Homocysteine (Hcy), a sulphur-containing amino acid, is produced as an important intermediary of methionine metabolism( Reference Messedi, Frigui and Chaabouni 1 ). The fasting plasma Hcy levels in healthy adults are 5–15 μmol/l, and higher than 15 μmol/l is considered to be hyperhomocysteinaemia (HHcy). In recent years, many studies have supported the association of HHcy with an increased risk of CVD( Reference Refsum, Ueland and Nygard 2 ). HHcy has also been linked to increased risks of neural tube defects( Reference Steegers-Theunissen, Boers and Trijbels 3 ), Alzheimer’s disease( Reference Clarke, Smith and Jobst 4 ), pregnancy complications( Reference Schaffer, Verdoia and Cassetti 5 , Reference Yajnik, Chandak and Joglekar 6 ) and inflammatory bowel disease( Reference Mahmud, Molloy and McPartlin 7 ).
Factors affecting plasma Hcy level include age, sex, smoking, drinking, nutritional factors, liver and kidney function and folate/Hcy metabolism-related gene polymorphisms, and both genetics and folic acid intake are important( Reference Gonzalez-Gross, Benser and Breidenassel 8 – Reference Taguchi, Mori and Hamada 12 ). In the past two decades, many studies have been conducted to explore the decrease in plasma Hcy levels after folate supplementation( Reference den Heijer, Brouwer and Bos 13 – Reference Zappacosta, Mastroiacovo and Persichilli 15 ) However, these studies only report the reduction in plasma Hcy levels after long-term folate supplementation and do not report whether plasma Hcy was reduced to a normal level (5–15 μmol/l).
There is no report on the relationship between gene polymorphism and the efficacy of folate therapy in patients with HHcy. In this study, we gave folate supplements to patients with HHcy at a sufficient dose and reported the efficacy of folate therapy to reduce plasma Hcy levels to normal. The subjects were divided into the failure group and the success group according to the plasma Hcy level after the intervention. The relationships between environmental factors and folate metabolism-related gene polymorphisms and the efficacy of folate on HHcy were explored. This study provides a scientific basis for more effective prevention and treatment of HHcy.
Methods
Study design and participants
We performed a prospective cohort study of HHcy patients (Hcy≥15 μmol/l) who had plasma Hcy levels measured in the Department of Neurology in the Fifth Affiliated Hospital of Zhengzhou University from July to December 2014. The subjects included in our study met the following criteria: (1) tested for the plasma Hcy level, (2) diagnosed with HHcy (total plasma Hcy ≥15 μmol/l), (3) >18 years of age and (4) voluntarily participated in this study and received 90 d of folic acid supplementation. Subjects with a history of serious infection, hepatic or kidney diseases, haematologic disorders or cancer and those who used vitamin B or folic acid supplements or use medications that interfere with folate metabolism (methotrexate, phenytoin, etc.) in the prior 2 weeks were excluded. Thus, a total of 858 subjects were ultimately included in this study. Fasting plasma Hcy levels were measured at day 1. Then, the enrolled patients were treated with oral folic acid (5 mg/d) for 90 d. The compliance with oral folic acid was assessed by telephone interview at 45 and 90 d of follow-up. Plasma Hcy levels were obtained at the second follow-up.
The study was approved by the Ethics Review Committee of the Life Science of Zhengzhou University. All subjects or relatives signed informed consent.
Efficacy criteria and grouping standard
The therapy was effective if patients’ Hcy levels decreased to 15 μmol/l or less, which put them in the success group. The therapy was unsuccessful if patients’ Hcy levels were ≥15 μmol/l, which put them in the failure group.
Patients were also grouped according to their baseline plasma Hcy level. Baseline Hcy levels with elevations of 16–30, 31–100 and>100 μmol/l were classified as mild, moderate and severe HHcy, respectively( Reference Kaul, Zadeh and Shah 16 ).
Blood collection and laboratory techniques
At baseline, overnight-fasting blood samples were drawn for the clinical chemistry tests and for the measurements of plasma Hcy, which were also measured at the end of study period. The remaining samples were immediately placed on ice, transported to the molecular biology laboratory and centrifuged at 12 000 rpm for 5 min. Plasma and cells were separated and stored at −80°C until analysis. Genomic DNA was extracted using a whole-blood genomic DNA extraction kit (BioTeke®). DNA extraction was performed according to the manufacturer’s instructions.
Sample size
According to the relevant references, and with the effects of folic acid supplementation and folate-related enzyme gene polymorphisms on plasma Hcy level taken into consideration, a nonparametric matching design was adopted. The SNP with the lowest variation rate of genetic loci in the population was selected to estimate the sample size. Minor allele frequency (MAF) P 0 =13 %, expected risk ratio (RR)=2·0, α=0·05 and β=0·10. The formula is:

The sample size was approximately 300, with 150 in each group.
DNA quantification and purity determination
The purity of the extracted DNA solution was measured by an ultramicroscopic UV-V is spectrophotometer. The ratios of A260:A280 and A260:A230 were determined at pH 7–8·5. The purity of DNA was determined by the ratio of A260:A280; a ratio between 1·8 and 2·0 indicated a higher DNA purity, and a ratio <1·8 indicated contamination by protein or phenolic substances.
SNP selection and genotyping
HaploView4.2 software was used to download the information on MTRR, MTR, MTHFR and MTHFD SNP in the Chinese Han population from HapMap. Selection criteria were as follows: MAF>0·05; linkage disequilibrium value r 2 >0·8; and functional SNP or SNP that induces a change in protein activity.
We selected three SNP in MTRR: rs1801394, rs162036 and rs1532268; three SNP in MTR: rs1805087, rs1266164 and rs12354209; three SNP in MTHFR: rs1801133, rs1801131 and rs2274976; and two SNP in MTHFD: rs2236225 and rs1950902. Genotypes and alleles were detected using Sequenom’s MassArray system.
Statistical analysis
Statistical analyses were conducted using the SPSS software package (version 21.0 for windows). Patients who were lost to follow-up or had poor compliance were excluded. The numerical data were expressed as median values with interquartile ranges (25th–75th percentiles). Changes in Hcy level from pre-treatment to post-treatment were compared by Student’s t test. The relative decrease in Hcy levels is expressed as (Hcy after treatment – Hcy at baseline)/(Hcy at baseline). The difference in the efficacy rate was assessed by the χ 2 test. The significance of any differences between the two groups was examined with Student’s t test or the χ 2 test.
The relationship between SNP and the efficacy of folic acid therapy for HHcy were examined using unconditioned logistic regression models with and without adjustment for smoking, drinking, history of diabetes, hypertension, CHD and biochemical indicators. The IHG webpage’s online detection method was used to analyse Hardy–Weinberg equilibrium. SHEsis online software was used to estimate haplotypes. Multi-factor dimensionality reduction (MDR) software was used to evaluate gene–gene and gene–environment interactions. We considered a two-tailed P value<0·05 as significant. The statistical power was calculated by the standard formula.
Results
Efficacy of folic acid treatment for hyperhomocysteinaemia
A total of 1033 patients with HHcy were enrolled at the baseline, and 175 patients who were lost to follow-up or had poor compliance were excluded. A total of 858 were ultimately included in this study. The average age of the patients was 64·74 (sd 13·34) years. After treatment with folic acid for 90 d, the plasma Hcy levels of 484 patients decreased to 15·0 μmol/l or less, corresponding to an efficacy rate of 56·41 %. Before treatment, the median value of the plasma Hcy levels was 22·10 (sd 8·55) μmol/l. After treatment, the value was 15·90 (sd 5·74) μmol/l. The relative decrease in plasma Hcy levels was 28·05 % overall. Plasma Hcy levels after treatment were significantly lower than those before treatment (t=28·41, P<0·05).
Table 1 shows the distribution of demographic features and plasma Hcy baseline levels. Table 2 shows the general characteristics of the success group and failure group. Furthermore, baseline Hcy level had an effect on the efficacy of folate therapy in patients with HHcy (Table 3).
Table 1 Distribution of demographic features and plasma homocysteine (Hcy) baseline levels (Numbers and percentages; mean values and standard deviations)
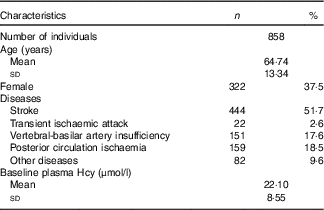
Table 2 General characteristics of success group and failure group (Mean values and standard deviations)

Hcy, homocysteine; FPG, fasting plasma glucose; TC, total cholesterol.
* t Value, not χ 2.
Table 3 Effect of the baseline homocysteine (Hcy) level on the efficacy of folate treatment for hyperhomocysteinaemia (HHcy)

Association of MTHFR gene polymorphism with the efficacy of folate
The frequency distributions of the genotypes and alleles of the MTHFR rs1801133, rs1801131 and rs2274976 SNP in the failure group and the success group are shown in Table 4. The frequency distributions of rs1801133 and rs1801131 genotypes and alleles were significantly different between the failure group and the success group after adjustment for age, sex, smoking, drinking and biochemical parameters by the binary logistic regression analysis (P<0·05). There was no significant difference in rs2274976 genotype or allele frequency between the failure group and success group, which indicates that rs1801131 and rs1801133 were associated with the effects of folic acid on HHcy, and rs2274976 was not.
Table 4 Association of MTHFR gene polymorphism with the efficacy of folate treatment for hyperhomocysteinaemia (Numbers and percentages; odds ratios and 95 % confidence intervals)

Ref., referent values.
The TT genotype and CT+TT genotypes of the rs1801133 SNP made up 47·29 and 89·14 % of the failure group, respectively, which were significantly higher than those in the success group (33·85 and 77·85 %) (P<0·05). Compared with the CC genotype, the risk of treatment failure was 2·68 times (P<0·000, OR=2·68; 95 % CI=1·59, 4·54) and 2·12 times (P=0·003, OR=2·12; 95 % CI 1·3, 3·44) as high in individuals carrying the TT genotype and CT+TT genotypes, respectively. The frequency of the T allele in the failure group was 68·21 %, which was significantly higher than the 55·85 % in the success group (P<0·05). Taking the C allele as a control, the risk of treatment failure was 1·69 times (P<0·000, OR=1·69; 95 % CI 1·35, 2·13) as high in individuals carrying the T allele.
The genotype and allele of rs1801131 were significantly different between the failure group and the success group (P<0·05). Compared with the AA genotype, the AC genotype and the CC genotype could decrease the risk of failure of folic acid therapy for HHcy (OR=0·52; 95 % CI 0·33, 0·81) and 0·26 (95 % CI 0·07, 0·91), respectively). Individuals with the C allele favoured folic acid therapy for HHcy (OR=0·58; 95 % CI 0·41, 0·81), with the A allele as control.
Association of MTHFD gene polymorphism with the efficacy of folate
The frequency distributions of genotypes and alleles of the MTHFD rs2236225 and rs1950902 SNP in the failure group and the success group are shown in Table 5. By unconditioned logistic regression analysis, we did not find that the frequency distribution of genotypes and alleles of rs2236225 were significantly different between the failure group and the success group and only found that the frequency distribution of the CT+TT genotypes of rs1950902 was statistically significantly different between the failure group and the success group. These findings suggest that rs2236225 has nothing to do with the efficacy of folic acid treatment on HHcy and that rs1950902 is weakly correlated with the efficacy of folic acid treatment on HHcy.
Table 5 Association of MTHFD gene polymorphism with the efficacy of folate treatment for hyperhomocysteinaemia (Numbers and percentages; odds ratios and 95 % confidence intervals)

Ref., referent values.
Association of MTR gene polymorphism with the efficacy of folate
The frequency distribution of the genotypes and alleles of the MTR SNP rs1805087, rs1266164 and rs12354209 in the failure group and the success group are shown in Table 6. By unconditioned logistic regression analysis, after adjustment for age, sex, smoking, drinking, personal disease history, biochemical indexes and other factors, the genotypes and alleles of rs1805087, rs1266164 and rs12354209 in the failure group and the success group were not statistically significantly different, which suggested that rs1805087, rs1266164 and rs12354209 had no effect on HHcy treatment with folic acid.
Table 6 Association of MTR gene polymorphism with the efficacy of folate treatment for hyperhomocysteinaemia (Numbers and percentages; odds ratios and 95 % confidence intervals)

Ref., referent values.
Association of MTRR gene polymorphism with the efficacy of folate
The frequency distribution of genotypes and alleles of the MTRR SNP rs1801394, rs162036 and rs1532268 in the failure group and the success group are shown in Table 7. By unconditioned logistic regression analysis, after adjustment for age, sex, smoking, drinking, personal disease history, biochemical indexes and other factors, there were significant differences in the genotypes and alleles of rs1801394 and the rate of the AG+GG genotypes of rs162036 between the failure group and the success group, but there was no significant difference in the genotypes and alleles of rs1532268, which suggested that rs1801394 and rs162036 are associated with the efficacy of folic acid treatment for HHcy, but rs1532268 is not.
Table 7 Association of MTRR gene polymorphism with the efficacy of folate treatment for hyperhomocysteinaemia (Numbers and percentages; odds ratios and 95 % confidence intervals)

Ref., referent values.
The frequency distribution of the AG, GG and AG+GG genotypes of rs1801394 in the failure group were 55·59, 8·95 and 64·54 %, respectively, which were significantly higher than those in the success group (36·92, 5·85 and 42·77 %) (P<0·05). Compared with the AA genotype, the risk of folic acid treatment of HHcy failure in individuals carrying the AG genotype, GG genotype and AG+GG genotypes was 2·66 times (P<0·000, OR=2·66; 95 % CI 1·83, 3·85), 2·88 times (P=0·003, OR=2·88; 95 % CI 1·44, 5·76) and 2·68 times (P<0·000, OR=2·68; 95 % CI 1·87, 3·85) as high as that for individuals carrying the CC genotype. The frequency of the G allele in the failure group was 36·74 %, which was significantly higher than that of the success group (24·31 %, P<0·05). The risk of treatment failure in individuals carrying the G allele was 1·81 times as great as in individuals carrying the A allele (P=0·000, OR=1·81; 95 % CI 1·42, 2·30).
The frequencies of the AG genotype and AG+GG genotypes of rs162036 in the failure group were 19·49 and 24·28 %, respectively, which were significantly lower than those in the success group (30·15 and 33·23 %, P<0·05). Compared with the AA genotype, the individuals with the AG genotype and the AG+GG genotypes favoured folic acid therapy for HHcy (OR=0·56; 95 % CI 0·37, 0·83 and OR=0·63; 95 % CI 0·43, 0·92, respectively). Individuals with the G allele were favourably treated with folic acid, but there was no significant difference (P>0·05) from the A allele as control.
Association of MTHFR and MTRR gene haplotypes with the efficacy of folate
The linkage disequilibrium parameters (r 2) of MTHFR rs1801133 and rs1801131 and MTRR rs1801394 and rs162036 were 0·96 and 0·75, respectively, which suggested that there was a linkage disequilibrium between the two.
The haplotypes of MTHFR rs1801133-rs1801131 and MTRR rs1801394-rs162036 were analysed. The frequency distribution of each haplotype in the success and failure groups are shown in Tables 8 and 9. The haplotype analysis showed that rs1801133 and rs1801131 produced four haplotypes. The frequencies of the CA and CC haplotypes in the failure group were lower than those in the success group (P<0·05). These results show an association with risk of folic acid treatment failure. The frequency of the TA haplotype was significantly higher in the failure group than in the success group (P<0·05), which suggested that the TA haplotype could increase the risk of failure of folic acid therapy for HHcy.
Table 8 Association of haplotypes of MTHFR rs1801133-rs1801131 with the efficacy of folate treatment for hyperhomocysteinaemia (Numbers and percentages; odds ratios and 95 % confidence intervals)

Table 9 Association of haplotypes of MTRR rs1801394-rs162036 with the efficacy of folate treatment for hyperhomocysteinaemia (Odds ratios and 95 % confidence intervals)

rs1801394 and rs162036 also produced four haplotypes. The frequency of the AG haplotype in the failure group was lower than that in the success group (P<0·05), which suggested that the AG haplotype could reduce the risk of folic acid treatment failure for HHcy. The frequency of the GA haplotype in the failure group was significantly higher than that in the success group (P<0·05), which suggested that the GA haplotype could increase the risk of failure of folic acid treatment.
Gene–gene interaction
In this study, we analysed the interaction between genes and genes by using multi-factor dimensionality reduction (MDR) software. The polymorphisms of 11 SNP (rs1801133 (X1), rs1801131 (X2), rs2274976 (X3), rs2236225 (X4), rs1950902 (X5), rs1801394 (X6), rs162036 (X7), rs1532268 (X8), rs1805087 (X9), rs1266164 (X10) and rs12354209 (X11)) were introduced into the MDR software in the data format (txt format). The optimal model was obtained by software fitting with the interaction of first- to third-order different gene loci (Table 10).
Table 10 Gene–gene interaction (Odds ratios and 95 % confidence intervals)

* X1: rs1801133; X6: rs1801394; X8: rs1532268.
The optimal factor for the univariate model was rs1801394, which showed that the risk of treatment failure in the high-risk populations with rs1801394 was 2·44 times (OR=2·44; 95 % CI 1·77, 3·35) as high as in the low-risk populations. The optimal model of the two-factor interaction model was rs1801133 and rs1801394. The results showed that the risk of treatment failure in the high-risk populations with rs1801133 and rs1801394 was 2·65 times as high as in the low-risk group (OR=2·65; 95 % CI 1·92, 3·65). The optimal model of the three-factor interaction model was rs1801133, rs1801394 and rs1532268. The results showed that the risk of treatment failure in the high-risk populations with rs1801133, rs1801394 and rs1532268 was 3·03 times as high as that of the low-risk group (OR=3·03; 95 % CI 2·18. 4·21). According to the test set of the first- to third-order optimal model, the higher the value of the equilibrium accuracy and the higher the consistency of cross-validation, the better the model was. In addition, the two-factor interaction model was the best combination model. The effects of the combination of rs1801133 and rs1801394 on the efficacy of folic acid in the treatment of HHcy were more stable.
Gene–environment interaction
Next, we analysed the interaction between genes and environment with MDR. The results showed that smoking, diabetes, hypertension, CHD, total cholesterol (TC), HDL, LDL and other factors were significantly different between the failed group and the successful group (Table 2). With reference to the guideline of prevention and treatment of dyslipidaemia in Chinese adults, the TC, HDL-cholesterol and LDL-cholesterol converted into two categories of variables (TC≥5·18 mmol/l, HDL-cholesterol<1·04 mmol/l and LDL-cholesterol≥3·37 mmol/l assigned to 1). Then, smoking (X12), drinking (X13), diabetes mellitus (X14), history of hypertension (X15), history of CHD (X16), TC (X17), HDL (X18), LDL (X19) and the polymorphisms of eleven SNP were introduced into the MDR software in the data format (txt format). The optimal model was obtained by MDR software fitting with the interaction of first- to third-order different risk factors (Table 11).
Table 11 Gene–environment interaction (Odds ratios and 95 % confidence intervals)

* X1: rs1801133; X6: rs1801394; X15: history of hypertension; X16: history of CHD.
The optimal factor for the univariate model was CHD. The risk of failure of folic acid treatment with HHcy in patients with a history of CHD was 4·36 times (OR=4·36; 95 % CI 2·59, 4·68) that of patients without CHD. The optimal model of the two-factor interaction model was rs1801394 and history of CHD. The results showed that the risk of treatment failure in the high-risk population with rs1801394 and history of CHD was 4·75 times higher than that of the low-risk group (OR=4·75; 95 % CI 2·94, 7·08). The optimal model of the three-factor interaction model was rs1801133, history of CHD and history of hypertension. The results showed that the risk of treatment failure in the high-risk population with rs1801133, history of CHD and history of hypertension was 6·88 times higher than that of the low-risk group (OR=6·88; 95 % CI 3·98, 16·70). According to the optimal model of first to third order, the higher the value of the test set balance accuracy and the higher the consistency of cross-validation, the better the model was. The three-factor interaction model was the best combination model. That is to say, the effects of the combination of rs1801133, history of CHD and history of hypertension on the efficacy of folic acid in the treatment of HHcy were larger and more stable.
Discussion
HHcy is an independent risk factor for cardiovascular diseases, and oral folate can reduce plasma Hcy concentration. However, data on the Hcy-lowering effects of folic acid therapy are limited. In this study, we adopted a prospective method to observe the efficacy of folate therapy and found 484 of 858 HHcy patients (56·41 %) reached normal serum Hcy levels with folate treatment (5 mg/d) for 3 months. The efficiency of folate treatment of HHcy was low. Therefore, we combined the genetic factors and environmental factors to explore the causes of treatment failure.
We found that the CT genotype, TT genotype and T allele of MTHFR rs1801133 increased the risk of folate therapy failure for HHcy. Some studies have shown that rs1801131 was independent of plasma Hcy level and had no significant effect on the effect of folic acid on Hcy level( Reference Yang, Botto and Gallagher 17 – Reference Ho, Eikelboom and Hankey 20 ). This might be related to the distribution of MTHFR in different races and different populations. We also found that the AC genotype, the CC genotype and the C allele of MTHFR rs1801131 decreased the risk of folic acid therapy failure for HHcy. In addition, the genotype frequency distribution of MTHFR rs2274976 was not significantly different between the success group and the failure group and might not be related to the effect of folic acid on HHcy. At present, the majority of studies have shown the associations between MTHFR rs2274976 and diseases such as cleft lip, cleft palate and neural tube defects( Reference Murthy, Gurramkonda and Lakkakula 21 – Reference deAquino, Hoshi and Bagordakis 23 ). Few studies have reported the effect of MTHFR rs2274976 on the efficacy of folic acid supplementation on Hcy level.
The results showed that MTHFD rs2236225 and MTHFD rs1950902 were not associated with the efficacy of the folic acid therapy on HHcy. In fact, no study has found that rs2236225 or rs1950902 was related to Hcy level. The frequencies of the GG genotype, the AG genotype and the AA genotype of rs2236225 in this healthy population in northern China were 57·98, 35·57 and 6·45 % respectively, and the frequency of the A allele was 24·23 %, which was consistent with the gene distribution of the failure group and successful group. However, another study found that rs2236225 had nothing to do with the pathogenesis of CHD( Reference Cheng, Zhu and Dao 24 ). The interaction between rs2236225 and Hcy may be involved in the occurrence of gastric cancer in the Chinese Han population( Reference Wang, Ke and Chen 25 ).
There was no relationship between the genotypes and alleles of MTR rs1805087 and the efficacy of folic acid on HHcy. Studies have shown that there are significantly different genotype distributions of MTR rs1805087 in different regions and different ethnic groups( Reference Binia, Contreras and Canizales-Quinteros 26 ). This might be the reason why the results were inconsistent. This study found that MTR rs1266164 and rs12354209 were not associated with the effect of folic acid on HHcy.
MTRR has a key role in the remethylation of Hcy to methionine. We found that the AG genotype, GG genotype and G allele of MTRR rs1801394 increased the risk of treatment failure. rs1801394 has been associated with abnormal plasma Hcy levels in the body( Reference Hankey and Eikelboom 27 ). It had been found that individuals with the G allele of rs1801394 have a higher risk of developing HHcy than individuals with the A allele by 2·1 times( Reference Laraqui, Allami and Carrie 28 ). The AG genotype and AG+GG genotype of rs162036 were associated with the efficacy (OR0·56; 95 % CI 0·37, 0·83 and OR=0·63; 95 % CI 0·43, 0·92, respectively). These results indicate that mutations of rs162036 could reduce the risk of treatment failure. A previous study found that there was no significant difference in Hcy level between the AA genotype, AG genotype and GG genotype of rs162036( Reference Liang, Zhou and Wang 29 ), a result that might be related to the different types of subjects included in this paper. In this study, rs1532268 was not associated with the efficacy of folate in the treatment of HHcy.
To further explore the association between MTHFR or MTRR gene polymorphism and the efficacy of folate in the treatment of HHcy, the haplotypes of MTHFR rs1801133-rs1801131 and MTRR rs1801394-rs162036 were analysed. Our results suggest that the TA haplotype of MTHFR rs1801133-rs1801131 and the GA haplotype of MTRR rs1801394-rs162036 could increase the risk of failure of folic acid therapy for HHcy. The CA haplotype and CC haplotype of MTHFR rs1801133-rs1801131 and the AG haplotype of MTRR rs1801394-rs162036 could decrease the risk of failure of folic acid therapy for HHcy. The CA haplotype of rs1801133-rs1801131 was favourable for the treatment of HHcy by folic acid. It can be seen that the effect of the rs1801131 A allele was reduced to a certain extent when patients carried the rs1801133C allele. Each polymorphism site is not independent of the occurrence and development of the disease; there is still some correlation and interaction between the various sites( Reference Bahlo, Stankovich and Speed 30 ). The construction of the haplotype is the embodiment of genetic association, which can reveal the correlation between multiple SNP and diseases( Reference Stumpf 31 ).
In this study, MDR software was used to analyse the gene–gene interaction and gene–environment interaction. Our results suggest that the effects of the combination of rs1801133 and rs1801394 on the efficacy of folic acid in the treatment of HHcy were the most stable. It is also true that gene–gene interactions play a role in the treatment effect of folic acid, and the risk of interaction is higher than the risk conferred by single genes. Hcy metabolism involves multiple factors and multiple metabolic pathways. The Hcy level is not only affected by the level of folate but is also related to lifestyle, nutritional factors and genetic factors( Reference Liu, Gao and Sun 32 ). For these reasons, the effect of folic acid therapy on HHcy may be affected by gene–gene and gene–environment interactions. Our results show that the risk of treatment failure in the high-risk populations with rs1801133, history of CHD and history of hypertension may be 6·88 times higher than that of the low-risk group (OR=6·88; 95 % CI 3·98, 16·70). In addition, the three-factor interaction model was the best combination model. Therefore, it is helpful to improve the efficacy of folate therapy by controlling blood pressure at the normal level and actively treating CHD. However, the results were only statistically significant. Further studies are needed to reveal how gene–environment interactions affect the therapeutic effect.
This study aimed to resolve some of the issues found in disease prevention, and it has theoretical and practical value. The high rate of failure of oral folic acid intervention in HHcy has been neglected, and there is a lack of research on the failure of oral folic acid intervention for HHcy. No studies have shown whether the plasma Hcy of patients with HHcy was reduced to normal after folate intervention. This prospective study was carried out to explore the reasons for the failure of folic acid intervention in combination with genetic and environmental factors. In addition, this study had strict quality control in the selection of subjects, data collection and laboratory testing. A questionnaire survey was used to collect the data of the subjects face to face, and all the investigators were trained to reduce the bias.
Nevertheless, there are still some limitations to this study. The plasma folate level of the study group was not detected, and some interactions between folate level and other factors could have influence its efficacy. We did not collect information about other vitamins (B6 and B12), that may be involved in the metabolic pathway affected by the intervention. In addition, Hcy metabolism involves multiple factors and multiple metabolic pathways, and we only analysed the associations between the gene polymorphisms of some key enzymes in the folate metabolism pathway and the efficacy of folic acid on HHcy, not the gene polymorphisms in other pathways. We also failed to explore the associations of gene–gene and gene–environment interactions in different pathways with the efficacy of folic acid on HHcy.
Conclusion
Folate supplementation can effectively decrease Hcy levels. However, almost half of HHcy patients failed to reach the normal range. MTHFR rs1801133 and rs1801131 and MTRR rs1801394 and rs162036 were associated with the efficacy of folic acid treatment on HHcy. MTHFR rs1801133-rs1801131 haplotype and MTRR rs1801394-rs162036 haplotype were associated with the efficacy of folic acid treatment on HHcy. There was a significant interaction between rs1801133 and rs1801394 in the efficacy of folate therapy for HHcy. An interaction between rs1801133, CHD and hypertension was also found. A combination of SNP may be more effective in predicting the risk of failure of folate to alleviate HHcy.
Acknowledgements
The authors thank all staff from the Department of Neurology, the Fifth Affiliated Hospital of Zhengzhou University, for their assistance and support.
Funding was provided by the Department of Science and Technology of Henan Province (no. 132102310431). The Department of Science and Technology of Henan Province had no role in the design, analysis or writing of this article.
The authors’ responsibilities were as follows: B. D. had full access to all the data in the study and takes responsibility for the integrity of the data and the accuracy of the data analysis; D. T. and L. W.: study concept and design; H. T. and M. G.: acquisition of data; C. Z., Q. H. and W. W.: analysis and interpretation of the data; W. Z.: study supervision. All authors read and approved the final manuscript.
The authors declare that there are no conflicts of interest.