In ruminants, it is well recognised that endogenous urea-N production often exceeds apparent digestible N intake, yet ruminants still maintain a positive N balance by recycling 40–80 % of this urea-N to the gastrointestinal tract (GIT)(Reference Lapierre and Lobley1). Urea-N that passes into the rumen can provide ruminally available N for the synthesis of microbial protein, which is a major contributor to the metabolisable protein that is available for intestinal digestion(Reference Lapierre and Lobley1). Recently, we have demonstrated that feeding linoleic acid-rich sunflower oil (SFO) to partially defaunate the rumen was associated with (1) an increase in the proportion of endogenous urea-N that was recycled to the GIT and (2) a tendency to increase the proportion of recycled urea-N that was utilised for anabolic purposes(Reference Kiran and Mutsvangwa2). Previously, the removal of protozoa (i.e. defaunation) has been reported to improve N utilisation, mainly by decreasing the ruminal NH3-N pool, coupled with a greater sequestration of NH3-N into bacterial protein(Reference Firkins, Yu and Morrison3), and by decreasing intra-ruminal N recycling(Reference Jouany4). Our previous work showed that the positive effects of defaunation on N utilisation may also result from an increase in urea-N recycling to the rumen(Reference Kiran and Mutsvangwa2). Because defaunation decreases the ruminal NH3-N level(Reference Jouany4), this could potentially increase transepithelial urea-N transfer as the ruminal NH3-N level is negatively correlated with urea-N transfer into the rumen(Reference Kennedy and Milligan5). In addition, transepithelial movement of urea-N by passive diffusion into the rumen is facilitated by bacterial urease activity, which maintains a favourable concentration gradient(Reference Rémond, Meschy and Boivin6). Cheng & Wallace(Reference Cheng and Wallace7) demonstrated that urease activity in ruminal contents was negatively correlated with the ruminal NH3-N level.
Although the present results showed that a higher proportion of endogenous urea-N production was recycled to the GIT in partially defaunated ruminants, N intake differed due to protozoal status in that study as a result of decreased DM intake(Reference Kiran and Mutsvangwa2). Because of the positive correlation between N intake and hepatic output of urea-N(Reference Marini, Klein and Sands8, Reference Wickersham, Titgemeyer and Cochran9), data on urea-N recycling kinetics could have been confounded due to differences in N intake. In addition, the use of recycled urea-N for anabolic purposes showed only a tendency to be higher in partially defaunated animals compared with faunated animals(Reference Kiran and Mutsvangwa2), suggesting that ruminal energy supply might have limited microbial use of recycled urea-N for protein synthesis. Increasing dietary amounts of ruminally fermentable carbohydrate (RFC)(Reference Huntington10) or shifting carbohydrate digestion from the small intestine to the rumen via steam-flaking compared with dry-rolling of sorghum grain(Reference Theurer, Huntington and Huber11) has been shown to increase urea-N transfer to the rumen, in addition to increasing N sequestration into microbial protein as energy supply from RFC is the major driver for microbial growth(Reference Russell12, Reference Koenig, Beauchemin and Rode13). Also, substantial decreases in ruminal protozoal populations have been reported in ruminants fed high-grain diets or extensively processed barley(Reference Koenig, Beauchemin and Rode13, Reference Eadie, Hyldgaard-Jensen and Mann14). Providing more RFC, therefore, could improve N utilisation by stimulating both urea-N recycling to the GIT and microbial protein production. In western Canada and parts of the northern USA, barley grain is the principal cereal grain that is included in ruminant diets, and it is commonly fed either in a dry-rolled or in a pelleted form. Pelleting decreases particle size, thus shifting the site of carbohydrate digestion from post-ruminal sites to the rumen, and previous in situ studies in our laboratory have clearly indicated a higher soluble starch fraction, a higher degradation rate of the degradable starch fraction and a higher effective starch degradability of pelleted barley (PB) when compared with dry-rolled barley (DRB)(Reference Kiran and Mutsvangwa15). Based on this previous study, it can be expected that ruminal starch digestibility would be higher for PB compared with DRB, which could potentially alter urea-N recycling to the GIT and microbial protein synthesis in the rumen.
The major objective of the present study was to delineate how interactions between feeding SFO to partially defaunate the rumen and altering dietary RFC may alter urea-N kinetics and N metabolism in growing lambs. We hypothesised that feeding SFO to partially defaunate the rumen would increase urea-N recycling to the GIT and the utilisation of recycled urea-N for anabolic purposes, and that these effects would be more pronounced if ruminal energy supply was increased by feeding PB compared with DRB.
Materials and methods
Lambs that were used in the present study were cared for and handled in accordance with the guidelines of the Canadian Council of Animal Care(16), and their use was approved by the University of Saskatchewan Animal Care Committee (UCACS protocol no. 20040048).
Animals and experimental design
A total of four Suffolk ram lambs (61·5 (se 4·0) kg initial body weight) that were fitted with ruminal cannulae were used. The experiment was run as a 4 × 4 Latin square design with 27 d periods and a 2 × 2 factorial arrangement of treatments. Experimental periods consisted of 21 d of dietary adaptation and 6 d of data collection. Lambs were housed in individual floor pens (during dietary adaptation) or in metabolism crates (during data collection).
Experimental treatments and feeding management
The treatment factors were (1) 0 ( − SFO) v. 6 % (+SFO) SFO (on a DM basis) as a defaunating agent and (2) DRB v. PB as the principal source of RFC. The ingredient and chemical compositions of the experimental diets containing DRB or PB are presented in Table 1. The experimental diets were formulated to be isonitrogenous at 16 g N/kg DM. The preparation of DRB and PB was as described by Kiran & Mutsvangwa(Reference Kiran and Mutsvangwa15). DRB or PB was fed as the principal sources of dietary RFC in order to manipulate ruminal energy availability for microbial growth. Diets were offered in the form of a meal for ad libitum intake twice daily in equal portions at 09.00 and 17.00 hours, ensuring that 10 % orts remained each day. Lambs had free access to water.
Table 1 Ingredient and chemical composition of the experimental diets

* Salt, Co and I mix: Co, 100 mg/kg; I, 150 mg/kg.
† Provided (per kg of concentrate DM): CuO, 3·3 mg; ZnO, 22 mg; MnO, 18·5 mg; vitamin A, 1495 IU (0·449 mg); vitamin D, 125·5 IU (0·0031 mg); vitamin E, 22 IU (20 mg); sodium selenite, 0·1 mg.
‡ Calculated according to the National Research Council(60) guidelines.
Partial defaunation and refaunation
Linoleic acid-rich SFO (Safeway™ Canada, Saskatoon, SK, Canada) was fed (60 g/kg DM) as an anti-protozoal agent. The protocol for ruminal defaunation was based on a previous study(Reference Ivan, Mir and Koenig17) and similar to Kiran & Mutsvangwa(Reference Kiran and Mutsvangwa2). The measured fatty acid composition of SFO was 6·24, 3·87, 26·15, 67·07 and 0·49 % of palmitic (16 : 0), stearic (18 : 0), oleic (18 : 1), linoleic (18 : 2n-6) and linolenic (18 : 3n-3) acids, respectively. For lambs receiving SFO, daily allotments were calculated based on the previous day's DM intake. To avoid cross-inoculation of +SFO lambs by − SFO lambs via direct physical contact, individual floor pens and metabolism crates housing +SFO lambs were spaced several metres away from those housing − SFO lambs. In addition, ruminal fluid sampling of +SFO lambs was conducted before − SFO lambs in order to minimise the risk of cross-inoculation. To enumerate protozoa, 200 ml of ruminal contents were sampled at 11.00 hours on day 1 to 3, 6, 9, 12, 15, 18 and 21–26. The processing of ruminal contents for protozoal counting was conducted as described by Kiran & Mutsvangwa(Reference Kiran and Mutsvangwa2), and protozoal enumeration and differentiation into different genera (i.e. Entodinium sp., holotrichs and cellulolytic protozoa) were conducted as described by Ogimoto & Imai(Reference Ogimoto and Imai18). Partially defaunated lambs were refaunated at the start of an experimental period as described previously(Reference Kiran and Mutsvangwa2).
Sample collection
Experimental lambs were moved from individual floor pens into individual metabolism crates on day 19 of each experimental period to allow acclimatisation before the initiation of data collection on day 22. During the 6 d data collection period, individual lamb feed intake was recorded daily. Samples of the experimental diets and orts were collected daily, stored at − 20°C and composited per lamb for each experimental period before chemical analysis. On day 21 of each experimental period, lambs were fitted with temporary vinyl catheters (0·86 mm internal diameter × 1·32 mm outer diameter; Scientific Commodities, Inc., Lake Havasu City, AZ, USA) in the right and left jugular veins to allow for simultaneous isotope infusion and blood sampling. Urea-N transfer to the GIT and whole-body N balance were determined between days 22 and 26 as described by Lobley et al. (Reference Lobley, Bremner and Zuur19). Briefly, background samples of urine and faeces were collected on day 21 to measure the natural abundance of 15N. Starting on day 22 of each experimental period, [15N15N]urea (99 atom % 15N; Cambridge Isotope Laboratories, Andover, MA, USA) prepared in 0·15 m-sterile saline was infused continuously into a jugular vein using a peristaltic pump for 96 h. Daily dosage rates of [15N15N]urea ranged from 324 to 582 mg/lamb depending on N intake, such that the predicted plateau enrichment of [15N15N]urea in urine was 0·15 mol % excess. Total faeces and urine were collected daily between days 22 and 26 before the 09.00 hours feeding to determine daily outputs. Collection, processing and sampling of faeces and urine for N, and analysis of faecal 15N enrichment, urinary enrichment of [15N15N]-, [14N15N]- and [14N14N]urea and urinary purine derivatives (PD) were as described previously(Reference Kiran and Mutsvangwa2).
On day 26, 200 ml of ruminal contents were sampled at 09.00, 11.00, 13.00, 15.00 and 17.00 hours and squeezed through two layers of cheesecloth. Ruminal pH was immediately determined and 10 ml aliquots of ruminal fluid were preserved as described previously(Reference Kiran and Mutsvangwa2) for NH3-N and volatile fatty acid (VFA) analysis. The remaining ruminal fluid was kept frozen at − 20°C for the isolation of ruminal microbes. At the same time points corresponding to ruminal fluid sampling, blood samples were drawn from a jugular vein catheter into vacutainers containing heparin, and plasma was obtained as described previously(Reference Kiran and Mutsvangwa2) for plasma urea-N (PUN) analysis. On day 27, ruminal epithelial tissue biopsies were taken by clipping at 2 h after the morning feeding as described previously(Reference Kiran and Mutsvangwa2).
Ruminal starch degradation in diets containing DRB and PB was determined using the in situ method as described by Yu et al. (Reference Yu, Christensen and McKinnon20). Briefly, approximately 7 g of each diet were weighed into nylon bags and incubated in the rumen of a dairy cow fed barley silage for 2, 4, 6, 8, 12, 24 and 48 h. The rumen incubation protocol, nylon bags, washing and drying procedures for nylon bags were as described by Yu et al. (Reference Yu, Christensen and McKinnon20). Dried samples of the diet and nylon bag residues were analysed later for starch.
Sample analyses
At the end of the trial, frozen samples of the experimental diets, orts and faeces were thawed overnight at room temperature and analysed for DM by drying in an oven at 60°C for 48 h (method 930.15)(21). Dried diets, orts and faeces were then ground through a 1 mm screen using a Christy–Norris mill (Christy and Norris Limited, Chelmsford, Essex, UK). Ground diets, orts and faecal samples were pooled per lamb and analysed for organic matter (OM) (method 942.05), N using the macro-Kjeldahl procedure (method 2001.11), total starch (method 996.11) using a commercial kit (Total Starch Assay Kit; Megazyme International Ireland Limited, Wicklow, Republic of Ireland), diethyl ether extract (method 2003.05)(21), and acid-detergent fibre and neutral-detergent fibre(Reference Van Soest, Robertson and Lewis22). Amylase and sodium sulphite were used for neutral-detergent fibre determination. Total N in pooled urine was determined using the macro-Kjeldahl procedure(21). Dried nylon bag residues were analysed for total starch (method 996.11) using a commercial kit. The fatty acid composition of SFO was determined using GC (Agilent 6890; Agilent Technologies, Mississauga, ON, Canada) as described by Soita et al. (Reference Soita, Fehr and Christensen23).
Preserved ruminal fluid samples were analysed for VFA by GC, as described by Erwin et al. (Reference Erwin, Marco and Emery24), and NH3-N using a phenol-hypochlorite assay(Reference Broderick and Kang25). Frozen ruminal fluid samples for microbial isolation were thawed at room temperature and centrifuged at 20 000 g at 4°C for 20 min, and the supernatant fraction was discarded. The microbial pellet was washed with double-distilled water and centrifuged at 20 000 g at 4°C for 20 min, and this process was repeated two more times(Reference Makkar and Becker26). The microbial pellet was then freeze-dried, ground with a mortar and pestle and analysed for total N and individual purines as described by Makkar & Becker(Reference Makkar and Becker26). Daily urine samples (2 ml) that were diluted with distilled water (8 ml) were pooled per lamb proportionally to daily urine output and analysed for allantoin, xanthine plus hypoxanthine(Reference Chen and Gomes27) and uric acid by a quantitative enzymatic colorimetric method using a commercial assay kit (Stanbio Uric Acid Liquicolor Kit, Procedure No. 1045; Stanbio Laboratories, Boerne, TX, USA). Total PD excretion/d was calculated as allantoin+uric acid+xanthine plus hypoxanthine. Microbial non-NH3-N (NAN) supply was calculated based on total PD excretion in urine(Reference Chen and Gomes27), using the determined purine N:microbial N ratios and body-weight measurements obtained on day 19. PUN and urinary urea-N were determined by the diacetyl monoxime method(Reference Marsh, Fingerhunt and Kirsch28) using a commercial kit (Stanbio Urea N Kit, Procedure No. 0580; Stanbio Laboratories).
To determine the proportions of [15N15N]-, [14N15N]- and [14N14N]urea in daily urine and background urine samples, urinary urea was isolated by applying urine containing 1·5 mg of urea-N through pre-packed cation exchange resin columns (AG-50W- × 8 Resin, 100–200 mesh, H+ form; Biorad, Richmond, CA, USA) as described by Archibeque et al. (Reference Archibeque, Burns and Huntington29). The proportions of [15N15N]-, [14N15N]- and [14N14N]urea in isolated urinary urea-N were analysed by isotope ratio-MS(Reference Lobley, Bremner and Zuur19) at the N-15 Analysis Laboratory, University of Illinois at Urbana-Champaign. Under the conditions of this assay, [14N14N]-, [14N15N]- and [15N15N]urea molecules should yield ions with mass/charge values of 28, 29 and 30, respectively. To account for non-monomolecular reactions, standards that were prepared from [15N15N]urea (99·8 atom % 15N) and [14N14N]urea (natural abundance urea; 0·364 atom % 15N) were also analysed, and the necessary corrections for [14N15N]urea that was produced by non-monomolecular reactions were then made(Reference Lobley, Bremner and Zuur19). Faecal samples were analysed for total 15N enrichment by combustion to N2 gas in an elemental analyser and continuous flow isotope ratio-MS(Reference Lobley, Bremner and Zuur19). For urea transporter-B (UT-B) gene expression analysis, processing of ruminal epithelial tissue, RNA extraction, complementary DNA generation and quantification of gene transcript abundance using quantitative PCR were conducted as described previously(Reference Kiran and Mutsvangwa2). Ovine-glyceraldehyde 3-phosphate dehydrogenase was used as an internal reference to UT-B mRNA expression. The results are presented as target gene transcript abundance normalised to glyceraldehyde 3-phosphate dehydrogenase transcript abundance. Expression of glyceraldehyde 3-phosphate dehydrogenase mRNA in equal amounts of total RNA indicated that glyceraldehyde 3-phosphate dehydrogenase in rumen epithelial tissue was stable and unaffected by the experimental conditions that were employed.
Calculation of urea-nitrogen kinetics and statistical analysis
Rumen degradation characteristics of starch were analysed using the non-linear regression (NLIN) procedure(30) using iterative least-squares regression (Gauss–Newton method) as described by Yu et al. (Reference Yu, Christensen and McKinnon20). Urea-N kinetics was calculated according to the model of Lobley et al. (Reference Lobley, Bremner and Zuur19), using urinary 15N enrichment of [15N15N]- and [14N15N]urea and total 15N excretion in faeces. All data were analysed using ANOVA as a 4 × 4 Latin square using the Proc Mixed procedure of SAS(30), with animal and period as the random effects. The model used included the following independent variables: animal, period, SFO ( − SFO v. +SFO), barley grain processing (DRB v. PB) and the SFO × barley grain processing interaction. When significant SFO × barley grain processing interactions were detected, treatment means were compared using the Tukey–Kramer multiple comparison test. Data on PUN, ruminal pH and ruminal concentrations of VFA and NH3-N were analysed accounting for repeated measures(Reference Wang and Goonewardene31). For protozoal counts, statistical analysis was performed on log-transformed data. Treatment differences were considered significant when P < 0·05 and tendencies are discussed when 0·05 < P < 0·10. Data are presented as means with their standard errors.
Results
In situ ruminal starch degradation kinetics
In situ ruminal starch degradation kinetics is presented in Table 2. The in situ soluble and degradable starch fractions, the degradation rate of the degradable starch fraction and the effective starch degradability were greater (P ≤ 0·02) in the diet that contained PB when compared with that containing DRB.
Table 2 In situ ruminal degradation kinetics of starch in experimental diets containing dry-rolled barley or pelleted barley
(Mean values with their standard errors)

* Calculated assuming a ruminal passage rate of 6 %/h.
DM intake and total tract nutrient digestibilities
DM and OM intakes were unaffected (P ≥ 0·14) by treatment (Table 3). Total tract digestibilities of DM, OM, N, acid-detergent fibre and neutral-detergent fibre were unaffected (P ≥ 0·24) by feeding SFO and by the method of barley grain processing. Total tract digestibility of starch was greater (P = 0·001) in lambs fed PB compared with those fed DRB, but was unaffected by feeding SFO.
Table 3 Sunflower oil (SFO), basal DM, total organic matter (OM) intakes, and total tract nutrient digestibility in lambs fed dry-rolled or pelleted barley with (+SFO) or without (−SFO) SFO as a partial defaunating agent
(Mean values with their standard errors, n 4)
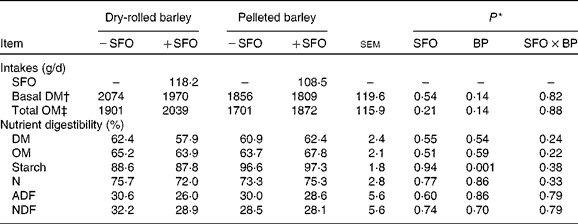
BP, barley grain processing; ADF, acid-detergent fibre; NDF, neutral-detergent fibre.
* SFO, addition of 6 % (DM basis) of linoleic acid-rich sunflower oil as a partial defaunating agent; BP, dry-rolled v. pelleted; SFO × BP, interaction.
† Basal DM intake does not include SFO intake.
‡ Total OM intake includes SFO intake.
Ruminal protozoal counts and fermentation characteristics
Treatment means for ruminal counts of protozoa during the measurement period (days 22–27) are presented in Table 4. Ruminal counts of total protozoa, Entodinium sp., holotrichs and cellulolytic protozoa were greater in − SFO lambs fed DRB compared with those fed PB (P < 0·01); consequently, there was a greater difference in ruminal counts of total protozoa, Entodinium sp., holotrichs and cellulolytic protozoa between − SFO and +SFO lambs fed DRB compared with those fed PB (interaction; P < 0·01). Experimental lambs were successfully refaunated by the administration of the ruminal fluid from naturally faunated sheep over 3 d, and mean populations (n 4) for total protozoa (10·6 (se 0·3) × 105/ml), Entodinium sp. (10·2 (se 0·3) × 105/ml), holotrichs (0·24 (se 0·02) × 105/ml) and cellulolytic protozoa (0·20 (se 0·02) × 105/ml) were restored to pre-defaunation levels in previously partially defaunated lambs by day 15 of the experimental period.
Table 4 Ruminal fermentation characteristics and protozoa number in lambs fed dry-rolled or pelleted barley with (+SFO) or without (−SFO) sunflower oil (SFO) as a partial defaunating agent
(Mean values with their standard errors, n 4)

BP, barley grain processing; ND, not detectable; VFA, volatile fatty acid.
a,b,c Mean values within a row with unlike superscript letters were significantly different (P < 0·05).
* SFO, addition of 6 % (DM basis) of linoleic acid-rich sunflower oil as a partial defaunating agent; BP, dry-rolled v. pelleted; SFO × BP, interaction.
† Treatment means for ruminal counts of protozoa during the measurement period (days 22–27).
‡ Includes Polyplastron, Diplodinium and Enoplastron spp.
For ruminal pH and NH3-N and VFA concentrations, sampling time × barley grain processing × ruminal protozoal status interactions were minor, so treatment means are presented (Table 4). Ruminal pH was higher (P = 0·001) in +SFO lambs compared with − SFO lambs. Ruminal pH was lower (P = 0·02) in lambs fed PB compared with those fed DRB. Ruminal NH3-N concentration was lower (P = 0·001) in +SFO lambs compared with − SFO lambs, and the difference in the ruminal NH3-N concentration between +SFO and − SFO lambs was greater with DRB than with PB (interaction; P < 0·01). Ruminal concentrations of total VFA, acetate and butyrate were lower (P = 0·001), whereas those of propionate were higher (P = 0·001) in +SFO lambs compared with − SFO lambs; consequently, the acetate:propionate ratio was lower (P = 0·001) in +SFO lambs compared with − SFO lambs. Ruminal concentrations of total and individual VFA were similar in lambs fed either DRB or PB, except for a higher (P = 0·001) ruminal concentration of valerate and a lower (P = 0·009) acetate:propionate ratio in lambs fed PB compared with those fed DRB.
Nitrogen balance and plasma urea-nitrogen concentration
Intakes of N were similar across treatments (Table 5). Faecal N excretion was unaffected by treatment. Urinary excretion of total N was 2·7–4·9 g/d less (P = 0·003) in +SFO lambs compared with − SFO lambs; consequently, total N excretion, when expressed as absolute amounts (P = 0·02) or as a proportion of N intake (P = 0·001), was lower, and N retention, when expressed as absolute amounts (P = 0·002) or as a proportion of N intake (P = 0·001), was higher in +SFO lambs compared with − SFO lambs. Lambs fed PB excreted 2·5–4·7 g/d less (P = 0·004) urinary N compared with those fed DRB. Total N loss, when expressed in absolute amounts (P = 0·01) or as a proportion of N intake (P = 0·001), was lower in lambs fed PB compared with those fed DRB. Consequently, N retention when expressed in absolute amounts tended to be greater (P = 0·09) or when expressed as a proportion of N intake was greater (P = 0·001) in lambs fed PB compared with those fed DRB. The PUN concentration was greater (P = 0·001) in − SFO lambs compared with +SFO lambs, but the method of barley grain processing had no effect on PUN concentration.
Table 5 Nitrogen intake, nitrogen balance and plasma urea-nitrogen in lambs fed dry-rolled or pelleted barley with (+SFO) or without (−SFO) sunflower oil (SFO) as a partial defaunating agent
(Mean values with their standard errors, n 4)

BP, barley grain processing.
a,b,c Mean values within a row with unlike superscript letters were significantly different (P < 0·05).
* SFO, addition of 6 % (DM basis) of linoleic acid-rich sunflower oil as a partial defaunating agent; BP, dry-rolled v. pelleted; SFO × BP, interaction.
Urea-nitrogen kinetics, mRNA abundance of urea transporter-B and microbial non-ammonia nitrogen supply
Across treatments, urinary [15N15N]urea enrichment reached a plateau within 24–48 h of isotopic infusion, whereas plateau enrichment of [14N15N]urea in urine was not attained until between 48 and 72 h of isotopic infusion (data not shown). Faecal 15N enrichments increased daily over the 4 d isotopic infusion without attaining a definite plateau (data not shown); therefore, for calculations of urea-N kinetics, faecal 15N enrichments over the final 72–96 h of infusion were used.
Only minor interactions on urea-N kinetics were detected between feeding SFO and the method of barley grain processing (Table 6). Endogenous urea-N production (i.e. urea-N entry rate; UER) was similar in − SFO and +SFO lambs (P = 0·48). The urea-N gastrointestinal entry rate (i.e. GER), when expressed in absolute amounts (P = 0·008) or as a proportion of UER (i.e. UER to GIT; P = 0·001), was greater in +SFO lambs compared with − SFO lambs. The urea-N that was used for anabolic purposes (i.e. UUA), when expressed in absolute amounts (P = 0·008) or as a proportion of GER (i.e. GER to UUA; P = 0·04), was higher in +SFO lambs compared with − SFO lambs. Conversely, the amount of UER that was lost in urine, when expressed in absolute amounts (P = 0·001) or as a proportion of UER (i.e. UER to urine; P = 0·001), was lower in +SFO lambs compared with − SFO lambs. The urea-N that re-entered the ornithine cycle (i.e. ROC) was unaffected by feeding SFO; however, when expressed as a proportion of GER (i.e. GER to ROC), there was a tendency (P = 0·08) for it to be lower in +SFO lambs compared with − SFO lambs. The urea-N to faecal excretion (i.e. UFE) was unaffected by feeding SFO. UER, GER, UUA, UFE and ROC were similar in lambs fed DRB or PB. The urinary urea-N elimination, when expressed in absolute amounts (P = 0·001) or as a proportion of UER (P = 0·01), was lower, whereas GER when expressed as a proportion of UER was higher (P = 0·01), in lambs fed PB compared with those fed DRB. The expression of UT-B mRNA was unaffected by treatment.
Table 6 Urea-nitrogen recycling kinetics, as measured using 4 d continuous intra-jugular infusions of [15N15N]urea, and urea transporter-B (UT-B) mRNA abundance in ruminal epithelial tissue in lambs fed dry-rolled or pelleted barley with (+SFO) or without (−SFO) sunflower oil (SFO) as a partial defaunating agent
(Mean values with their standard errors, n 4)

BP, barley grain processing; UER, urea-N entry rate; GER, gastrointestinal entry rate; ROC, urea-N that re-enters the ornithine cycle; UFE, urea-N to faecal excretion; UUE, urinary urea-N elimination; UUA, urea-N utilised for anabolic purposes; GAPDH, glyceraldehyde 3-phosphate dehydrogenase.
a,b,c Mean values within a row with unlike superscript letters were significantly different (P < 0·05).
* SFO, addition of 6 % (DM basis) of linoleic acid-rich sunflower oil as a partial defaunating agent; BP, dry-rolled v. pelleted; SFO × BP, interaction.
Urinary excretion of allantoin and total PD was higher (P = 0·001) and, correspondingly, microbial NAN supply estimated using urinary PD excretion and measured purine N:microbial N ratios was higher (P = 0·001) in +SFO lambs compared with − SFO lambs (Table 7). Compared with lambs fed DRB, those fed PB excreted more allantoin (P = 0·02) and tended to excrete more xanthine plus hypoxanthine (P = 0·06) and total PD (P = 0·09); however, microbial NAN supply was unaffected (P = 0·22) by barley grain processing.
Table 7 Urinary output, urinary purine derivative (PD) excretion and microbial non-ammonia nitrogen (NAN) supply in lambs fed dry-rolled or pelleted barley with (+SFO) or without (−SFO) sunflower oil (SFO) as a partial defaunating agent
(Mean values with their standard errors, n 4)
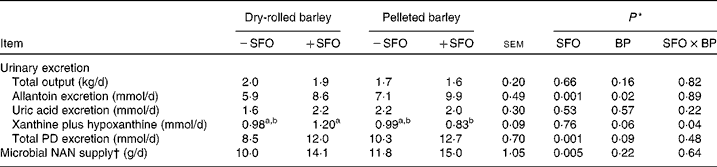
BP, barley grain processing; PD, purine derivatives.
a,b Mean values within a row with unlike superscript letters were significantly different (P < 0·05).
* SFO, addition of 6 % (DM basis) of linoleic acid-rich sunflower oil as a partial defaunating agent; BP, dry-rolled v. pelleted; SFO × BP, interaction.
† Microbial NAN supply was calculated according to Chen & Gomes(Reference Chen and Gomes27), using determined purine N:microbial N ratios.
Discussion
Feeding SFO to achieve partial defaunation in lambs had no impact on DM intake, which is in contrast to our previous study(Reference Kiran and Mutsvangwa2) in which we observed a decrease in DM intake when lambs were fed SFO. Lambs that were used in the present study were heavier (+17 kg) and older than those that were used in our initial study, and this could partly explain why responses in DM intake were different. As expected, based on previous studies in which SFO was used as a defaunating agent(Reference Kiran and Mutsvangwa2, Reference Ivan, Mir and Koenig17), ruminal protozoal counts decreased substantially within 6 d after the initiation of feeding SFO. Feeding PB also resulted in a decrease in ruminal protozoal counts when compared with DRB, supporting previous studies that have reported decreases in ruminal protozoal populations in ruminants fed extensively processed barley(Reference Koenig, Beauchemin and Rode13, Reference Eadie, Hyldgaard-Jensen and Mann14). The major changes that were observed in ruminal fermentation characteristics, i.e. a decrease in ruminal NH3-N, total VFA, acetate and butyrate concentrations and an increase in ruminal propionate concentration, are typical of changes that are associated with partial or complete defaunation(Reference Ivan, Mir and Koenig17, Reference Koenig, Newbold and McIntosh32, Reference Newbold, Chamberlain and Williams33). Ruminal pH was higher in +SFO lambs compared with − SFO lambs, which is in agreement with other studies(Reference Kiran and Mutsvangwa2, Reference Ivan, Mir and Koenig17, Reference Ivan, Mir and Mir34).
Total tract digestibilities of DM, OM, starch, N, acid-detergent fibre and neutral-detergent fibre were unaffected by feeding SFO to achieve partial defaunation, supporting previous findings(Reference Kiran and Mutsvangwa2). It appears that, because of the inhibition of protozoal activities in the rumen, most of the available data when high-oil diets are fed to achieve partial or complete defaunation indicate a decrease in ruminal OM or fibre digestion(Reference Oldick and Firkins35, Reference Faichney, Gordon and Welch36). If ruminal fibre digestion was depressed by feeding SFO in the present study, this was compensated for post-ruminally as total tract digestibilities were not affected. Both in situ ruminal and total tract starch digestibility were greater with PB compared with DRB. In common with the present study, Yang et al. (Reference Yang, Beauchemin and Rode37) reported increases in ruminal and total tract starch digestion, as the extent of barley grain processing was increased in dairy cows fed barley-based diets. The present results suggest that the lower ruminal digestion of DRB (as reflected by the lower in situ degradation kinetics relative to PB) was not compensated for by greater intestinal digestion, which supports previous findings(Reference Yang, Beauchemin and Rode37).
In ruminants, protozoa impose inefficiencies in N utilisation at a number of different levels, and the eradication of ruminal protozoa typically improves N utilisation(Reference Jouany4). In the present study, − SFO and +SFO lambs had similar N intakes and faecal N losses; however, feeding SFO to partially defaunate the rumen decreased total N excretion by 3·7–4·1 g/d, with most of this decrease in total N excretion being accounted for by a decrease in urinary urea-N excretion. Consequently, +SFO lambs retained 2·3–2·9 g/d more N when compared with − SFO lambs, thus suggesting a greater efficiency of N utilisation with defaunation as has been reported by others(Reference Kiran and Mutsvangwa2, Reference Jouany4). This improved N utilisation in defaunated ruminants is accounted for by various mechanisms, including a decrease in the ruminal NH3-N pool, coupled with a greater sequestration of NH3-N into bacterial protein(Reference Firkins, Yu and Morrison3), a decrease in intra-ruminal N recycling(Reference Jouany4) and an increase in microbial N flow to the duodenum by 20–60 %(Reference Jouany4, Reference Koenig, Newbold and McIntosh32). Besides the change in protozoal status, associated changes in energy supply and ruminal VFA patterns could also be partly responsible for improving the efficiency of post-absorptive N use in +SFO lambs. Assuming that the energy content of SFO is 36·8 MJ/kg, dietary supplementation with 6 % SFO increased the energy intake of +SFO lambs by 3·98–4·35 MJ of gross energy/d, and this additional energy intake could have influenced post-absorptive N use. In growing steers, increasing energy intake by abomasally infusing 150 g/d of a mixture of fatty acids (equivalent to an additional 5·44 MJ of gross energy/d) decreased urinary N excretion and increased N retention(Reference Schroeder, Titgemeyer and Awawdeh38), similar to the present findings. Also, the shift in ruminal VFA proportions that we observed with feeding SFO, with more propionate and less acetate, provided an additional glucogenic substrate that could have altered N use. In addition to improving propionate supply, supplemental SFO also could have provided another potential glucose precursor (glycerol) that could have improved N use. In ruminants wholly nourished by intra-gastric infusion, supplementation with glucogenic precursors improved N retention(Reference Chowdhury, Ørskov and De39, Reference Ørskov, Meehan and MacLeod40). This suggests that providing glucogenic precursors can potentially spare amino acids from being used for gluconeogenesis, thus making more amino acids available for protein accretion. Raggio et al. (Reference Raggio, Lobley and Lemosquet41) observed a decrease in whole-body leucine oxidation when lactating cows received intra-ruminal infusions of propionate, providing further evidence of a sparing effect of glucogenic precursors on amino acids.
A major objective of the present study was to determine the effects of feeding SFO as a defaunating agent on urea-N transfer to the GIT and the anabolic utilisation of the recycled urea-N in lambs. The present data on urea-N recycling kinetics offer interesting insights into additional potential mechanisms by which ruminal defaunation could improve N utilisation. The UER was similar in − SFO and +SFO lambs, reflecting the similar N intakes, as N intake and UER are positively correlated(Reference Marini, Klein and Sands8, Reference Wickersham, Titgemeyer and Cochran9). However, the GER, when expressed in absolute amounts or as a proportion of UER, was higher in +SFO lambs compared with − SFO lambs. Concomitant with these changes in the GER, urinary urea-N elimination when expressed in absolute amounts or as a proportion of UER was lower in +SFO lambs when compared with − SFO lambs. Also, although there were no differences between − SFO and +SFO lambs in the absolute amounts of ROC, the GER:ROC ratio tended to be lower in +SFO lambs compared with − SFO lambs. When taken together, these data clearly indicate that increased urea-N recycling to the GIT is also a major contributor to the improved N utilisation that has been consistently observed in defaunated ruminants. In our previous study, partial defaunation by feeding SFO was associated with a higher proportion of endogenous urea-N production being recycled to the GIT; however, N intake was lower in partially defaunated sheep compared with faunated sheep in that study(Reference Kiran and Mutsvangwa2). Because of the positive correlation between N intake and hepatic output of urea-N(Reference Marini, Klein and Sands8, Reference Wickersham, Titgemeyer and Cochran9), data on urea-N recycling kinetics were confounded due to these differences in N intake. In the present study, N intake did not differ due to feeding SFO, thus allowing more definitive conclusions to be made on the effects on urea-N kinetics of feeding SFO as a defaunating agent.
Endogenous urea-N can diffuse through all compartments of the GIT(Reference Lapierre and Lobley1), and although the [15N15N]urea infusion technique that was used in the present study cannot distinguish between urea-N transfer to the fore-stomach and post-stomach compartments, measurements of urea-N recycling to the GIT obtained using arterio-venous preparations indicated that in concentrate-fed ruminants (as in the present study), 65–76 % of urea-N transfer to the GIT entered the fore-stomach(Reference Huntington10, Reference Reynolds and Huntington42). It is urea-N that is transferred to the fore-stomach that can provide additional degradable N than be incorporated into microbial protein, thus contributing amino acids to the host animal after intestinal digestion. In the present study, however, we did not measure the direct incorporation of recycled 15N into microbial protein; rather, we measured anabolic utilisation (UUA) as part of the isotopic technique, in addition to estimating microbial NAN supply based on urinary excretion of PD(Reference Chen and Gomes27). The anabolic utilisation of recycled urea-N within the GIT, particularly the rumen, is assumed to be predominantly as sequestration of liberated NH3-N into microbial protein as most of the recycled urea-N first passes through the microbial N pool(Reference Lobley, Bremner and Zuur19), but other potential anabolic uses within the body could include amination and transamination reactions(Reference Lobley, Bremner and Zuur19). The ability to partition how much of the GER is directed towards anabolic and catabolic fates is a major advantage of the isotopic technique; however, a limitation is that UUA is calculated as the difference between GER and (ROC+UFE). Because faecal 15N enrichments failed to attain a definite plateau, UFE was probably underestimated; as such UUA was probably overestimated, so our data on UUA should be interpreted somewhat cautiously. However, when we used a curve fitting approach using the daily faecal 15N enrichments over the 4 d isotopic infusions (data not shown) to estimate plateau (maximum) enrichments, the measured ‘plateau’ enrichments were only 3·1–6·3 % lower than the mathematically derived ‘plateau’ enrichments. This suggests that UUA was only slightly overestimated. Others(Reference Lobley, Bremner and Zuur19, Reference Sunny, Owens and Baldwin43) have also reported a failure of faecal 15N enrichments to attain a definite plateau with 4 d isotopic infusions, so future studies should consider isotopic infusions longer than 4 d. For +SFO lambs, 0·546 of GER was directed towards anabolic fates, compared with only 0·456 for − SFO lambs. Furthermore, the ROC:GER ratio was 0·082 units lower for +SFO lambs compared with − SFO lambs, suggesting that more of the GER was used for productive purposes in +SFO lambs compared with − SFO lambs. In support of our findings of the improved productive use of recycled urea-N in partially defaunated lambs, microbial NAN supply was 27–41 % greater in +SFO lambs compared with − SFO lambs. It is plausible that urea-N that was recycled to the rumen could have buffered the rumen from the lower NH3-N concentrations prevailing in +SFO lambs, thus providing additional N that could be used for microbial protein synthesis. Additionally, because fat provides little energy for microbial growth(Reference Wu and Palmquist44), the positive response in intestinal microbial NAN flow when ruminal protozoal populations are decreased has also been attributed to decreased protozoal predation on bacteria and decreased competition from protozoa for growth substrates(Reference Jouany4). In calculating microbial NAN supply, we used purine N:N ratios that were determined in bacteria; however, microbial NAN supply in faunated and, to a much smaller extent, partially defaunated lambs was constituted of both bacterial and protozoal N. Because the purine N:N ratio has been reported to be higher for bacteria than for protozoa(Reference Firkins, Berger and Merchen45) and only the purine N:bacterial N ratio was used to calculate microbial NAN supply, it is possible that microbial NAN supply was underestimated particularly in faunated lambs(Reference Koenig, Newbold and McIntosh32). Nonetheless, despite these limitations, our data support previous findings of a greater microbial NAN flow to the intestine when ruminal protozoal populations are decreased(Reference Jouany4, Reference Koenig, Newbold and McIntosh32).
The major mechanism that could be responsible for the greater urea-N transfer to the GIT that we observed in partially protozoa-free lambs is plausibly the lower ruminal NH3-N concentration that was observed in these lambs compared with faunated lambs. Elevating ruminal concentration of NH3-N has been demonstrated to reduce urea-N transfer from blood into the rumen(Reference Rémond, Meschy and Boivin6). Also, other evidence indicates that the transepithelial movement of urea-N by passive diffusion into the rumen is facilitated by bacterial urease activity, which maintains a favourable concentration gradient(Reference Rémond, Meschy and Boivin6). Cheng & Wallace(Reference Cheng and Wallace7) demonstrated that urease activity in ruminal contents was negatively correlated with ruminal NH3-N concentration. Because feeding SFO to partially defaunate the rumen resulted in a lower ruminal NH3-N concentration, this could have facilitated the transfer of a greater proportion of UER to the GIT in +SFO lambs compared with − SFO lambs. In addition, the change in propionate supply could also have altered urea-N transfer to the GIT. Feeding SFO to partially defaunate the rumen increased ruminal concentrations of propionate and, presumably, the absorption of propionate from the rumen, as this is a concentration-dependent process(Reference Van Soest46). Propionate supplementation via intra-ruminal(Reference Savary-Auzeloux, Majdoub and LeFloc'h47) or intra-abomasal(Reference Kim, Choung, Dhamberlain, Lobley, White and MacRae48) infusion in sheep was associated with greater urea-N recycling to the GIT and N retention. The mechanism by which propionate increases urea-N recycling to the GIT is unclear, but it may be related to an alteration in ruminal bacterial activities and metabolism(Reference Savary-Auzeloux, Majdoub and LeFloc'h47) or changes in ruminal epithelial surface area and/or permeability(Reference Abdoun, Stumpff and Martens49), as propionate has been shown to stimulate epithelial cell proliferation(Reference Sakata and Tamate50).
Increasing ruminal energy availability by feeding PB compared with DRB decreased urinary urea-N and increased N retention, supporting previous studies(Reference Theurer, Huntington and Huber11, Reference Taniguchi, Huntington and Glenn51). These positive effects on N utilisation of increasing ruminal energy availability have been partly mediated via increased urea-N recycling to the rumen(Reference Kennedy and Milligan5, Reference Huntington10, Reference Theurer, Huntington and Huber11), in addition to a greater sequestration of N into microbial protein that is then available for intestinal digestion(Reference Theurer, Huber and Delgado-Elorduy52). Surprisingly, in the present study, all the major dependent variables quantifying urea-N recycling kinetics (i.e. UER, GER, UUA and ROC) were unaffected by barley grain processing, contradicting previous studies(Reference Huntington10, Reference Theurer, Huntington and Huber11). The magnitude of urea-N transfer across the ruminal wall appears to be regulated by many intra-ruminal factors, including ruminal pH(Reference Rémond, Meschy and Boivin6). In the present study, lambs that fed PB exhibited a lower ruminal pH compared with those fed DRB. A more acidic ruminal environment in lambs fed PB could have decreased bacterial urease activity in the rumen(Reference Gozho, Hobin and Mutsvangwa53), which, in turn, might have impaired urea-N transfer due to an unfavourable concentration gradient. Also, new evidence obtained using an in vitro isolated ruminal epithelium model indicates that urea-N transport is inhibited at luminal pH < 6·0(Reference Abdoun, Stumpff and Rabbani54), and mean pH in lambs fed PB was 5·8 compared with 6·0 for those fed DRB. Another surprising observation from the present study was that feeding PB did not stimulate microbial NAN supply when compared with feeding DRB, contradicting previous studies(Reference Huntington10, Reference Theurer, Huntington and Huber11). It is well established that the amount of microbial protein that is synthesised in the rumen is largely driven by the availability of energy from RFC. In the present study, in situ measurements of ruminal starch digestion clearly indicate that starch digestion was greater for PB compared with DRB. Also, total tract starch digestion was greater in lambs fed PB compared with those fed DRB. Together, these observations would suggest that, quantitatively, ruminal energy supply was greater with PB compared with DRB, so it is not clear why microbial NAN supply was not stimulated with PB. Because the diets that were fed in the present study contained only 16 g N/kg DM, it is likely that the rumen was limited in both NH3-N and amino acids to support microbial growth. Feeding PB to increase ruminal ATP availability when NH3-N and amino acids were potentially in short supply could have limited the use of the additional ATP for microbial protein synthesis(Reference Russell55). In that situation, the additional ATP could have been directed towards non-growth functions, a phenomenon referred to as energy spilling(Reference Russell55). In addition, Russell & Wilson(Reference Russell and Wilson56) observed inhibitory effects on microbial protein synthesis when ruminal pH was < 6·2, and this was partly attributed to increased energy spilling(Reference Russell55). In the present study, it is plausible that the more acidic ruminal environment in lambs fed PB could have compromised microbial growth by increasing energy spilling.
The presence of carrier-mediated, facilitative UT-B proteins has been demonstrated in the rumen of bovine(Reference Stewart, Graham and Cattell57, Reference Marini and Van Amburgh58) and ovine(Reference Marini, Klein and Sands8), and these UT-B proteins have been suggested to facilitate urea-N flux into the GIT lumen(Reference Lapierre and Lobley1). Our data indicate that changes in urea-N transfer to the GIT as a result of feeding SFO occurred without concomitant changes in UT-B expression, suggesting that the effects of ruminal NH3-N and propionate on urea-N transfer are probably not mediated through changes in UT-B expression. Recently, Simmons et al. (Reference Simmons, Chaudhry and Graham59) reported differences in UT-B expression between forage- and concentrate-fed steers, but ruminal NH3-N concentration was unaltered, and changes in ruminal VFA profiles were minimal. The limited research that is available on dietary regulation of UT expression in ruminal epithelium is equivocal(Reference Marini, Klein and Sands8, Reference Stewart, Graham and Cattell57, Reference Marini and Van Amburgh58), and additional research is needed to delineate the effects of diet and ruminal metabolites on UT expression.
In conclusion, collectively, these results provide the first major evidence that, at similar N intakes, the improved N utilisation that is typically observed in partially defaunated ruminants is partly mediated by an increase in urea-N transfer to the GIT and its anabolic use. This increase in urea-N recycling to the GIT in partially defaunated ruminants could be partly mediated by the decrease in ruminal NH3-N level, which has been demonstrated to stimulate transepithelial urea-N transfer. However, feeding SFO increased energy intake, in addition to altering the profile of energy metabolites entering portal blood from the rumen, and these changes in energy intake and energy metabolites could also be partly responsible for the observed changes in urea-N recycling and N utilisation. Although partial defaunation increased urea-N recycling to the GIT, the response was not augmented by increasing the amount of energy that is derived from carbohydrate fermentation in the rumen.
Acknowledgements
The present study was supported by a research grant from the Natural Sciences and Engineering Research Council of Canada held by T. M. The authors thank Réal Lepage and staff of the Livestock Research Building (University of Saskatchewan) for assistance with animal care, and Jason Marshall for assistance with quantitative PCR. T. M. and K. D. designed the research (i.e. conception of the project, development of overall research plan and study oversight). K. D. conducted the research, analysed data and performed statistical analysis. T. M. and K. D. wrote the manuscript, and T. M. had the primary responsibility for the final content of the manuscript. Both authors read and approved the final manuscript. There are no conflicts of interest.