The aetiology of multiple sclerosis (MS), a disease characterised by chronic inflammation of the central nervous system, is unknown, but an autoimmune and/or infectious component has been implicated(Reference Brück1–Reference Hunter and Hafler5). A distinct feature of the MS brain is the appearance of lymphocytes around small blood vessels(Reference Brown4, Reference Adams6). Fatty acids, particularly the n-6 fatty acids, have also been shown to have a role in the pathogenesis and treatment of the disease(Reference Harbige and Sharief2). Phospholipids make up the basic structure of all cell membranes and the type of fatty acids present in the phospholipids are closely related to the biological features of the phospholipids(Reference Caret, Denniston, Topping and Wheatley7, Reference Manzoli, Stefoni and Manzoli-Guidotti8). Membrane lipids differ in their headgroup and hydrocarbon chains(Reference Barenholz, Cevc, Baszkin and Norde9). The polar groups can be choline, ethanolamine, serine, inositol, inositol phosphates or glycerol(Reference Koay and Walmsley10). The phospholipid sphingomyelin (SM), a sphingolipid, is a major component of myelin in the brain(Reference Koay and Walmsley10). Phosphatidylcholine (PC) is the most abundant phospholipid in animal cell membranes, and phosphatidylethanolamine (PE) the second most(Reference Williams11, Reference Harlos and Aibl12). The fatty acids contained within the different phospholipid fractions can be saturated or unsaturated (mono- or poly-), and the PUFA are subdivided into different series, the n-9, n-6 and n-3 subtypes(Reference Koay and Walmsley10). Membrane fatty acids fulfill a variety of roles within immune cells and changes in the membrane phospholipid composition influence immune cell function in a variety of ways(Reference Calder13). These include alterations in the physical properties of the membrane, effects on cell signalling pathways, alterations in the pattern of lipid mediators produced, and an increase in eicosanoid production as part of the inflammatory process(Reference Calder13, Reference Khanapure, Garvey and Janero14).
Measurements of fatty acids in biological fluids and cell membranes including leucocytes from MS patients have been inconsistent. Significant decreases in the relative percentages of 18 : 2n-6 (linoleic acid), the precursor for 20 : 4n-6, have been shown in peripheral blood lymphocyte membranes in MS patients, with accompanying lower plasma levels(Reference Tsang, Belin and Monro15, Reference Cheravil16), while Fisher et al. (Reference Fisher, Johnson and Natale17) showed a similar decrease in the leucocytes of MS patients, but not in their plasma. Therefore in the present study our aim was to investigate the fatty acid composition within the different phospholipid fractions in peripheral blood mononuclear cell (PBMC) membranes of MS patients, using stringent exclusion criteria, and correlate it with severity of neurological outcome as measured by the Kurtzke Expanded Disability Status Scale (EDSS) and its Functional System Scores (FSS)(Reference Kurtzke18). The exclusion criteria used in the present study included the use of fatty acid supplements, interferon and cortisone or presence of a second disease for both MS patients and control subjects. In addition we have used plasma C-reactive protein (CRP) concentrations as an inflammatory marker. CRP has been used as an inflammatory marker in assessing inflammation in MS patients(Reference Giovannoni, Miller and Losseff19, Reference Sellner, Greeve and Mattle20).
Materials and methods
Ethical approval
Ethical approval for the study was obtained from the Health Sciences Research Ethics Committee of the Cape Peninsula University of Technology. MS patients were contacted and recruited through the MS Society, Western Cape Branch, South Africa.
Study population
The study population consisted of twenty-six female Caucasians and twenty-five age- and sex-matched control subjects. The median age of the MS patients was 51 (range 42–62) years and that of the controls was 52 (range 40–60) years. The time since diagnosis was 9 (range 6–17) years, and the median EDSS of the MS patients was 5·25 (range 4·50–7·50). Five of the patients were active disease cases whilst twenty-one were in the remission phase. Of the patients in remission, eleven had had relapses 5–12 months previously, while ten patients had had no relapses during the previous year. The sample size was based on the prevalence rate given for rare diseases, which is 1 %(Reference Naing, Winn and Rusli21). The patients recruited were diagnosed by a neurologist based on clinical, laboratory and MRI findings. The exclusion criteria used in the present study included the use of fatty acid supplements, interferon and cortisone or presence of a second disease for both relapsing–remitting MS (RRMS) patients and control subjects.
Measurement of the disability status of patients
The functional disability status (disease severity) of each patient was measured by a trained clinician using the Kurtzke EDSS(Reference Kurtzke18). The EDSS quantifies disability in eight functional systems and allows neurologists to assign an FSS in each of them. The functional systems are pyramidal, cerebellar, brainstem, sensory, bowel and bladder, visual, cerebral and ‘other’. Higher values indicate greater disability. Scales for the total Kurtzke EDSS are from 0 to 10, in which the 0 score indicates no disability at all and 10 indicates death due to MS. The clinician was blinded to the results of the fatty acid analysis (i.e. the clinical evaluation was not influenced by prior knowledge of the fatty acid values).
Blood sample analysis
Venous blood (15 ml) from both the patients and control subjects was collected into anti-coagulant EDTA tubes (Beckman Coulter South Africa (Pty) Ltd, Johannesburg, South Africa) and immediately separated using histopaque-1077 separation medium according to the manufacturer's instructions (Sigma-Aldrich (Pty) Ltd, Aston Manor, South Africa). The PBMC were washed in a 0·85 % saline solution and stored in 1 ml saline at − 80°C. A 0·85 % saline solution was used in the present study instead of the prescribed balanced PBS solution, as phosphate was inappropriate for additional tests done in the study. For all participants an extra 5 ml EDTA blood was collected for full blood count analysis. Full blood counts were determined on a Beckman Coulter LH 750 haematology analyser: lymphocytes were 25·9 % (6·89 (5·83–8·48) × 109); monocytes were 5·8 % (1·52 (1·05–2·00) × 109).
The PBMC membrane fatty acid composition of PC, PE, phosphatidylserine (PS), phosphatidylinositol (PI) and SM phospholipids of RRMS patients and control subjects were measured by GC. PBMC (800 μl) were extracted with chloroform–methanol (18 ml; 2:1, v/v) according to a modified method of Folch et al. (Reference Folch, Lees and Sloane Stanley22). All the extraction solvents contained 0·01 % butylated hydroxytoluene as an antioxidant. NEFA were separated from the total phospholipid fraction by TLC on pre-coated silica gel sixty plates (10 × 10 cm) without a fluorescent indicator (no. 1.05 721; Merck, Darmstadt, Germany) using the solvent system petroleum benzine (boiling point 40–60°C)–diethyl ether (peroxide free)–acetic acid (90:30:1, by vol.) as previously described(Reference Van Jaarsveld, Smuts and Tichelaar23). Individual phospholipid classes were separated by TLC on pre-coated silica gel 60 plates (10 × 10 cm) without a fluorescent indicator (Merck) using chloroform–petroleum benzene–methanol–acetic acid–boric acid (40:30:20:10:1·8, by vol.) as solvent(Reference Gilfillan, Chu and Smart24). The lipid bands containing PC, PE, PS, PI and SM were visualised with long-wave UV light after spraying the plates with chloroform–methanol (1:1, v/v) containing 2,5-bis-(5′-tert-butylbenzoxazolyl-[2′])thiophene (10 mg/100 ml; Sigma-Aldrich). The lipids were transmethylated using 5 % H2SO4–methanol at 70°C for 2 h (SM for 18 h). After cooling, the resulting fatty acid methyl esters (FAME) were extracted with 1 ml water and 2 ml n-hexane. The top hexane layer was evaporated to dryness, redissolved in CS2 and analysed by GLC (Finnigan Focus GC, equipped with flame ionisation detection; Thermo Electron Corporation, Austin, TX, USA) using 30 m BPX 70 capillary columns of 0·32 mm internal diameter (SGE International Pty Ltd, Ringwood, Victoria, Australia). Gas flow rates were: N2 (make up gas), 25 ml/min; air, 250 ml/min; H2 (carrier gas), 25 ml/min, with a split ratio of 20:1. Temperature programming was linear at 5°C/min, initial temperature 140°C, final temperature 220°C, injector temperature 220°C, and detector temperature 250°C. The FAME were identified by comparison of the retention times with those of a standard FAME mixture (Nu-Chek Prep Inc., Elysian, MN, USA). The individual FAME were quantified against an internal standard (C17: 0; Sigma-Aldrich).
CRP concentrations were determined in a routine chemical pathology laboratory on a Beckman nephelometer autoanalyser using reagents from Beckman (Beckman Coulter South Africa (Pty) Ltd, Johannesburg, South Africa). The diagnostic values of this laboratory are considered positive for CRP values greater than or equal to 5 μg/ml.
Statistical analysis
A statistics programme (STATISTICA 7; StatSoft Inc., 1984–2004, Tulsa, OK, USA) was used to perform all statistical analyses. The PBMC membrane PC, PE, PS, SM and PI phospholipid fatty acid profiles are reported in μg fatty acids/mg protein. Descriptive data are presented as median and range. For asymmetrical data the Mann–Whitney U test was used to compare distributions between the cases and control subjects. Correlations between fatty acids, CRP and EDSS FSS were calculated using Spearman's rank correlation coefficient. Results were considered significant if P values were less than 0·05.
Results
Differences in the fatty acid profile between relapsing–remitting multiple sclerosis patients and controls
Significant differences were observed between the RRMS and control groups in their fatty acid profile. PE, PS 22 : 4n-6, as well as total 22 : 4n-6, were lower in RRMS patients than in the control subjects (P = 0·01, P = 0·03 and P = 0·02 respectively) (Table 1).
Table 1 Differences in the peripheral blood mononuclear cell membrane fatty acids between relapsing–remitting multiple sclerosis (RRMS) patients and control subjects
(Medians and ranges)

PC, phosphatidylcholine; PE, phosphatidylethanolamine; PS, phosphatidylserine; PI, phosphatidylinositol; SM, sphingomyelin.
C-reactive protein concentrations
No significant differences were observed in the CRP concentrations between the RRMS patients and the control subjects; however, significant inverse correlations were observed between the RRMS membrane fatty acid values and the CRP concentrations but not in the control group (Table 2). In RRMS patients with CRP values above 5 μg/ml, PC 20 : 4n-6 demonstrated a decreasing trend whilst the opposite was observed in controls (Fig. 1). There was a significant positive correlation in the RRMS patients between the CRP concentrations and the EDSS (R 0·45; P = 0·03) (Fig. 2).
Table 2 Correlations between C-reactive protein values and the peripheral blood mononuclear cell membrane fatty acids in controls and relapsing–remitting multiple sclerosis (RRMS) patients
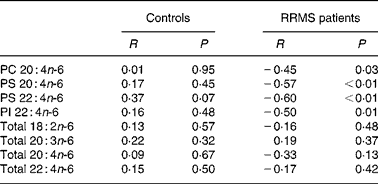
PC, phosphatidylcholine; PS, phosphatidylserine; PI, phosphatidylinositol.

Fig. 1 C-reactive protein (CRP) () and phosphatidylcholine (PC) 20 : 4n-6 (
) levels. In relapsing–remitting multiple sclerosis (RRMS) patients with CRP values above 5 μg/ml, PC 20 : 4n-6 demonstrated a non-significant decreasing trend whilst the opposite was observed in controls. FA, fatty acids.
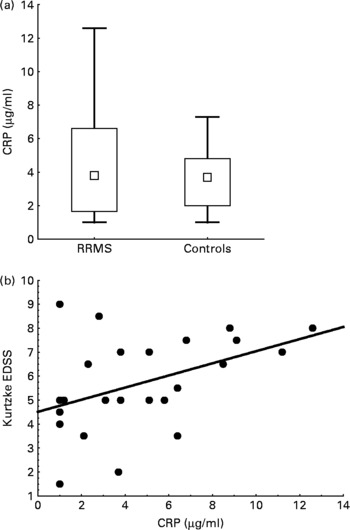
Fig. 2 C-reactive protein (CRP) levels and the Expanded Disability Status Scale (EDSS)(Reference Kurtzke18). (a) CRP levels in relapsing–remitting multiple sclerosis (RRMS) patients and control subjects. Values are medians, 25th–75th percentiles and ranges. The median for RRMS patients was 3·80 (25th–75th percentiles 1·65–6·60) μg/ml; for controls it was 3·70 (25th–75th percentiles 2·00–4·80) μg/ml (P = 0·35). (b) Correlation between CRP levels and the EDSS in RRMS patients. The correlation was significantly positive (R 0·45; P = 0·03).
Metabolic relationship between the fatty acids in the n-6 fatty acid series in relapsing–remitting multiple sclerosis patients and control subjects
The metabolic relationships between the n-6 fatty acids are summarised in Table 3. In both the RRMS and control subjects, 18 : 2n-6 demonstrated in general a positive correlation with 20 : 3n-6, 20 : 4n-6 and 22 : 4n-6. A positive correlation was also observed between 20 : 3n-6 and 20 : 4n-6, as well as between 20 : 4n-6 and 22 : 4n-6. However, total 18 : 2n-6 and 20 : 3n-6 were positively correlated in the RRMS patients only.
Table 3 Correlations between the fatty acids within the n-6 fatty acid series in controls and relapsing–remitting multiple sclerosis (RRMS) patients

PC, phosphatidylcholine; PE, phosphatidylethanolamine; PS, phosphatidylserine; PI, phosphatidylinositol; SM, sphingomyelin.
Correlation between the n-6 and n-3 fatty acids and the Kurtzke Expanded Disability Status Scale and Functional System Scores in relapsing–remitting multiple sclerosis patients
Table 4 summarises the significant correlations between PBMC membrane fatty acids and the Kurtzke EDSS and FSS. PC, PS and PI 18 : 2n-6 showed positive correlations with the pyramidal, brainstem and bowel/bladder FSS respectively. PC and PS 20 : 3n-6 showed positive correlations with the EDSS and cerebral FSS respectively. Total 20 : 3n-6 also showed a positive correlation with the EDSS. Both PE and PI 20 : 4n-6 showed inverse correlations with the sensory FSS and PC 22 : 4n-6 showed an inverse correlation with the visual FSS. Total 20 : 4n-6 also showed an inverse correlation with the sensory FSS. In the n-3 fatty acid series, both PC 20 : 5n-3 (EPA) and PC 22 : 6n-3 (DHA), showed positive correlations with the brainstem FSS.
Table 4 Correlations between membrane fatty acids and the Expanded Disability Status Scale (EDSS) and Functional System Score (FSS) in relapsing–remitting multiple sclerosis patients

PC, phosphatidylcholine; PS, phosphatidylserine; PI, phosphatidylinositol; PE, phosphatidylethanolamine.
Discussion
Fatty acids, particularly 18 : 2n-6 and 20 : 4n-6, have been shown to be reduced in the plasma, platelets, erythrocytes, leucocytes and cerebrospinal fluid of RRMS patients, but the reports are inconsistent(Reference Harbige and Sharief2). Cultural and ethnic differences, as well as dietary variability, especially in a diseased state, have been implicated in the differences observed in these studies. In the present study, the elongation product of 20 : 4n-6, 22 : 4n-6, in PBMC membrane PE and PS phospholipids was significantly decreased in the RRMS patients studied. In addition, 18 : 2n-6 and 20 : 3n-6 showed positive correlations with the EDSS. It is unlikely that the reduced 22 : 4n-6 levels were due to dietary requirements as 18 : 2n-6, the parent dietary fatty acid, was similar in both RRMS and control subjects. The n-6 PUFA are precursors for a number of mediators of inflammation that are released from membrane phospholipids in the course of inflammatory activation(Reference Bagga, Wang and Farias-Eisner25, Reference Simopoulos26). It is possible that lower n-6 PUFA in MS could be due to an increased eicosanoid production that regulates a great number of inflammatory effects, which depends on each precursor(Reference De Pablo and De Cienfuegos27). This in turn could affect the total fatty acids pool, hence neural requirements, since the human brain requires four times the amount of 20 : 4n-6 than 22 : 6n-3 on a daily basis(Reference Harbige and Sharief2, Reference Horrobin, Peet, Glen and Horrobin28, Reference Rappaport and Dodge29). Similar to Harbige & Sharief(Reference Harbige and Sharief2), we have shown a disturbed relationship between 20 : 3n-6 and 20 : 4n-6 in the PC fraction in MS. Furthermore, we have found a reversal of the 20 : 3n-6 and 20 : 4n-6 relationship in the PC and PE fractions of MS PBMC membranes compared with controls. Since PE is the second most abundant phospholipid in animal cell membranes, the positive correlation between 20 : 3n-6 and 20 : 4n-6 in the PE fraction may be compensating for the negative correlation between 20 : 3n-6 and 20 : 4n-6 in the PC fraction.
CRP has been used as an inflammatory marker in assessing inflammation in RRMS patients(Reference Giovannoni, Miller and Losseff19, Reference Sellner, Greeve and Mattle20). Several studies have shown inverse correlation with CRP and PUFA, but inconsistencies exist in specific fatty acids(Reference Yoneyama, Miura and Sasaki30–Reference Petersson, Lind and Hulthe33). Petersson et al. (Reference Petersson, Lind and Hulthe33) demonstrated inverse (18 : 2n-6) and positive (20 : 3n-6) association with CRP. Similarly, significant inverse correlations were observed between the CRP and membrane PC and PS 20 : 4n-6, as well as with PS and PI 22 : 4n-6 in RRMS cases. In contrast, in control subjects with CRP levels above 5 μg/ml, PC 20 : 4n-6 demonstrated an increasing trend whilst in RRMS patients the opposite was observed. These results suggest that the degree of inflammation is an important determinant of resultant fatty acid concentrations. In addition, we observed a positive correlation between CRP and disease severity as measured by the EDSS. It has been reported that serum levels of many inflammatory markers do not correlate with short-term disease progression(Reference Giovannoni, Miller and Losseff19). Giovannoni et al. (Reference Giovannoni, Miller and Losseff19) found no correlation between inflammatory markers, including CRP, and the EDSS, or changes in any of the MRI measured in a group of RRMS patients they studied over an 18-month period. However, in an earlier study the same authors(Reference Giovannoni, Thorpe and Kidd34) demonstrated that raised CRP concentrations correlated with infectious episodes and clinical relapse. We think that the present results could possibly be explained by the fact that we excluded patients on disease-modifying agents, interferon and corticosteroids, which are potent anti-inflammatory agents.
The limitations of the present study were that only female patients were used and the small sample size. As we also did not consider dietary fatty acid intake, this could have affected the fatty acid levels observed in the study. The main strength of the present study is that neither the cases nor the controls were on any fatty acid supplements, and the patients were not on interferon or corticosteroid treatment. Since it is difficult to recruit patients with RRMS not on any of these medications or supplementations, this resulted in a small sample size. In conclusion, the present results concur with Harbige & Sharief(Reference Harbige and Sharief2) that the relationship between n-6 fatty acids 20 : 3n-6 and 20 : 4n-6 is disturbed in RRMS patients and affects the physiological integrity of immune cells. Furthermore we have shown the phospholipid fractions involved. It would, however, be of interest to investigate the role of desaturases and elongases in fatty acid metabolism in MS and other inflammatory conditions. The essential parent fatty acids, 18 : 2n-6 and 18 : 3n-3 (α-linolenic acid), cannot be synthesised in the body and must be obtained from the diet(Reference Zamaria35). Most of the effects of 18 : 2n-6 and 18 : 3n-3 are dependent not only on the ingested essential fatty acids themselves, but on their derivatives(Reference Horrobin, Manku and Horrobin36). The parent dietary essential fatty acids are converted to their metabolites (the n-6 and n-3 series respectively) by a series of alternating desaturations and elongations(Reference Horrobin, Manku and Horrobin36, Reference Sprecher37). The inverse correlations between fatty acids, CRP and disease severity and fatty acids suggest that the disease state may in part explain the reported inconsistencies in fatty acid levels in MS patients. Based on the present results, we suggest that further studies should take into account CRP levels in the interpretation of results.
Acknowledgements
We would like to extend our sincere gratitude to the following: MS Society, Western Cape Branch, South Africa and Sister Treska Botha for the recruitment of patients; Zakariya Mohammed for statistical analysis; Johanna van Wyk for technical support in the analysis of fatty acids; Dr Marius de Klerk for assisting with the measurement of the EDSS and FSS.
The present study was funded by a grant from the University Research Fund of the Cape Peninsula University of Technology.
G. H. designed the study and wrote the text. M. H. was a collaborator, was involved in the interpretation of the study and proofread the manuscript. S. J. v R. was a co-supervisor, was involved in the recruitment of volunteers for the study and proofread the manuscript. S. A. was a co-supervisor, participated in fatty acid analysis, interpretation of the study and proofread the manuscript. D. W. M. was involved in fatty acid analysis and interpretation of the study, and proofread the manuscript. P. v J. participated in fatty acid analysis and interpretation of the study, and proofread the manuscript. C. S. acted as a collaborator and proofread the manuscript. F. H. measured neurological outcome and proofread the manuscript. R. E. was a collaborator and proofread the manuscript. T. M. was the supervisor and coordinator of this group and was involved in the recruitment of volunteers for the study, and proofread the manuscript.
There are no conflicts of interest to declare.