intracellular and extracellular areas have been identified. It has been reported that increased intracellular Ca ion stimulates inflammation events and Ca channel blocker drugs diminish these events(Reference Tanas, Odabasoglu and Halici3–Reference Kumtepe, Odabasoglu and Karaca7). However, the chronic inflammation model, induced by subcutaneous implantation of foreign bodies, is used commonly in experiments(Reference Tanas, Odabasoglu and Halici3, Reference Odabasoglu, Halici and Cakir5, Reference Suleyman, Odabasoglu and Aslan8, Reference Hosseinzadeh, Ramezani and Salmani9). Chronic inflammation starts with the occurrence of proliferative cells(Reference Hosseinzadeh, Ramezani and Salmani9). These cells can be spread or can form a granuloma tissue. Inflammatory granuloma tissue is a typical example of chronic inflammation. On the other hand, granuloma formation is initialised by an antigen (for example, a cotton pellet). The cotton pellet stimulates the immune system, antibodies and production of IL. This stimulation results with the proliferation of lymphocytes and the formation of granulation tissue over the pellets(Reference Suleyman, Odabasoglu and Aslan8, Reference Kapu, Ngwai and Kayode10). Non-steroidal anti-inflammatory drugs and ulcers reduce granuloma tissue that is formed with cellular reactions by inhibiting granulocyte infiltration, while glucocorticoids do it by inhibiting fibrosis and macrophage function(Reference Odabasoglu, Halici and Cakir5, Reference Suleyman, Odabasoglu and Aslan8–Reference Kapu, Ngwai and Kayode10).
Reactive oxygen species (ROS) including free radicals such as hydroxyl radicals (OH∙) or superoxide anions () and non-free radical species such as H2O2 constantly cause lipid peroxidation (LPO) in tissues by attacking unsaturated fatty acids. Oxidative stress also plays an important role in the pathogenesis of many other diseases such as respiratory distress syndrome, rheumatoid arthritis, local or systemic inflammatory disorders, sepsis, diabetes, atherosclerosis and cancer(Reference Halici, Dengiz and Odabasoglu2, Reference Kapu, Ngwai and Kayode10–Reference Nishida, Ohta and Ishiguro18). On the other hand, aerobic organisms possess antioxidant defence systems including enzymatic and non-enzymatic antioxidants to combat the toxic effects of ROS. These antioxidants are superoxide dismutase (SOD), glutathione peroxidase (GPx), catalase (CAT), GSH, glutathione reductase (GR), tocopherols, vitamin C, β-carotene, vitamin A and flavonoids. Myeloperoxidase (MPx), found in azurophilic granules of mammalian neutrophils and in human monocytes, is involved in microbial killing and inflammatory tissue damage, and neutrophil infiltration into gastric mucosal tissues is a critical process in the pathogenesis of gastric lesions induced by non-steroidal anti-inflammatory drugs and ulcers(Reference Suleyman, Odabasoglu and Aslan8, Reference Cadirci, Oral and Odabasoglu19). NO, enzymatically produced from NO synthase (NOS), first discovered in the vascular endothelium, plays many important roles in health and diseases. NOS is present in several isoforms in a large number of cells besides endothelial cells, such as the constitutive endothelial NOS and neuronal NOS under physiological conditions and inducible NO synthase (iNOS), which is induced by cytokines under pathophysiological conditions(Reference Salvemini, Wang and Wyatt4, Reference Handy and More11, Reference Karaca, Odabasoglu and Kumtepe16–Reference Nishida, Ohta and Ishiguro18).
α-Lipoic acid (ALA, Fig. 1) is a naturally occurring essential co-enzyme in mitochondrial multienzyme complexes catalysing the oxidative decarboxylation of α-keto acids such as pyruvate, α-ketoglutarate and branched-chain α-keto acid(Reference Huong and Ide20–Reference Odabasoglu22). ALA has been shown to combat oxidative stress by quenching a variety of intracellular ROS(Reference Bilska and Wlodex21, Reference Odabasoglu22). In addition to ROS scavenging, ALA has also been shown to be involved in the recycling of other cellular antioxidants including vitamins C and E, and GSH(Reference Biewenga, Haene and Bast23). ALA has been demonstrated to be effective in preventing pathology in various experimental models in which ROS have been implicated, such as ischaemia–reperfusion injury(Reference Sehirli, Sener and Cetinel15), diabetes(Reference Hurdag, Ozkara and Citci24), hypertension(Reference Malinska and Winiarska25), radiation injury(Reference Demir, Demir and Ilhan26) and HIV activation(Reference Patrick27). On the other hand, in cancer cells, ROS also play a crucial role in cell growth and apoptosis regulation. ALA and its reduced form, dihydrolipoic acid, have been shown to inhibit proliferation and induce apoptosis of several cancer and transformed cell lines, while being less active towards normal non-transformed cells(Reference Wenzel, Nickel and Daniel28).

Fig. 1 Chemical structure of α-lipoic acid.
In the present study, we aimed to evaluate: (a) the reducing effects of ALA on acute (CAR induced) and chronic phases (cotton pellet granule) of inflammation, (b) the changes of LPO and antioxidant levels (SOD, GSH, CAT, glutathione S-transferase (GST), GPx and GR), and MPx and iNOS activities, as markers of acute inflammation, in paw tissues of rats and (c) in vitro antioxidant potency of ALA.
Materials and methods
Animals
A total of seventy-eight male Wistar rats, weighing 180–190 g, were provided from the Experimental Animal Laboratory of Ataturk University, the Experimental Animal Teaching and Research Center. The animals were housed in groups of five or six per cage for at least 5 d under controlled conditions of constant temperature/humidity and exposed to a 12 h light–dark cycle(29). Animals for acute inflammation experiments were assigned randomly to the control groups receiving either CAR (0·1 ml of 1 % CAR per animal) or water and to the experimental groups receiving CAR plus ALA at doses of 50, 100 and 200 mg/kg, indomethacin (IND) at a dose of 25 mg/kg and diclofenac (DIC) at a dose of 25 mg/kg. Besides, the animals were grouped similarly to evaluate chronic inflammation via the cotton pellet-induced granuloma test. The experiments were conducted according to the ethical norms approved by the Ethics Committee of the Experimental Animal Teaching and Research Center (no: B.30.2.ATA.0.70/144-4).
Chemicals
All chemicals for laboratory experiments were purchased from Sigma Chemical (Munich, Germany). Other compounds were obtained from various sources: ALA was obtained from Life Time (Istanbul, Turkey), DIC (Myadren 50 mg Tablet) was obtained from Fako (Istanbul, Turkey) and IND (Endosetin capsule) was obtained from Nobel (Istanbul, Turkey).
Carrageenan-induced paw oedema in rats
In this type of experiment, the effects of ALA and positive controls (IND and DIC) on CAR-induced paw oedema in rats were investigated(Reference Suleyman, Odabasoglu and Aslan8). The animals were divided into seven groups, each consisting of six rats (Table 1 and Fig. 2). ALA, CAR, IND and DIC solutions were prepared by dissolving in distilled water. Briefly, three doses of ALA (50, 100 and 200 mg/kg) and one dose of IND (25 mg/kg) and DIC (25 mg/kg) were administered orally to rats with the aid of gavages. The paw volumes of the animals were calculated plethysmometrically, and 0·1 ml of 1 % CAR was injected into the hind paw of each animal 1 h after the last dose. The change in paw volume was detected as five replicates every 60 min by plethysmometry. The anti-inflammatory potency of ALA was determined via comparison with the result obtained from the animals receiving equal volumes of positive controls (IND and DIC), CAR (control) and distilled water (negative control). The last group of animals (seventh group) was used for antioxidant enzyme research and is called the healthy group (no drug or CAR administration). The anti-inflammatory effects of these drugs were calculated by the following equation:

where D represents the percentage difference in paw volume after the administration of drugs to the rats, and C represents the percentage difference of volume in the control groups.
Table 1 Effects of α-lipoic acid (ALA), indomethacin (IND) and diclofenac (DIC) on carrageenan (CAR)-induced paw oedema (fourth hour) in rats†
(Mean values with their standard errors, n 6)

n, Number of animals.
Mean values were significantly different when compared to that of the control group: *P < 0·05, **P < 0·01.
† Three doses of ALA (50, 100 and 200 mg/kg), and one dose of IND (25 mg/kg) or DIC (25 mg/kg) were administered to rats by oral administration. Three doses of the ALA-treated group and one dose of the IND- and DIC-treated groups were compared with the CAR group. The paw volumes of the animals were calculated plethysmometrically, and 0·1 ml of 1 % CAR was injected into the hind paw of each animal 1 h after oral drug administration.
‡ Inhibition % in paw volumes in relation to control (carrageenan) group.

Fig. 2 Effects of three doses of α-lipoic acid (ALA) and one dose of indomethacin (IND) or diclofenac (DIC) on carrageenan (CAR)-injected rats, with time-dependent increase in the rat's paw volume after CAR injection. Three doses of ALA (50, 100 and 200 mg/kg) and one dose of IND (25 mg/kg) or DIC (25 mg/kg) were administered to rats by oral administration. The paw volumes of the animals were calculated plethysmometrically, and 0·1 ml of 1 % CAR was injected into the hind paw of each animal 1 h after oral drug administration. –♦–, CAR; –■–, CAR+IND; –▲–, CAR+DIC; – × –, CAR+ALA (50 mg/kg); , CAR+ALA (100 mg/kg); –●–, CAR+ALA (200 mg/kg).
Biochemical analysis of carrageenan-induced paw oedema in rats
Preparation of tissues homogenates
After the plethysmometric analyses, the activities of CAT, SOD, GST, GPx, GR, iNOS and MPx, and the amounts of LPO or total GSH in paw tissues of rat oedema were determined. To prepare the tissue homogenates, paw oedema tissues of each rat groups (n 6) were ground with liquid N2 in a mortar. The ground tissues (0·5 g each) were then treated with 4·5 ml of an appropriate buffer. The mixtures were homogenised on ice using an ultra-turrax homogeniser for 15 min. Homogenates were filtered and centrifuged by using a refrigerated centrifuge at 4°C. Then, these supernatants were used for the determination of the enzymatic activities. All the assays were carried out at room temperature as three parallel measurements.
Glutathione S-transferase activity
Total GST activity was determined as described by Habig & Jakoby(Reference Habig and Jakoby30). Briefly, the enzyme activity was assayed spectrophotometrically at 340 nm in a 4 ml cuvette containing 0·1 m-PBS (pH 6·5), 3 0mm-GSH, 30 mm-1-chloro-2,6-dinitrobenzene and tissue homogenate. Enzyme activity was expressed as nmol/min per mg protein.
Glutathione peroxidase activity
GPx activity was determined according to a previously reported method(Reference Lawrence and Burk31). The absorbance at 340 nm was recorded for 5 min. The activity was determined by measuring the amount of oxidised NADPH as μmol/min per mg tissue.
Glutathione reductase activity
GR activity was determined spectrophotometrically by measuring the rate of NADPH oxidation at 340 nm(Reference Carlberg and Mannervik32). Results are expressed as the amount of enzymes required to catalyse the oxidation of 1 μmol of NADPH/min per mg of tissue.
Catalase activity
Decomposition of H2O2 in the presence of a CAT was measured at 240 nm(Reference Aebi33). CAT activity was defined as the amount of enzymes required to decompose 1 nmol of H2O2/min, at 25°C and pH 7·8. Results are expressed as mmol/min per mg of tissue.
Superoxide dismutase activity
As outlined by Sun et al. (Reference Sun, Larry and Ying34), SOD estimation was based on the generation of superoxide radicals produced by xanthine and xanthine oxidase, which reacts with nitro blue tetrazolium to form formazan dye. SOD activity was then measured at 560 nm by the degree of inhibition of this reaction and is expressed as mmol/min per mg of tissue.
Inducible nitric oxide synthase activity
NOS activity was measured spectrophotometrically using the oxidation of oxyhaemoglobin to methaemoglobin by NO as described previously(Reference Knowles, Salter and Brooks35, Reference Feelisch and Noack36). The absorption difference between 401 and 421 nm was continuously monitored with a dual wavelength recording spectrophotometer (Thermo Spectronic-HEλIOS β, Cambridge, UK) at 37°C. For total NOS assay, the incubation medium contained 1·6 μm-oxyhaemoglobin, 200 μm-CaCl2, 1 mm-MgCl2, 100 μm-l-arginine, 100 μm-NADPH, 40 mm-potassium phosphate (pH 7·2), 1 mm-NG-nitro-l-arginine and 10 % (v/v) tissue extract with 50 mm-l-valine to inhibit arginase. For the cNOS (constitutive) assay, 1 mm-glycol diethyl ether diamine tetraacetic acid was added to the above-mentioned incubation medium without NG-nitro-l-arginine. Oxyhaemoglobin oxidation was confirmed as being caused by NO synthesis. iNOS activity was calculated by subtracting the cNOS activity from the total NOS activity.
Myeloperoxidase activity
MPx activity was measured according to the modified method of Bradley et al. (Reference Bradley, Priebat and Christensen37). The homogenised samples were frozen and thawed three times, and centrifuged at 1500 g for 10 min at 4°C. MPx activity in the supernatant was determined by adding 100 ml of the supernatant to 1·9 ml of a 10 mmol/l phosphate buffer (pH 6·0) and 1 ml of 1·5 mmol/l o-dianisidine dihydrochloride containing 0·0005 % (w/v) H2O2. The changes in absorbance at 450 nm of each sample were recorded on a UV–visible spectrophotometer (Fig. 3). MPx activity in tissues was expressed as μmol/min per mg tissue.

Fig. 3 Effects of α-lipoic acid (ALA), indomethacin (IND) and diclofenac (DIC) on changes in the activity of myeloperoxidase (MPx) enzyme in carrageenan (CAR)-injected rat paws (fifth hour). Three doses of ALA (50, 100 and 200 mg/kg) and one dose of IND (25 mg/kg) or DIC (25 mg/kg) were administered to rats by oral administration. The paw volumes of the animals were calculated plethysmometrically, and 0·1 ml of 1 % CAR was injected into the hind paw of each animal 1 h after oral drug administration. (The same experiment has been performed three times, and then data determined are expressed as means with standard errors of three parallel measurements. Three doses of ALA-treated group and one dose of IND- and DIC-treated groups were compared with the CAR group. The CAR group was compared with the healthy group.) Mean values were significantly different: *P < 0·05, **P < 0·01.
Total GSH determination
The amount of GSH in the paw oedema was measured according to the method of Sedlak & Lindsay(Reference Sedlak and Lindsay38). The paw oedema was collected by scraping, then it was weighed and homogenised in 2 ml of a 50 mm-Tris–HCl buffer containing 20 mm-EDTA and 0·2 m-sucrose at a pH of 7·5. The homogenate was centrifuged. After centrifugation at 4200 rpm for 40 min at 4°C, the supernatant was used to determine GSH using 5,5′-dithiobis(2-nitrobenzoic acid). Absorbance was measured at 412 nm using a spectrophotometer. The results of the GSH level in the paws were expressed as nmol/mg tissue.
Determination of lipid peroxidation or malonaldehyde formation
The levels of LPO were determined by estimating malonaldehyde using the thiobarbituric acid test(Reference Ohkawa, Ohishi and Yagi39). Briefly, the rat stomachs were promptly excised and rinsed with cold saline. To minimise the possibility of interference of Hb with free radicals, any blood adhering to the mucosa was carefully removed. The paw tissues were scraped, weighed and homogenised in 10 ml of 100 g/l KCl. The homogenate (0·5 ml) was added with a solution containing 0·2 ml of 80 g/l sodium laurylsulphate, 1·5 ml of 200 g/l acetic acid, 1·5 ml of 8 g/l 2-thiobarbiturate and 0·3 ml distilled water. The mixture was incubated at 98°C for 1 h. Upon cooling, 5 ml of n-butanol–pyridine (15:l) was added. The mixture was vortexed for 1 min and centrifuged for 30 min at 4000 rpm. The supernatant was measured at 532 nm. The standard curve was obtained using 1,1,3,3,-tetramethoxypropane. The recovery was over 90 %. All the samples were measured in duplicate. The results were expressed as nmol malonaldehyde/g of wet tissue.
Cotton pellet-induced granuloma test in rats
In this series of experiments, the effect of ALA on the proliferative phase of inflammation was investigated using the cotton pellet test(Reference Suleyman, Odabasoglu and Aslan8). Animals were divided into six groups each consisting of six rats (Table 2). Three groups of animals received ALA at doses of 50, 100 and 200 mg/kg body weight (wt) with the aids of gavages, while the other groups of animals received DIC at a dose of 25 mg/kg body wt and IND at a dose of 25 mg/kg body wt orally, and the control group received an equal volume of distilled water. Thirty minutes after administration of the drugs, the animals were anaesthetised using 25 mg/kg body wt sodium thiopental. Under sterilised conditions, cotton pellets of 7 (sem 1) mg were implanted subcutaneously in the interscapular area. ALA, DIC and IND were administered once a day for 7 d at the doses indicated earlier. On the eighth day, the animals were killed via high-dose anaesthesia, and the cotton pellets with the granuloma tissue about them were removed. The wet weights of these pellets were measured, and the anti-proliferative effects of ALA, DIC and IND were determined by comparison with the control group.
Table 2 Effects of α-lipoic acid (ALA), indomethacin (IND) and diclofenac (DIC) on cotton pellet-induced granuloma test in rats†
(Mean values with their standard errors)

n, Number of animals.
Mean values were significantly different when compared with that of the control group: **P < 0·01.
† Three doses of ALA (50, 100 and 200 mg/kg) and one dose of IND (25 mg/kg) or DIC (25 mg/kg) were administered to rats by oral administration. At 30 min after administration of the drugs, cotton pellets were implanted subcutaneously in the interscapular area under anaesthesia. ALA, DIC and IND were administered once a day for 7 d. On the eighth day, the cotton pellets with the granuloma tissue about them were removed. Three doses of the ALA-treated group, and one dose of the IND- or DIC-treated groups were compared with the control group.
‡ Inhibition in granuloma weights in relation to the control (untreated) group.
Total antioxidant activity assays
The total antioxidant activities of ALA were determined by the thiocyanate method(Reference Tanas, Odabasoglu and Halici3, Reference Odabasoglu, Aslan and Cakir40) and compared with known antioxidants, trolox and ascorbic acid. Briefly, each sample (1 mg) in 1 ml distilled water was mixed with 5 ml linoleic acid emulsion (0·02 m, pH 7·0) and 5 ml of the phosphate buffer (0·2 m, pH 7·0). The linoleic acid emulsion was prepared by mixing 0·5608 g of linoleic acid with 0·5608 g of Tween-20 as an emulsifier and 100 ml of the phosphate buffer, and then the mixture was homogenised. The reaction mixture was incubated at 37°C. Aliquots of 0·1 ml were taken at different intervals during incubation. The degree of oxidation was measured according to the thiocyanate method by sequentially adding 4·7 ml ethanol (75 %), 0·1 ml ammonium thiocyanate (30 %), 0·1 ml sample solution and 0·1 ml ferrous chloride (0·02 m, in 3·5 % HCl). The mixture was allowed to stand for 3 min and then the peroxide value was determined by reading the absorbance at 500 nm using the UV-visible spectrophotometer (Thermo Spectronic-HEλIOS β). A control was performed with linoleic acid but without the extracts. Inhibition % was calculated using the following formula:

Trolox and ascorbic acid solutions prepared in the conditions mentioned earlier were used as positive controls.
Statistical analyses
Data of enzyme activities and inflammation were subjected to one-way ANOVA, with the presence of negative and positive controls, by using SPSS 11.0 software (SPSS, Inc., Chicago, IL, USA). Differences among the groups were attained using the least significant difference option, and significance was declared at P < 0·05, 0·01 and 0·005.
Results
Carrageenan-induced paw oedema
Intraplantar injection of CAR in rats led to a quadratic and time-dependent increase in paw volume (Fig. 2). The increase in paw volume was observed at 1 h and was maximal at 4 h after the administration. However, CAR-induced paw oedema was reduced in a dose-dependent manner by treatment with ALA at 1, 2, 3, 4 and 5 h after an injection of CAR (Fig. 2). At doses of 100 and 200 mg/kg, ALA significantly (P < 0·05 and 0·01) reduced CAR-induced paw oedema by 29·6 and 40·7 %, respectively, at the fourth hour (Table 1). At doses of 25 mg/kg, treating DIC and IND also significantly reduced the oedema by 44·4 and 55·5 %, respectively. The paw volume increased by 0·54 ml in the control group relative to the baseline values, while increases of 0·44, 0·38, 0·32, 0·24 and 0·30 ml were observed in the groups administered with 50, 100 and 200 mg/kg of ALA, IND and DIC, respectively. The present results show that IND, DIC, and 100 and 200 mg/kg of ALA (Fig. 2) had significant anti-inflammatory effects against the paw oedema caused by CAR. The anti-inflammatory effect of ALA was also weaker than that of DIC and IND, which inhibit PG synthesis by cyclo-oxygenase.
Comparison of enzymatic activities and lipid peroxidation or GSH levels in rat paw tissues
In order to explore the effects of antioxidant defences on the inflammation process in all paw tissues, the activities of antioxidant enzymes (GST, GPx, GR SOD and CAT) and the amounts of GSH and LPO were evaluated. The results are presented in Tables 3 and 4 with Figs 4 and 5. Table 3 shows that the activities of GPx, GR and SOD for CAR-injected groups were lower, and GST and CAT activities were higher than those for the healthy rat group. However, compared with GST, GPx and SOD levels in ALA-administered paw tissues, the opposite results were found for the activities of these enzymes in CAR-injected tissues. In contrast to CAR-injected paws, DIC and IND increased the activities of GPx and GR (P < 0·05) and decreased CAT activity (P < 0·05). Table 3 shows that the activity of GST was decreased by the administration of 50 and 100 mg ALA per kg (P < 0·05), while the activity of GR was reduced during CAR oedema and this reduction was not affected by all the doses of ALA (P>0·05). Similarly, DIC decreased GST activity but increased GR activity (P < 0·05). However, IND increased both the activity of GST elevated by CAR further and the activity of GR alleviated by CAR. The activity of GPx was 22·33 in healthy paws. This score was decreased by CAR to 21·44 (P>0·05), but it was increased by 50 and 100 mg/kg doses of ALA (P < 0·05 and P < 0·01), DIC (P < 0·05) and IND (P>0·01). However, compared with the activities of the CAT and SOD enzymes in ALA-, IND- and DIC-administered paw tissues, different results were found. The injected CAR increased CAT activity, while decreased SOD activity in paw tissues. In contrast to CAR-injected paws, all the doses of ALA and DIC increased SOD activity (P < 0·05), while DIC and IND decreased CAT activity (P < 0·05). On the other hand, CAT activity was elevated significantly (P < 0·01) by all the doses of ALA further (Table 4). Likewise, both IND with DIC and all the doses of ALA had an increasing effect (P < 0·05) on the level of GSH alleviated by CAR (Fig. 4), and in contrast to CAR-injected paws (Fig. 5), all the doses of ALA and DIC decreased the amount of LPO (P < 0·01).
Table 3 Effects of α-lipoic acid (ALA), indomethacin (IND) and diclofenac (DIC) on changes in the activities of some glutathione metabolism enzymes ((glutathione S-transferase (GST), glutathione peroxidase (GPx) and glutathione reductase (GR)) in CAR-injected paws (fifth hour) of rats†
(Mean values with their standard errors of three parallel measurements)

n, Number of animals.
Mean values were significantly different when compared to that of control group: *P < 0·05, **P < 0·01.
† Three doses of ALA (50, 100 and 200 mg/kg), and one dose of IND (25 mg/kg) or DIC (25 mg/kg) were administered to rats by oral administration. Three doses of the ALA-treated group, and one dose of the IND- or DIC-treated groups were compared with the CAR group. The CAR group was compared with the healthy group. The paw volumes of the animals were calculated plethysmometrically, and 0·1 ml of 1 % CAR was injected into the hind paw of each animal 1 h after oral drug administration.
‡ The same experiment was performed three times and then data determined are expressed as means with their standard errors of three parallel measurements.
Table 4 Effects of α-lipoic acid (ALA), indomethacin (IND) and diclofenac (DIC) on changes in the activity of catalase (CAT) and superoxide dismutase (SOD) enzymes in CAR-injected paws (fifth hour) of rats†
(Mean values with their standard errors of three parallel measurements)

n, Number of animals.
Mean values were significantly different when compared to that of the control group: *P < 0·05, **P < 0·01.
† Three doses of ALA (50, 100 and 200 mg/kg), and one dose of IND (25 mg/kg) or DIC (25 mg/kg) were administered to rats by oral administration. Three doses of the ALA-treated group and one dose of the IND- or DIC-treated groups were compared with the CAR group. The CAR group was compared with the healthy group. The paw volumes of the animals were calculated plethysmometrically, and 0·1 ml of 1 % CAR was injected into the hind paw of each animal 1 h after oral drug administration.
‡ The same experiment was performed three times.

Fig. 4 Effects of α-lipoic acid (ALA), indomethacin (IND) and diclofenac (DIC) on changes in level the of GSH in carrageenan (CAR)-injected rat paws (fifth hour). Three doses of ALA (50, 100 and 200 mg/kg) and one dose of IND (25 mg/kg) or DIC (25 mg/kg) were administered to rats by oral administration. The paw volumes of the animals were calculated plethysmometrically, and 0·1 ml of 1 % CAR was injected into the hind paw of each animal 1 h after oral drug administration. (The same experiment has been performed three times and then data determined are expressed as means with standard errors of three parallel measurements. Three doses of the ALA-treated group, and one dose of the IND- and DIC-treated groups were compared with the CAR group. The CAR group was compared with the healthy group.) Mean values were significantly different: *P < 0·05, **P < 0·01.

Fig. 5 Effects of α-lipoic acid (ALA), indomethacin (IND) and diclofenac (DIC) on changes in the amount of lipid peroxidation (LPO) in carrageenan (CAR)-injected rat paws (fifth hour). Three doses of ALA (50, 100 and 200 mg/kg) and one dose of IND (25 mg/kg) or DIC (25 mg/kg) were administered to rats by oral administration. The paw volumes of the animals were calculated plethysmometrically, and 0·1 ml of 1 % CAR was injected into the hind paw of each animal 1 h after oral drug administration. (The same experiment has been performed three times and then the data determined are expressed as means with standard errors of three parallel measurements. Three doses of the ALA-treated group, and one dose of the IND- and DIC-treated groups were compared with the CAR group. The CAR group was compared with the healthy group.) Mean values were significantly different: **P < 0·01.
In the present study, changes in MPx activity of paw tissues were widely used as an index of neutrophil infiltration in gastric and inflammatory tissue damage. MPx activity was determined by using an appropriate method. It is observed from Fig. 3 that the administration of CAR significantly (P < 0·01) increased the MPx activity. In contrast to CAR injection, the administration of the 50 (P < 0·05), 100 and 200 (P < 0·01) mg/kg doses of ALA and 25 mg/kg dose of IND reduced the level of MPx; however, DIC did not indicate any effect. Separately, iNOS activity in the rat paw tissues was also given in the Table 5. The intraplantar injection of CAR increased significantly iNOS activities (P < 0·01) in paw homogenates at the fifth hour. The activity of iNOS was 0·14 in healthy paws. This score was elevated by CAR to 0·36 (P < 0·05), but it was alleviated by CAR+DIC, CAR+IND and CAR+ALA (50, 100 and 200 mg/kg doses) to 0·18, 0·22, 0·17, 0·13 and 0·11, respectively (P < 0·01).
Table 5 Effects of α-lipoic acid (ALA), indomethacin (IND) and diclofenac (DIC) on changes in the activity of inducible nitric oxide synthase (iNOS) enzyme in CAR-injected paws (fifth hour) of rats†
(Mean values with their standard errors of three parallel measurements)

n, Number of animals.
Mean values were significantly different when compared to that of control group: **P < 0·01.
† Three doses of ALA (50, 100 and 200 mg/kg), and one dose of IND (25 mg/kg) or DIC (25 mg/kg) were administered to rats by oral administration. Three doses of the ALA-treated group, and one dose of the DIC- and IND-treated groups were compared with the CAR group. The CAR group was compared with the healthy group. The paw volumes of the animals were calculated plethysmometrically, and 0·1 ml of 1 % CAR was injected into the hind paw of each animal 1 h after oral drug administration.
‡ The same experiment was performed three times.
Cotton pellet-induced granuloma test
As shown in Table 2, the mean weight of the cotton pellets in the ALA (50, 100 and 200 mg/kg body wt) groups was 146 (sem 3·8), 141 (sem 3·8) and 136 (sem 4·1) mg, respectively, while it was 108 (sem 4·9) and 73 (sem 5·71) mg in the DIC (25 mg/kg body wt) and IND (25 mg/kg body wt), respectively. According to these results, the anti-proliferative effect of ALA (50, 100 and 200 mg/kg body wt) groups was shown to be 67·7, 68·9 and 70·6 %, respectively, and of DIC was 76·1 % and of IND was an 83·8 % response. The present results show that ALA in all the doses also has significant (P < 0·01) anti-inflammatory effects on the chronic inflammation process.
In vitro antioxidant activity of α-lipoic acid
ROS had negative effects on the pathogenesis of inflammation and gastric ulcer. Because of this, antioxidants may be effective on the pathogenesis of these diseases. Therefore, in the present study, the in vitro antioxidant action of ALA was also determined using thiocyanate methods and compared to the positive controls, trolox and ascorbic acid (Table 6). ALA in 5 mg acted as a powerful antioxidant by inhibiting the peroxidation of linoleic acid with 79·1 %. In particular, in 1 and 2·5 mg, ALA showed weak antioxidant potential.
Table 6 Antioxidant activity of three doses of α-lipoic acid (ALA) and one dose of positive controls (Trolox and ascorbic acid) on linoleic acid oxidation, in vitro
(Mean values with their standard errors)
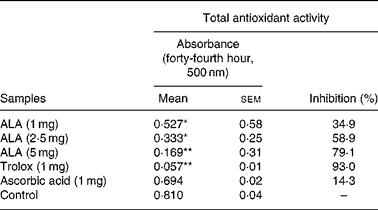
Mean values were significantly different when compared to that of the control group: *P < 0·05, **P < 0·01.
Discussion
Inflammatory processes are the physiological response of the organism to different stimulants such as trauma, infections or immunological mechanisms. The arachidonic acid cascade is highly activated during inflammation, resulting in the formation of eicosanoids, and it is mediated by cyclo-oxygenase and 5-lipoxygenase enzymes. These complex inflammatory reactions involve the release of a wide variety of inflammatory mediators, i.e. PG, thromboxanes and leukotrienes. Although drugs with a central mechanism of anti-pyretic and anti-inflammatory actions are known to down-regulate fever and inflammation, the role of the antioxidant mechanism pathway in mediating the action of such agents is still unknown.
In the present study, the anti-inflammatory effects of the ALA, a highly effective antioxidant agent, were investigated. The effect of ALA on the acute phase of inflammation was observed in the CAR-induced paw oedema test, and its anti-inflammatory potency was compared to DIC and IND. In our experiment, the mean paw volume of the control group reached its peak at the fourth hour. The mean paw volume in the groups receiving CAR, ALA, DIC and IND started to decrease at the fourth hour. At doses of 50, 100 and 200 mg/kg, ALA significantly (P < 0·01) reduced CAR-induced paw oedema by 18·5, 29·6 and 40·7 %, respectively, at the fourth hour (Table 1). DIC and IND, which significantly reduced the increase in paw volume by 44·4 and 55·5 %, respectively, showed a greater effect in this model of inflammation.
CAR injection into the rat paw provokes a local, acute inflammatory reaction which is a suitable method for evaluating anti-inflammatory agents(Reference Halici, Dengiz and Odabasoglu2–Reference Odabasoglu, Halici and Cakir5, Reference Suleyman, Odabasoglu and Aslan8). It appears that the delayed onset of CAR-induced oedema has been linked to inflammation mediators such as NO, PG, cytokines and free radicals, such as H2O2, superoxide, and hydroxyl radical, as well as to the release of other neutrophil-derived mediators(Reference Halici, Dengiz and Odabasoglu2–Reference Odabasoglu, Halici and Cakir5, Reference Karaca, Odabasoglu and Kumtepe16, Reference Dengiz, Odabasoglu and Halici17). In order to explore the effects of antioxidant defences on the acute inflammation process in all paw tissues, the levels of antioxidant parameters were evaluated.
The GST (E.C. 2.5.1.18) are a multigene family of isoenzymes responsible for detoxification of xenobiotics in aerobic organisms. Endogenous substrates include the toxic products of tissue damage, including the hydroperoxide products of oxidative damage (including lipid peroxides), and aromatic xenobiotics. The present study determined that GST activity was inhibited by both ALA and DIC in CAR-induced paw tissues in which CAR significantly increased (Table 3). El Midaoui et al. (Reference El Midaoui, Elimadi and Wu41) reported that high-glucose feeding rapidly induced hypertension and that the plasma and aorta GST activities of rats were decreased by ALA administration.
Although oxygen intermediates are highly reactive and potentially cytotoxic, their formation is not typically deleterious to the cells, because these ROS are neutralised by endogenous enzymes and free radical scavengers. First, SOD catalysing the dismutation of superoxide anions () to H2O2 and GSH-related enzymes preserving GSH status plays an important role in the antioxidant defence system by ensuring the degradation of these species into less harmful compounds(Reference Halici, Dengiz and Odabasoglu2, Reference Tanas, Odabasoglu and Halici3, Reference Cadirci, Altunkaynak and Halici14, Reference Karaca, Odabasoglu and Kumtepe16, Reference Halici, Karaca and Keles42). From the present study (Table 4), we have established that ALA and DIC increase SOD activity, which was inhibited by CAR in rat paw tissues. The similar results were reported by Selvakumar et al. (Reference Selvakumar, Prahalathan and Sudharsan43) in cyclophosphamide-induced rat sperm and by Kokilavani et al. (Reference Kokilavani, Devi and Sivarajan44) in arsenic-induced toxicity of rats. Separately, Khattab(Reference Khattab45) reported the importance of superoxide anion in CAR-induced acute inflammation and highlighted that the potential anti-inflammatory effects of SOD mimetic are mediated via the superoxide anion scavenging in acute inflammation. SOD destroys the highly reactive radical superoxide by converting it into the less reactive peroxide (H2O2), which can be destroyed by a CAT reaction. CAT is a highly reactive enzyme, reacting with H2O2 to form water and molecular oxygen and can form methanol, ethanol, formic acid or phenols via donating hydrogen. In the present study (Table 4), we established that IND and DIC decreased, but all the doses of ALA increased further CAT activity, which was increased by CAR in rat paw tissues. On the other hand, it was reported by Selvakumar et al. (Reference Selvakumar, Prahalathan and Sudharsan43) that ALA has an increasing effect on the activity of the CAT enzyme in rats.
Separately, similar to SOD, we showed that GPx activity was elevated by one dose of DIC and IND, or 100 and 200 mg/kg doses of ALA, which was alleviated by CAR in rat paw tissues (Table 3). Decrease in the activity of GPx during CAR administration indicates the reduction in the levels of GSH and increase in the levels of peroxides. Moreover, the fact that arsenic decreased Se levels in rats(Reference Lawrence and Burk31), which acts as a cofactor for GPx, might be a reason for the decrease in the activity of GPx.
On the other hand, Chen et al. (Reference Chen, Kamal and Hannon46) suggested that CAT stimulated the expression of mRNA and protein for cyclo-oxygenase-2 in rat aortic smooth muscle cells, despite not affecting the expression of either mRNA or protein for cyclo-oxygenase-1. That is, CAT exerted a biphasic effect on PG synthesis and enhanced PG production by external administrations in small concentrations. This suggested that at small concentrations, CAT administration may cause inflammation as reflected by increased cyclo-oxygenase-2 activity. One of the factors causing the formation of CAR-induced acute inflammation process is possibly an augmentation of CAT activity, which was ascertained in the results of the present experiment.
Reduced GSH having pleiotropic roles, including the maintenance of cells in a reduced state, serves as an electron donor for certain anti-oxidative enzymes (e.g. GPx) and the formation of conjugates with some harmful endogenous and xenobiotic compounds via catalysis of GST. The anti-inflammatory effects of ALA can be supported by GSH levels in CAR-induced rat paw tissues. Furthermore, treatment with ALA enhanced GSH level which had been decreased by CAR in the rat paws (Fig. 4). In other studies, it was shown that ALA increased GSH levels in arsenic-induced toxicity of rats(Reference Kokilavani, Devi and Sivarajan44), cyclophosphamide-induced rat sperm(Reference Selvakumar, Prahalathan and Sudharsan43), ischaemia–reperfusion injury of rats(Reference Sehirli, Sener and Cetinel15) and 2,4,6-trinitrobenzene sulphonic acid-induced gut inflammation of rats(Reference Feelisch and Noack36).
Since ALA raises intracellular GSH levels, it has been used for treating diseases in which oxidative stress plays a major role. On the other hand, GR indirectly participates in the protection of cells against oxidative stress. The enzymatic activities of GR have been investigated in various tissues under physiological and pathological conditions(Reference Shacter, Lopez and Pati47). Table 3 shows that GR activity was activated by both IND and DIC in CAR-induced paw tissues in which CAR significantly inhibited. However, ALA has no effect on the GR activity.
A primary measure of oxidative damage in tissues is LPO, where thiobarbituric acid is measured as the index of LPO. The injection of the inflammation-inducing agent, CAR, considerably increases the levels of LPO(Reference Govindarajan, Vijayakumar and Rao48). In the present investigation, there is a significant increase in the levels of LPO in the paws of CAR-injected rats (Fig. 5). The increase in the levels of LPO is due to the increased release of Fe that is believed to be involved in the Fenton type of reaction. This ‘free Fe’ is considered as a potent enhancer of ROS formation, as exemplified by the reduction of H2O2 with the generation of highly aggressive hydroxyl radicals. However, administrations of CAR plus ALA, IND and DIC significantly (P < 0·01) alleviated the amount of LPO. Similar results related to effects of ALA on LPO have been reported by the literature(Reference Sehirli, Sener and Cetinel15, Reference Selvakumar, Prahalathan and Sudharsan43, Reference Kokilavani, Devi and Sivarajan44). In the present study, ALA pre-treatment significantly reversed the increase of lipid peroxide and the altered antioxidant status. The increase in LPO reported here may be the result of increased production of free radicals and/or a decrease in antioxidant status. ALA scavenges the singlet oxygen, H2O2 and hydroxyl radicals, and also chelates the ferrous ions involved in the production of hydroxyl radicals(Reference Bilska and Wlodex21, Reference Odabasoglu22).
MPx is an enzyme found primarily in azurophilic granules of neutrophils, which is used as a marker for tissue neutrophil content, and its inhibition implicates the presence of anti-inflammatory activity(Reference Bradley, Priebat and Christensen37). In the present study, we determined that MPx activity significantly (P < 0·05) increased in the paw at 5 h after CAR injection when compared with healthy rats (Fig. 3). Moreover, MPx activity was alleviated by 50, 100 and 200 mg/kg doses of ALA more than IND. On the other hand, DIC had no effect on the activity of MPx in CAR-injected rat paws. It was reported that the release of MPx from inflammatory cells is another indicator to evaluate the degree of inflammation, and anti-inflammatory drugs may also exert their effects via the inhibition of MPx pathways(Reference El Midaoui, Elimadi and Wu41, Reference Shacter, Lopez and Pati47, Reference Paino, Ximenes and Fonseca49). A variety of anti-inflammatory drugs depress the increases in MPx activity in the case of inflammation(Reference Paino, Ximenes and Fonseca49). Our data show that ALA and IND significantly decreased MPx activity (Fig. 4). The effect of DIC on MPx activity is inconsistent(Reference Matsui, Murata and Kobayashi50) because of variations in different tissues. Each molecule of ALA contains two atoms of sulphur (Fig. 1). Because of its high sulphur content, ALA can inactivate MPx activity in rat paws. The decrease in MPx activity in response to ALA could be linked to the sulphur present in its chemical structure. Interestingly, most of the non-steroidal anti-inflammatory drugs and other drugs contain a thiol group (e.g. d-penicillamine and tiopronin) and are widely used in the treatment of rheumatoid arthritis(Reference Bonta, Parnham, Vincent, Ellis and West51) and related diseases. It was shown that ALA treatment was effective in improving tissue LPO, and MPx activity increased and decreased GSH levels in 2,4,6-trinitrobenzene sulphonic acid-induced gut inflammation and ischaemia–reperfusion injury in rats(Reference Sehirli, Sener and Cetinel15, Reference Kolgazi, Jahovic and Yüksel52).
A growing body of evidence suggests that sustained production of NO resulting from up-regulation of the inducible isoform of NOS after CAR injection may cause an inflammatory response in rat paws. It is well established that iNOS levels under normal physiological conditions are low; however, the administration of CAR causes the induction of iNOS(Reference Cuzzocrea, Bruscoli and Crisafulli53, Reference Hurdag, Uyaner and Gurel54).
In the present study, it was demonstrated that CAR administration in rats resulted in significant increases in iNOS levels in paws which were attenuated by ALA pre-treatment (Table 5). Similarly, it was reported that ALA alleviated the activity of iNOS(Reference Hurdag, Ozkara and Citci24). In addition to ROS, excessive production of reactive nitrogenous species, especially NO through the activation of the constitutive as well as the expression of iNOS, has been shown to play an important role in various models of inflammation(Reference Tanas, Odabasoglu and Halici3, Reference Salvemini, Wang and Wyatt4, Reference Handy and More11). It has been also widely accepted that NO produced by iNOS is cytotoxic(Reference Tanas, Odabasoglu and Halici3, Reference Nishida, Ohta and Ishiguro18, Reference Odabasoglu, Suleyman and Aslan55, Reference Jean, Chen and Duh56). In view of these results, it can be suggested that ALA is a cytoprotective compound.
The molecular regulation of iNOS expression resulting in excessive amounts of NO released in paws is a complex event and requires the transcription factor NF-κB. It has been shown that down-regulation of NF-κB with antioxidants resulted in decreases in the amount of NO released in the circulation, a treatment effect attributed to the reduction in iNOS expression(Reference van den Berg, Haenen and van den Berg57). Another explanation for the reduction in NO levels in paws might be due to the direct scavenging effect of NO by the sulphydryl groups of ALA(Reference Biewenga, Haene and Bast23). It has been demonstrated that oxidative stress up-regulates and antioxidants down-regulate the pleiotropic transcription NF-κB, a DNA-binding protein that induces the expression of cytokines and vascular adhesion molecules(Reference van den Berg, Haenen and van den Berg57). The ability of ALA to inhibit the activation of NF-κB has been demonstrated with human T-cells exposed to TNF-α or phorbol-12-myristate-13-acetate or cultured aortic endothelial cells challenged with advanced glycation end products. Cho et al. (Reference Cho, Lee and Lee58) showed that ALA decreased activations of NF-κB and pro-inflammatory chemical mediators, such as IL-4 and IL-5 in BAL fluids and ovalbumin-specific IgE. Similarly, Zhang & Frei(Reference Zhang and Frei59) reported that ALA may also affect NF-κB-dependent expression of many other inflammatory genes, such as IL-1 and IL-6, a tissue factor and TNF-α in numerous cell types. These investigations suggest that the functional mechanism underlying the therapeutic efficacy of ALA as an antioxidant on initiation and progression of various inflammatory diseases may be related to inhibition of NF-κB activation through decreased oxidative stress and subsequent reduction in pro-inflammatory chemical mediators.
In the chronic inflammation experiment, ALA significantly inhibited the weight of the cotton pellets inserted under the skin of rats. The effects of ALA, IND and DIC on cotton pellet-induced granuloma in rats are shown in Table 2. At doses of 50, 100 and 200 mg/kg, ALA as well as DIC and IND (25 mg/kg) inhibited markedly (P < 0·01) granuloma formation surrounding the pellets compared with the control group. ALA (200 mg/kg) produced a maximum inhibition of 70·6 %, while 50 and 100 mg/kg of ALA produced 67·7 and 68·9 % inhibition in granuloma weight, respectively. IND and DIC significantly decreased the weight of implanted cotton pellets 83·8 and 76·1 % in comparison with the control group, respectively.
It is known that inflammatory granuloma is a typical response of a chronic inflammatory process, and it has been established that the dry weight of the pellets is well correlated with granulomatous tissue. The cotton pellet granuloma method is frequently used to investigate the chronic phase of inflammation(Reference Halici, Dengiz and Odabasoglu2–Reference Odabasoglu, Halici and Cakir5, Reference Suleyman, Odabasoglu and Aslan8, Reference Olajide, Makinde and Awe60). The anti-proliferative potency of IND and DIC was higher than that of ALA in the cotton pellet-induced granuloma test. Little data have been reported about mediators of chronic inflammation. However, the chronic inflammation model, induced by subcutaneous implantation of foreign bodies, is used commonly in experiments. Chronic inflammation starts with the occurrence of proliferative cells. These cells can be spread or can form a granuloma tissue. Inflammatory granuloma tissue is a typical example of chronic inflammation(Reference Olajide, Makinde and Awe60). Granuloma formation is initialised by an antigen (for example, a cotton pellet). The cotton pellet stimulates the immune system, antibodies and the production of IL. This stimulation results with the proliferation of lymphocytes and the formation of granulation tissue over the pellets. Non-steroidal anti-inflammatory drugs and ulcers reduce granuloma tissue that is formed with cellular reactions by inhibiting granulocyte infiltration, while glucocorticoids do it by inhibiting fibrosis and macrophage functions.
It was reported that ALA on initiation and progression of various inflammatory diseases might be related to the inhibition of NF-κB activation through decreased oxidative stress and subsequent reduction in pro-inflammatory chemical mediators(Reference Cho, Lee and Lee58, Reference Zhang and Frei59). Antioxidants have various mechanisms such as prevention of chain initiation, binding of transition metal ion catalysts, decomposition of peroxides, prevention of continued hydrogen abstraction and radical scavenging(Reference Tanas, Odabasoglu and Halici3, Reference Odabasoglu, Halici and Cakir5, Reference Dengiz, Odabasoglu and Halici17). In the present study, ALA (1, 2·5 and 5 mg/ml), ascorbic acid (1 mg/ml) and Trolox (1 mg/ml) after 44 h incubation with linoleic acid emulsion are also summarised as inhibition (%) in Table 6. Trolox and ascorbic acid were used as positive controls for the hydrophilic antioxidants. In general, the oxidation of linoleic acid was inhibited by ALA, ascorbic acid and Trolox, as observed when compared with the control. However, the antioxidant activities of all the doses of ALA and Trolox except for ascorbic acid in comparison with the control were statistically significant (P < 0·05 and 0·01). The 1, 2·5 and 5 mg/ml doses of ALA exhibited potent antioxidant activities with 34·9, 58·9 and 79·1 % inhibition of linoleic acid peroxidation, respectively. However, the antioxidant activities of ALA were lower than that of Trolox. The present results showed that ALA has a strong anti-oxidative potential (Table 6). Given this, it must be considered that the antioxidant potential of ALA might play an important role in the prevention of inflammation processes in rats.
In conclusion, the current results showed that tested agents (ALA, IND and DIC) reduced paw volume, at a smaller magnitude in response to ALA than IND and DIC. The level of antioxidant system enzymes (GST, GPx, GR, SOD and CAT, MPx, iNOS) and levels of GSH or LPO were adversely affected by oedema induction. ALA ameliorated the adverse effects of oedema on these enzymes. In addition, ALA exhibited an anti-inflammatory effect on the cotton pellet-induced chronic inflammation in rats. The anti-inflammatory properties of ALA which has a strong anti-oxidative potency could be related to its positive effects on the antioxidant system in a variety of rat tissues. In the light of the present results, we can suggest that ALA, an over-the counter drug with no side effects, possesses a dual beneficial effect, such that it might improve the prognosis of acute and chronic inflammatory diseases when used alone and/or combined with a known anti-inflammatory agent.
Acknowledgements
None of the authors has a commercial interest, financial interest and/or other relationship with manufacturers of pharmaceuticals, laboratory supplies and/or medical devices or with commercial providers of medically related services. F. O. was the main moderator of the study. F. O., M. H., F. A. and Y. B. performed the biochemical investigation in the present study. Z. H., E. C. and H. S. performed the pharmacologic investigations in the present study. H. A. contributed in pharmacological paw experiments as an orthopaedist. A. C. contributed in both writing period and statistical analyses.