A spinal cord injury (SCI) results from trauma to or disease of the spinal cord, often causing permanent neurological deficits and accelerated morbidity and mortality throughout the lifespan(Reference Cadotte and Fehlings1,Reference Zeilig, Dolev and Weingarden2) . Depending on level and completeness of injury, SCI is associated with a range of co-morbidities that can limit functional independence, mobility and nutrient utilisation. These co-morbidities include motor paralysis, sensory loss, neurogenic restrictive and obstructive pulmonary disease, neurogenic bradycardia, neurogenic hypotension, sympathetic dysfunction, neurogenic adaptive myocardial atrophy, coronary artery disease, anabolic deficiency, spasticity, sarcopenia, heterotopic ossification, osteoporosis, upper extremity overuse, neurogenic obesity, cardiometabolic syndrome (CMS; including, dyslipidemia, hypertension and type 2 diabetes mellitus), pressure injuries, sexual dysfunction, and neurogenic bowel and neurogenic bladder(Reference Gater3).
In the acute phase of SCI, spinal shock often occurs in which all motor and sympathetic reflex activity is absent(Reference Ko4). The patient is often mechanically ventilated in the acute phase, such that even muscles of respiration are inactive(Reference Gater5). Basal/resting metabolism plummets as the body sheds unneeded paralysed muscle and bone, and nutrient needs are reduced(Reference Wing6). Once weaned from a ventilator, the individual will at least be able to activate muscles of respiration which may marginally increase total daily energy expenditure (TDEE) as they contract. During this time, the body will continue to lose unused muscle and bone until a homoeostasis is attained with minimal muscle protein reserve and bone mineral content reduced to fracture threshold(Reference Gater5,Reference Wing6) . The acute phase may last up to 12 months as the individual completes physical rehabilitation and reintegrates into the community with a new baseline functional level, and subsequent energy and nutrient ‘setpoint’, that falls well below previous baseline levels(Reference Gater5–Reference Nash, Groah and Gater8). The new setpoint is rarely matched by a similar reduction in energetic intake(Reference Farkas, Gorgey and Dolbow9). Overeating relative to energetic need and poor dietary habits (e.g. overeating, consuming sugary drink, etc.) contribute to inadequate nutrition and chronic health problems in the population with SCI(Reference Nash, Groah and Gater10,Reference Gater, Farkas and Berg11) . Therefore, ensuring the appropriate consumption of macronutrients and micronutrients relative to need becomes a challenge despite several dietary recommendations.
Authoritative guidelines provide evidence-based dietary recommendations. These guidelines are used to establish goals in planning healthy diets and lifestyles and provide the public information about nutritional science and a wholesome diet. The US Department of Agricultural (USDA) Dietary Guidelines for Americans (DGA)(12), Public Health England (PHE) Dietary Recommendations(13), Australian Dietary Guidelines (ADG)(14) and Institute of Medicine (IOM) Dietary Reference Intakes(15) are meant for use by healthy populations to meet nutritional needs and maintain an overall healthy diet and lifestyle. Both the WHO Healthy Diet(16) and the American Heart Association (AHA)(Reference Jensen, Ryan and Apovian17,Reference Eckel, Jakicic and Ard18) primarily focus on the prevention of obesity and chronic disease and, in the case of the AHA, reducing the risk of cardiovascular disease. Food and estimated average requirements, recommended dietary allowance, adequate intakes and tolerable upper-level intake (definitions are provided in Table 1) recommendations from these organisations are often harmonised. However, they differ in their methodologies, ratings of evidence, geographic location of the population of interest, references and ease of translation. A fundamental shortcoming of the guidelines is their translation to persons with life-changing injuries and/or those who have developed chronic health conditions that require special dietary modifications and considerations, such as those with a SCI. Persons with special needs are typically excluded from consideration when designing these guidelines.
Table 1. Definition of terms relating to dietary reference intakes

Adapted from US Department of Agricultural (USDA) Dietary Guidelines for Americans (DGA)(Reference Cadotte and Fehlings1) and Institute of Medicine (IOM) Dietary Reference Intakes(Reference Zeilig, Dolev and Weingarden2).
The overall purpose of this narrative review is to (1) critically appraise dietary intake relative to energy needs after SCI and (2) compare the existing literature with authoritative dietary guidelines from several countries that target obesity and cardiometabolic risk reduction. We extend the findings from our published meta-analysis and systematic review(Reference Farkas, Pitot and Berg19) that determined greater energetic intake relative to energy expenditure and an imbalance in fibre and micronutrient intake compared with the DGA in chronic SCI. In the present paper, we specifically aim to review both the acute (< 1-year post-injury) and chronic (≥ 1-year post-injury) phases of a SCI, include a wider collection of dietary and energy literature in SCI, critically evaluate energy expenditure and dietary intake assessment methods, incorporate dietary guidelines outside the US, and provide practical assessment and nutritional recommendations to facilitate a healthy dietary pattern after SCI. We also highlight neurogenic obesity and cardiometabolic risk after SCI, explore the potential influence of SCI on the central and peripheral mechanisms regulating energy homoeostasis and provide direction for future research by comparing existing literature on persons with and without SCI.
Neurogenic obesity and cardiometabolic risk after spinal cord injury
The prevalence of neurogenic obesity(Reference Farkas and Gater7,Reference Gater, Farkas and Tiozzo20) in adults with SCI ranges from 22 % to 97 %, compared with 42 % in the non-disabled population(Reference Gater5,Reference Gater, Farkas and Berg11,Reference Weaver, Collins and Kurichi21–Reference Gater, Farkas and Dolbow29) . Neurogenic obesity results from the dysfunction of energy metabolism, physical deconditioning(Reference Maher, McMillan and Nash30), a sedentary lifestyle(Reference Dearwater, Laporte and Robertson31), impaired fitness(Reference Noreau, Shephard and Simard32), sympathetic nervous system dysfunction(Reference Stjernberg, Blumberg and Wallin33,Reference Monroe, Tataranni and Pratley34) , altered hormonal homoeostasis(Reference Gater5,Reference Bauman, La Fountaine and Spungen35–Reference Clark, Schopp and Mazurek40) , changes in satiety(Reference Holmes41) and loss of lean body mass after SCI (Table 2)(Reference Modlesky, Bickel and Slade42–Reference Gorgey and Dudley47). The volume of marrow fat increases 36 % following the initial 12 weeks after the injury in part, because increases in fat mass are dissociated from obesity-related mechanical loading(Reference Minaire, Edouard and Arlot48). Following the SCI, bone loss is prompt(Reference Edwards, Schnitzer and Troy49), with bone mineral density at the knee and hip declining 2 to 4 % every month(Reference Edwards, Schnitzer and Troy49,Reference Bieringsorensen, Bohr and Schaadt50) and decreasing up to roughly 20 %(Reference Goenka, Sethi and Pandey51,Reference Bauman, Cirnigliaro and La Fountaine52) within the first year of the injury. Precipitous loss of skeletal muscle mass below the level of injury (LOI) is marked by decreased cross-sectional area of up to 48 % as immediate as 6 weeks after the injury(Reference Castro, Apple and Hillegass45). Muscle atrophy from 30 to 60 % of total lean body mass has also been reported(Reference Qin, Bauman and Cardozo53). Significant gains in fat mass occurring 2 to 7 months post-SCI contribute to a pathological cardiometabolic profile observed in the chronic phase of the injury(Reference Groah, Nash and Ward54).
Table 2. Factors contributing to neurogenic obesity and cardiometabolic syndrome after spinal cord injury (SCI)

Adapted from Farkas and Gater(Reference Farkas and Gater7), Gater et al.(Reference Gater5) and Nash et al.(Reference Nash, Groah and Gater8).
The accumulation of visceral fat is considered the principle mediator in the development of dyslipidemia, insulin resistance, hypertension, arteriosclerosis and CMS in the non-disabled population(Reference Balistreri, Caruso and Candore55–Reference Ouchi, Parker and Lugus57). Similar risk factors are used to quantify CMS in persons with SCI (Table 2)(Reference Farkas and Gater7,Reference da Silva Alves, de Aquino Lemos and Ruiz da Silva58–Reference Morse, Stolzmann and Nguyen64) . Cirnigliaro et al. (Reference Cirnigliaro, LaFountaine and Dengel65) reported that, when compared with a non-disabled group, persons with SCI had a 27 % increase in visceral fat volume for every centimetre increase in waist circumference, a marker of central obesity, and a 20 % increase in visceral fat volume for every unit increase in body mass index (BMI). Nash et al. (Reference Nash, Tractenberg and Mendez66) identified that being overweight/obese was significantly associated with CMS diagnosis. In a sample of 477 veterans with SCI, Gater et al. (Reference Gater, Farkas and Berg11) reported that 76·7 % were classified as obese when using an SCI-specific BMI cut-off of 22 kg/m2(Reference Laughton, Buchholz and Martin Ginis67). The authors also reported that 55·1 % had or were undergoing treatment for hypertension; approximately 50 % currently had or were previously diagnosed with type 2 diabetes mellitus; 69·7 % had or were under treatment for HDL-cholesterol < 40 mg/dl; and more than 57 % had CMS using modified International Diabetes Federation criteria(Reference Alberti, Zimmet and Shaw68). More recently, Gater and colleagues(Reference Gater, Farkas and Dolbow29) studied body composition using the gold standard four-compartment model and CMS in a sample of seventy-two participants with chronic motor complete SCI. The authors identified a mean BMI of 27·3 kg/m2 corresponding to 42 % body fat and CMS was in 59·4 % of the sample(Reference Gater, Farkas and Dolbow29). These findings demonstrate the high prevalence of neurogenic obesity and cardiometabolic complications after SCI and the need for dietary countermeasures (Table 2).
Central and peripheral mechanisms regulating energy homoeostasis and their implications in spinal cord injury
The central nervous system plays a vital role in modulating energy status, and the hypothalamus is the integrating, superordinate principal regulator of whole-body energy homoeostasis (Fig. 1). The arcuate nucleus within the hypothalamus plays a critical role in the regulation of feeding and metabolism. It integrates hormonal and nutritional signals from the peripheral circulation, as well as peripheral and central neuronal inputs, to generate a coordinated feedback response. The arcuate nucleus projects to second-order neurons in the paraventricular, dorsomedial, lateral and ventromedial nuclei of the hypothalamus. The second-order neurons further process the received information and project to multiple extrahypothalamic neurocircuits, leading to an integrated response that regulates energy intake and energy expenditure(Reference Timper and Brüning69). These centres jointly summate influences from various circulating substrates, hormones, neuropeptides and neurotransmitter signals that regulate food intake.

Fig. 1. The neuroendocrine components involved in the regulation of energy balance relevant to spinal cord injury. Organs and systemic signaling pathways are represented with green lines (circulating hormonal signals), red (voluntary neurological signals) and blue (autonomic neurological signals). The pop-out shows the action of these signals on regions in the hypothalamus and brainstem. Legend: 3V, third ventricle; ARC, arcuate nucleus; Carbs, carbohydrates, CCK, cholecystokinin; DMH, dorsomedial hypothalamic nucleus; FGF, fibroblast growth factors; FFA, free fatty acids; GLP-1, glucagon-like peptide 1; lat, lateral nucleus; n., nerve; PVN, paraventricular nucleus; P-YY-3–36, peptide YY3–36; SN, substantia nigra; and VMH, ventromedial hypothalamic nucleus. , Humoral;
, Autonomic;
, Voluntary
Gastrointestinal hormones also have a principal role in regulating central nervous system-dependent energy control. Ghrelin is mainly secreted from the stomach during a fasted state and stimulates body weight gain, adiposity and central feeding centres by activating neurons in the hypothalamus that stimulate food intake(Reference Timper and Brüning69). Various other hormones, such as peptide YY3–36, cholecystokinin and glucagon-like peptide 1 are secreted from the small intestine upon the ingestion of foodstuff and exert appetite-suppressing effects in various brain regions, such as hypothalamic and brainstem nuclei, and by modulating vagal afferents, the peripheral elements of the brain–gut axis (Fig. 1)(Reference Crespo, Cachero and Jiménez70). Gut peptides provide information on ‘real-time’ food consumption and modify electrical activity of the vagal afferent pathway by attaching to vagal receptors that extend into the digestive tract mucosa. These intestinal-derived signals are sent by the vagus nerve to the nucleus of the solitary tract, with further projection to hypothalamic regions (Fig. 1)(Reference Miller71).
Disruption of the central mechanisms modulating energy metabolism has been previously recognised as the aetiology of obesity in non-disabled persons(Reference Timper and Brüning69,Reference Miller71) . Obesity and cardiometabolic disorders are frequently associated with diminished production or resistance to the production of central and peripheral regulators of energy homoeostasis, including food intake and energy expenditure(Reference Elmquist and Joel72). Naznin et al. (Reference Naznin, Toshinai and Waise73) and Waise et al. (Reference Waise, Toshinai and Naznin74) reported that obesity-induced systemic inflammation spreads to the vagus nerve and subsequently the hypothalamus leading to the dysregulation of central and peripheral mechanisms governing satiety, energy regulation and fuel metabolism. With obesity and high-fat, energy-dense diets, increases in the concentration of saturated fatty acids from the periphery cross the blood–brain barrier and induce an inflammatory response on hypothalamic neurons(Reference Thaler, C-X and Schur75). Vinik et al. (Reference Vinik, Maser and Mitchell76) reported that obesity and type 2 diabetes mellitus-induced neuropathies alter vagal nerve neurotransmission, preventing bidirectional crosstalk between the central nervous system and the gut.
Although supraspinal centres remain intact following SCI, several neurological and endometabolic factors are influenced by the disruption of the central nervous system. With an SCI, compromised afferent and efferent signals from central and peripheral locations lead to dysregulation of the intricate equilibrium of energy metabolism. Physiological cues that are present in persons with SCI and influence appetite, satiety/satiation and energy balance are disrupted, further contributing to an imbalance in energy homoeostasis(Reference Holmes41). Besecker et al. (Reference Besecker, Blanke and Deiter77) have proposed an SCI-induced gastric vagal afferent neuropathy as a cause for homoeostatic dysregulation of energy balance in experimental SCI in rats. The authors hypothesise the disruption of the reflex transmission of chemical feeding-related signals from the gastrointestinal tract to the CNS(Reference Besecker, Blanke and Deiter77). The central and hypothesised peripheral dysregulation of energy homoeostasis and a deterioration of body composition that results in physical deconditioning produce the ‘perfect storm’ for the onset of neurogenic obesity and cardiometabolic risk in persons with SCI.
Energy expenditure after spinal cord injury
Energy balance reflects a dynamic relationship between energy expenditure and energy intake. TDEE represents the number of energies burned over 24 h and is the sum of basal metabolic rate (BMR), the thermic effect of physical activity (TEPA) and the thermic effect of food digestion (TEF)(Reference Farkas, Pitot and Gater78).
In the non-disabled population, TEPA and TEF account for approximately 20 % and 8% of TDEE(Reference Farkas, Pitot and Gater78), whereas after SCI they account for about 5 % and 6 %, respectively(Reference Monroe, Tataranni and Pratley34,Reference Buchholz, McGillivray and Pencharz79) . To date, limited research has tested differences in the TEPA and TEF between persons with and without SCI. Monroe et al. (Reference Monroe, Tataranni and Pratley34), measured TEPA using a respiratory chamber. They observed significantly less TEPA in men with SCI compared with men without SCI(Reference Monroe, Tataranni and Pratley34). This finding is likely because movement is restricted to the upper limbs. Consequently, the energy cost of exercise and activities of daily living is significantly lower in SCI compared with a non-disabled person(Reference Collins, Gater and Kiratli80). Aksnes et al. (Reference Aksnes, Brundin and Hjeltnes81) and Buchholz et al. (Reference Buchholz, McGillivray and Pencharz79)did not find differences in TEF between persons with chronic SCI and non-disabled controls, possibly because of a 2-h post-prandial testing window that only captured the parasympathetic-controlled obligatory phase of TEF. Alternatively, Monroe et al. (Reference Monroe, Tataranni and Pratley34) and Asahara and Yamasaki(Reference Asahara and Yamasaki82) reported significant differences between persons with and without SCI for a 24- and 3-hour test, respectively. The authors of both studies incorporated a longer testing duration, therefore, capturing both the obligatory and the sympathetic and skeletal muscle mass-mediated facultative phases of TEF(Reference Asahara and Yamasaki82). Both TEF and TEPA present unique assessment challenges attributed to the scarcity of literature. Therefore, TEF and TEPA should remain an activeresearch focus given their influence on energy intake and TDEE.
BMR typically accounts for 60 to 70 % of TDEE in the non-disabled population(Reference Melanson83), but in persons with SCI, it accounts for 70 to 80 %(Reference Gorgey, Caudill and Sistrun84). Fat-free mass, composed of bone, muscle and organs, contributes the most to BMR, and of fat-free mass, skeletal muscle mass accounts for 85 % of the variance. Attenuation of BMR following SCI originates from a significant reduction in metabolically active tissue(Reference Farkas, Gorgey and Dolbow9), sympathetic nervous system dysfunction(Reference Buchholz and Pencharz85) and altered hormonal milieu(Reference Gorgey, Khalil and Gill86).
Most SCI literature measures resting metabolic rate (RMR) or resting energy expenditure rather than the more precise measurement of BMR (Table 3). The available literature indicates that the mean measured BMR for persons with SCI ranges from 1022 to 1943 kcal/d, and mean measured RMR ranges from 959 to 2519 kcal/d (Table 3)(Reference Farkas, Gorgey and Dolbow9,Reference Monroe, Tataranni and Pratley34,Reference Buchholz, McGillivray and Pencharz79–Reference Aksnes, Brundin and Hjeltnes81,Reference Gorgey, Caudill and Sistrun84,Reference Bauman, Spungen and Wang87–Reference Aquilani, Boschi and Contardi120) . Buchholz et al. (Reference Buchholz, McGillivray and Pencharz79) reported resting metabolism was significantly lower in persons with paraplegia (1472 kcal/d) compared with BMI-matched non-disabled controls (1677 kcal/d). In another study by Buchholz et al. (Reference Buchholz, McGillivray and Pencharz121), authors examined RMR in twenty-seven persons with SCI by injury completeness (complete: 1417 v. incomplete: 1480 kcal/d) and sex (men: 1555 v. women: 1245 kcal/d), only observing significant differences by sex. Gorgey et al. (Reference Gorgey, Farkas and Dolbow110) recently reported non-significant differences in BMR by sex (men: 1421 v. women: 1367 kcal/d) and Farkas et al.(Reference Farkas, Gorgey and Dolbow9) observed significant differences by LOI (tetraplegia: 1224 and paraplegia: 1517 kcal/d) in motor complete SCI. Collins et al. (Reference Collins, Gater and Kiratli80), however, did not report significant differences in RMR by LOI. These differences may stem from population demographics as Farkas et al. (Reference Gorgey, Farkas and Dolbow110) examined chronic motor complete SCI, whereas Collins et al. (Reference Collins, Gater and Kiratli80) included complete and incomplete SCI. Additionally, Farkas et al. (Reference Gorgey, Farkas and Dolbow110) measured BMR and Collins et al. (Reference Collins, Gater and Kiratli80) assessed RMR in their respective studies.
Table 3. BMR/RMR in spinal cord injury literature

LOI, level of injury; AIS, American Spinal Injury Association Impairment Scale; TSI, time since injury; Para, paraplegia; PI, pressure injuries; C, complete; I, incomplete; Tetra, tetraplegia.
Blank spaces indicate data were not provided in the study; data are presented as mean ± standard deviation.
* RMR/resting energy expenditure was measured.
† Standard deviation not provided.
** BMR was measured.
RMR and BMR primarily differ in testing procedures, but both are non-invasively measured with indirect calorimetry using a metabolic cart(Reference Farkas, Pitot and Gater78). The participant lies in a supine position in a dark room with minimal movement following at least an 8-h fast for RMR or a 12-h fast for BMR(Reference McMurray, Soares and Caspersen122). Because RMR is not at a basal state, it is usually higher than BMR for persons with and without SCI as only a short quiescent period is required (10 to 20 min(Reference Compher, Frankenfield and Keim123)) prior to data acquisition(Reference Bauman, Spungen and Wang87). Rather for BMR, the participant is awakened in the morning following an overnight stay, refrains from exercise, caffeine and alcohol for the previous 24 h, is free from emotional stress, and familiar with the apparatus(Reference McMurray, Soares and Caspersen122,Reference Henry124) . Bauman et al. (Reference Bauman, Spungen and Wang87) examined BMR and RMR in pairs of monozygotic twins with and without SCI. The authors reported lower basal metabolism (SCI twin: 1387 and non-SCI twin: 1660 kcal/d) in both groups compared with RMR values (SCI twin: 1682 and non-SCI twin: 1854 kcal/d). Additionally, both values were significantly lower in SCI compared with individuals without the injury(Reference Bauman, Spungen and Wang87). There is an approximate 20 % difference between BMR and RMR values in persons with SCI compared with an 11% difference in persons without SCI. Considering that resting/basal metabolism are the largest components of TDEE in persons with and without SCI and it is significantly influenced by fat-free mass, several studies have reported that RMR can be used as a strong predictor of energy intake(Reference Blundell, Caudwell and Gibbons125–Reference Hopkins, Finlayson and Duarte128). When using RMR rather than BMR as a predictor of energetic intake in persons with SCI, dietary need can be overestimated by nearly 400 kcal/d (assuming approximately a 1900 kcal/d diet(Reference Farkas, Pitot and Berg19)). These data indicate BMR is a more sensitive indicator of energetic need, and less reliance should be placed on RMR in SCI research. However, TDEE remains superior as it accounts for the multiple components of daily energy expenditure.
According to published studies, during the acute phase of SCI, TDEE ranges from 2030 to 3344 kcal/d (Table 4)(Reference Cox, Weiss and Posuniak89,Reference Barco, Smith and Peerless93,Reference Desneves, Panisset and Rafferty94,Reference Rowan and Kazemi97,Reference Wouda, Lundgaard and Becker101,Reference Wouda, Lundgaard and Becker102,Reference Rodriguez, Benzel and Clevenger129) . In chronic SCI, TDEE is from 1332 to 2834 kcal/d (Table 4)(Reference Farkas, Gorgey and Dolbow9,Reference Monroe, Tataranni and Pratley34,Reference Desneves, Panisset and Rafferty94,Reference Shea, Shay and Leiter98–Reference Tanhoffer, Tanhoffer and Raymond100,Reference Buchholz, McGillivray and Pencharz121,Reference Mollinger, Spurr and el Ghatit130) . TDEE is reduced in persons with chronic SCI by as much as 54 % in persons with tetraplegia(Reference Mollinger, Spurr and el Ghatit130) and nearly 20 % in individuals with paraplegia(Reference Buchholz, McGillivray and Pencharz121). TDEE can be assessed by measuring average daily energy expenditure using direct, or whole body, calorimetry, doubly labelled water, or mechanical ventilation. Of the SCI literature that assessed TDEE, only 33 % measured TDEE. Direct calorimetry measures the amount of heat produced while enclosed within a respiratory chamber and is the gold standard for measuring energy metabolism(Reference Farkas, Pitot and Gater78). A test participant is completely enclosed in the chamber where there are no social interactions during the measurements and audiovisual contact with investigators(Reference Schutz131). In cross-sectional study designs, the participants spend a minimum of 24 h continuously, and up to a week (or more) in dietary intervention studies(Reference Schutz131). This method has several limitations, including the cost of highly specialised equipment, space to house the equipment, confinement of the participant and the need to exclude anything emitting heat other than the research subject. For persons with paralysis, and especially high injury levels, direct calorimetry is unrealistic because of the need for caregiver assistance, power wheelchairs and/or assistive electronic devices. To date, Monroe and colleagues(Reference Monroe, Tataranni and Pratley34) are the only investigators to use direct calorimetry with a respiratory chamber in persons with SCI. The authors demonstrated a TDEE of 1870 kcal/d in chronic complete SCI compared with 2376 kcal in persons without SCI(Reference Monroe, Tataranni and Pratley34), a 24 % difference.
Table 4. TDEE after SCI

SCI, spinal cord injury; LOI, level of injury; AIS, American Spinal Injury Association Impairment Scale; TSI, time since injury; TDEE, total daily energy expenditure; Para, paraplegia; C, complete; I, incomplete; Tetra, tetraplegia;
Blank spaces indicate data were not provided in the study; data are presented as mean ± standard deviation.
* Standard deviation are not provided.
Less labour- and time-intensive methods to measure energy expenditure are with doubly labelled water or a metabolic cart during mechanical ventilation. Doubly labelled water is centred around the difference between the apparent turnover rates of the hydrogen and oxygen of body water as a function of carbon dioxide production. The procedure encompasses enriching a research participant with heavy oxygen and heavy hydrogen and then determining the difference in washout kinetics between the isotopes. The oxygen isotope is lost as water and as carbon dioxide due to exchange in the bicarbonate pools. The hydrogen isotope is lost only as water(Reference Westerterp132). The strength of doubly labelled water is that it is a non-invasive and inconspicuous free-living evaluation of TDEE with no constraint or restriction for the participant. The total number of variables in the equations used to calculate TDEE from doubly labelled water is nine, plus two additional constants, from which the equation for isotope dilution spaces calculation includes five variables and one constant(Reference Schutz131), thus, making the technique mathematically complex and prone to miscalculation. Double labelled water is infrequently used (Desneves et al. (Reference Desneves, Panisset and Rafferty94) and Tanhoffer et al. (Reference Tanhoffer, Tanhoffer and Raymond99,Reference Tanhoffer, Tanhoffer and Raymond100) ) to measure TDEE after SCI. In 2012 and 2015, Tanhoffer et al. (Reference Tanhoffer, Tanhoffer and Raymond99,Reference Tanhoffer, Tanhoffer and Raymond100) reported a mean TDEE of 2346 and 2406 kcal/d, respectively, in chronic SCI, while Desneves et al. (Reference Desneves, Panisset and Rafferty94) reported 2354 kcal/d during the acute stage. These observed values far exceed the reported values by Monroe et al. (Reference Monroe, Tataranni and Pratley34) In the acute phase, mechanical ventilators provide a unique option to continuously measure respiratory gases with the addition of a metabolic monitor, similar to indirect calorimetry(Reference Weissman, Sardar and Kemper133), as published by Barco et al. (Reference Barco, Smith and Peerless93) Because nutritional risk is associated with ventilatory support after SCI and high levels of injury often need respiratory management(Reference Wong, Derry and Jamous134), the use of metabolic monitoring with mechanical ventilators can provide an easy method to determine energetic need during the initial hospitalisation. However, it is likely because of the lack of availability of the specialised equipment, cost and trained personnel required to measure TDEE that most studies rely on predicting TDEE, a method prone to error(Reference Farkas, Pitot and Gater78). Of the literature that assessed TDEE, 71 % estimated it through various methods (Table 4). Several of these methods are examined in the next section given their clinical use in defining energetic targets.
Energy (energetic) intake relative to energy expenditure after spinal cord injury
Energy intake reflects energetic gain by the ingestion of foodstuff of different energetic densities. Defining optimal nutrient intake and its management is challenging after SCI because practical guidelines for determining energy requirements for this niche population are limited. Energetic need is dependent on several factors after SCI, including the injury phase(Reference Perret and Stoffel-Kurt117) and the physical activity/therapy within phase(Reference Mollinger, Spurr and el Ghatit130), the level(Reference Farkas, Gorgey and Dolbow9,Reference Cox, Weiss and Posuniak89) and completeness(Reference Mollinger, Spurr and el Ghatit130) of the injury, sex(Reference Groah, Nash and Ljungberg135), body composition and its post-injury changes(Reference Buchholz and Pencharz85,Reference Yilmaz, Yasar and Goktepe103) , presence of infection or pressure injuries(Reference Aquilani, Boschi and Contardi120), and frequency and accuracy of reporting on the energetic intake used to assess nutrition(Reference Farkas, Pitot and Berg19,Reference Gorgey, Caudill and Sistrun84) . Consequently, tremendous variability in energetic intake (Table 5) is reported across the literature, even though most studies report it is within or exceeds daily AHA, ADG, DGA and PHE recommendations (Table 6).
Table 5. Total energetic and macronutrient intake in SCI literature
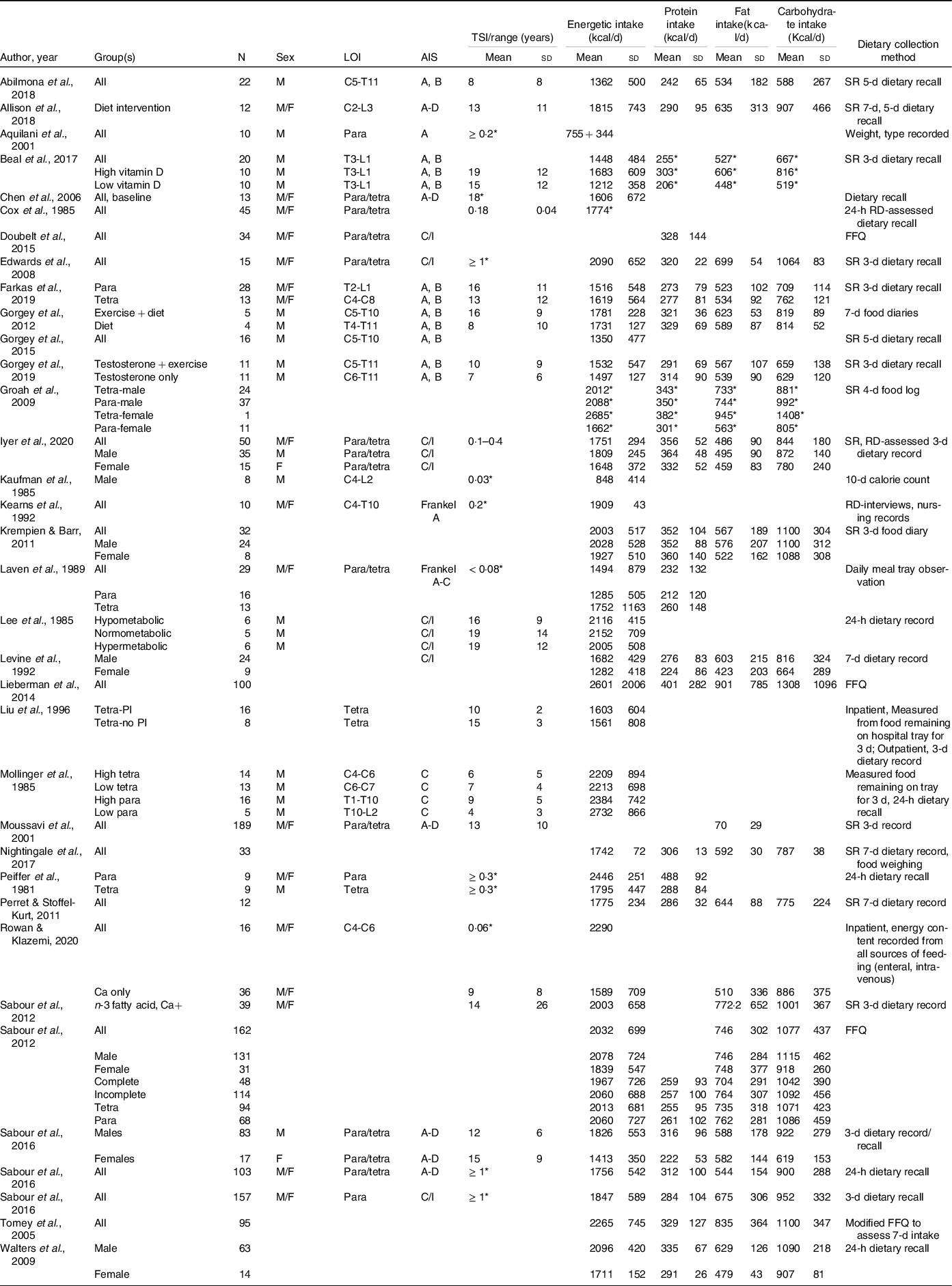
SCI, spinal cord injury; LOI, level of injury; AIS, American Spinal Injury Association Impairment Scale; TSI, time since injury; SR, self-report; Para, paraplegia; Tetra, tetraplegia; C, complete; I, incomplete; PI, pressure injuries; FFQ, food frequency questionnaire; RD, registered dietitian.
Blank spaces indicate data were not provided in the study; data are presented as mean ± standard deviation.
* Standard deviation not provided.
Table 6. Comparison of authoritative, evidence-based non-spinal cord injury dietary guidelines

AMDR, Acceptable Macronutrient Distribution Range; DGA, Dietary Guidelines for American; AI, adequate intake; UL, tolerable upper intake level.
* Guidelines were established to prevent CVD and manage overweight and obese adults.
† Estimated energy requirement equation for males = 662 – (9·53 × age (years)) + PA × ((15·91 × weight (kg)) + (539·6 × height (m))), where PA is the physical activity coefficient.
‡ Estimated energy requirement equation for females = 354 – (6·91 × age (years)) + PA × ((9·36 × weight (kg)) + (726 × height (m))), where PA is the physical activity coefficient.
§ Values in parentheses are an example of the total g/d of total fibre calculated from 14 g/1000 kcal multiplied by the median energy intake (kcal/1000 kcal/d) from the Continuing Survey of Food Intakes by Individuals (1994–1996, 1998).
The IOM encourages establishing energetic intake using a sex-specific prediction equation, relying on age, height, weight and physical activity. While less precise than measuring energy requirements, the IOM is the only guideline that makes such a recommendation rather than providing an acceptable macronutrient distribution range. A limitation of IOM’s equation is that it neglects to include resting or basal metabolism as the largest determinant of TDEE, and therefore energetic intake. TDEE can also be estimated using the product of BMR (or RMR) and the common activity correction factor of 1·2(Reference Farkas, Gorgey and Dolbow9). Several studies have predicted TDEE using 1·2 and other previously published activity, stress, injury, and/or trauma correction factors to determine energetic intake for persons with SCI(Reference Monroe, Tataranni and Pratley34,Reference Cox, Weiss and Posuniak89,Reference Rowan and Kazemi97,Reference Shea, Shay and Leiter98,Reference Wouda, Lundgaard and Becker101,Reference Wouda, Lundgaard and Becker102,Reference Buchholz, McGillivray and Pencharz121,Reference Rodriguez, Benzel and Clevenger129) .
In the acute phase of SCI, the literature indicates total energetic intake ranges from 755 to 2290 kcal/d (Table 5)(Reference Cox, Weiss and Posuniak89,Reference Rowan and Kazemi97,Reference Kearns, Thompson and Werner119,Reference Aquilani, Boschi and Contardi120,Reference Iyer, Beck and Walton136–Reference Laven, Huang and DeVivo138) . Over the first 4 weeks of the SCI, this value increases by over 400 kcal/d(Reference Kearns, Thompson and Werner119) and likely results from the thermic effect of voluntary respiration (diaphragmatic and intercostal muscle activation) for those weaned from mechanical ventilators. The conversion from the catabolic state to declining energetic needs is not well researched, though RMR has been shown to decrease 10 weeks post-SCI(Reference Felleiter, Haeberli and Schmid139). In seminal work by Cox et al. (Reference Cox, Weiss and Posuniak89), the authors reported that persons in the early rehabilitation phase of the injury require up to 54 % less energy content than would be predicted by most standard formulae. The authors further determined that in the rehabilitation phase, persons with tetraplegia need 22·7 kcal/kg/d and persons with paraplegia need 27·9 kcal/kg/d(Reference Cox, Weiss and Posuniak89), guidelines that are still widely used today(140). Of note, this calculation published by Cox et al. (Reference Cox, Weiss and Posuniak89) was developed in fifteen persons with tetraplegia and five with paraplegia and mostly men (86 %) whom typically expend(Reference Gorgey, Farkas and Dolbow110,Reference Buchholz, McGillivray and Pencharz121) and consume(Reference Iyer, Beck and Walton136,Reference Sabour, Soltani and Latifi141) more than women. The equations also do not account for the serial weight loss and weight regain that occurs during the early phase of the SCI and a drop in energy expenditure that persists through the rehabilitation phases of treatment.
When evaluating the energy intake and expenditure data, persons with acute SCI appear to be in a negative energy balance (TDEE: 2030 to 3344 kcal/d v. energetic intake: 755 to 2290 kcal/d). Distinct from other trauma conditions, persons with acute SCI do not demonstrate hypermetabolism following injury(Reference Kearns, Thompson and Werner119,Reference Rodriguez, Benzel and Clevenger129,Reference Kolpek, Ott and Record142,Reference Rodriguez, Clevenger and Osler143) . While a negative nitrogen balance does occur, this is obligatory. While the underlying mechanism contributing to a negative nitrogen balance following SCI remains poorly understood(Reference Kolpek, Ott and Record142–Reference Tator, van der Jagt and Malkin145), efforts made to shift the obligatory loss of nitrogen by increasing energetic intake can lead to overfeeding. Confounding this matter is that several studies and registered dietitians use correction factors to increase energetic intake. Kaufman et al. (Reference Kaufman, Rowlands and Stein137) and Barco et al. (Reference Barco, Smith and Peerless93) used an activity factor of 1·1. However, a stress factor of 1·2 to 1·75 is routinely used in in the literature(Reference Cox, Weiss and Posuniak89,Reference Rodriguez, Benzel and Clevenger129,Reference Kaufman, Rowlands and Stein137,Reference Rodriguez, Clevenger and Osler143) . Rodriguez et al. (Reference Rodriguez, Benzel and Clevenger129) examined the Harris-Benedict equation with an activity factor of 1·2 and an injury factor of 1·6 and identified that the equation overestimated energetic requirements in twelve persons with acute SCI. The same authors reported that establishing nutritional management upon serial indirect calorimetry measurements with higher stress and activity factors result in overfeeding(Reference Rodriguez, Benzel and Clevenger129). Kearns et al. (Reference Kearns, Thompson and Werner119) compared measured RMR with the Harris-Benedict equation in five individuals with tetraplegia and reported that the use of the equation leads to an overfeeding by nearly 70 %. While the time frame of the investigation relative to SCI was not specified, the authors suggested administering 80 % of predicted energetic needs(Reference Kearns, Thompson and Werner119).
The 2008 Paralyzed Veterans of America (PVA) Early Acute Management in Adults with Spinal Cord Injury: A Clinical Practice Guideline for Health-Care Professions state ‘Provide appropriate nutrition when resuscitation has been completed and there is no evidence of ongoing (spinal) shock or hypoperfusion’. The PVA recommendations do endorse the determination of energetic requirements for nutritional support using a 30-min energy expenditure measurement by indirect calorimetry. These guidelines for acute SCI do not define or provide a reference for what ‘appropriate nutrition’ entails, and hospitals and inpatient rehabilitation facilities do not use or often have access to metabolic carts, and insurance plans do not cover the cost of indirect calorimetry. Similarly, the Academy of Nutrition and Dietetics (AND) recommends the use of indirect calorimetry during the acute phase of SCI. They state ‘actual energy needs are at least 10 % below predicted needs(140)’. But the AND recommends in the absence of indirect calorimetry to use the Harris-Benedict formula using admission weight and an injury factor of 1·1 and an activity factor of 1·2(140). This type of prediction method overestimates TDEE and subsequently energetic intake in persons with SCI, thereby leading to overfeeding(Reference Rodriguez, Benzel and Clevenger129,Reference Rodriguez, Clevenger and Osler143) . Persons with SCI remain in an obligatory negative nitrogen balance, but providing excess energy content should be avoided. Overfeeding carries unique complications, such as hyperglycaemia, hypercapnia, hypertriacylglycerolaemia, uremia and obesity(Reference Nash, Groah and Gater8,Reference Todd, Gonzalez and Turner146) .
With regard to chronic SCI, the PVA Consortium for Spinal Cord Medicine recently assembled an expert panel to compile the Clinical Practice Guidelines on Identification and Management of Cardiometabolic Risk after SCI (PVA guidelines; Table 7; Fig. 2)(Reference Nash, Groah and Gater10). The inaugural guidelines recommended when establishing energetic targets, all persons with chronic SCI should undergo an energetic assessment using indirect calorimetry to estimate energy expenditure and assess energy needs(Reference Nash, Groah and Gater10). Given indirect calorimetry is used to measure resting and basal metabolism, an important consideration is how to determine TDEE. In 2019, Farkas et al. (Reference Farkas, Gorgey and Dolbow9) developed a novel SCI-specific correction factor of 1·15 to estimate TDEE from BMR (or RMR) using 2·7 ml of oxygen/kg of body weight/min(Reference Collins, Gater and Kiratli80), a MET (metabolic equivalent of task) for SCI. The SCI-specific TDEE prediction equation requires validation against the gold standard respiratory chamber but provides promise. It is a novel method to estimate TDEE, and to accurately determine energetic needs in chronic SCI.
Table 7. Practical dietary recommendations with example foods to consume and avoid for persons with SCI
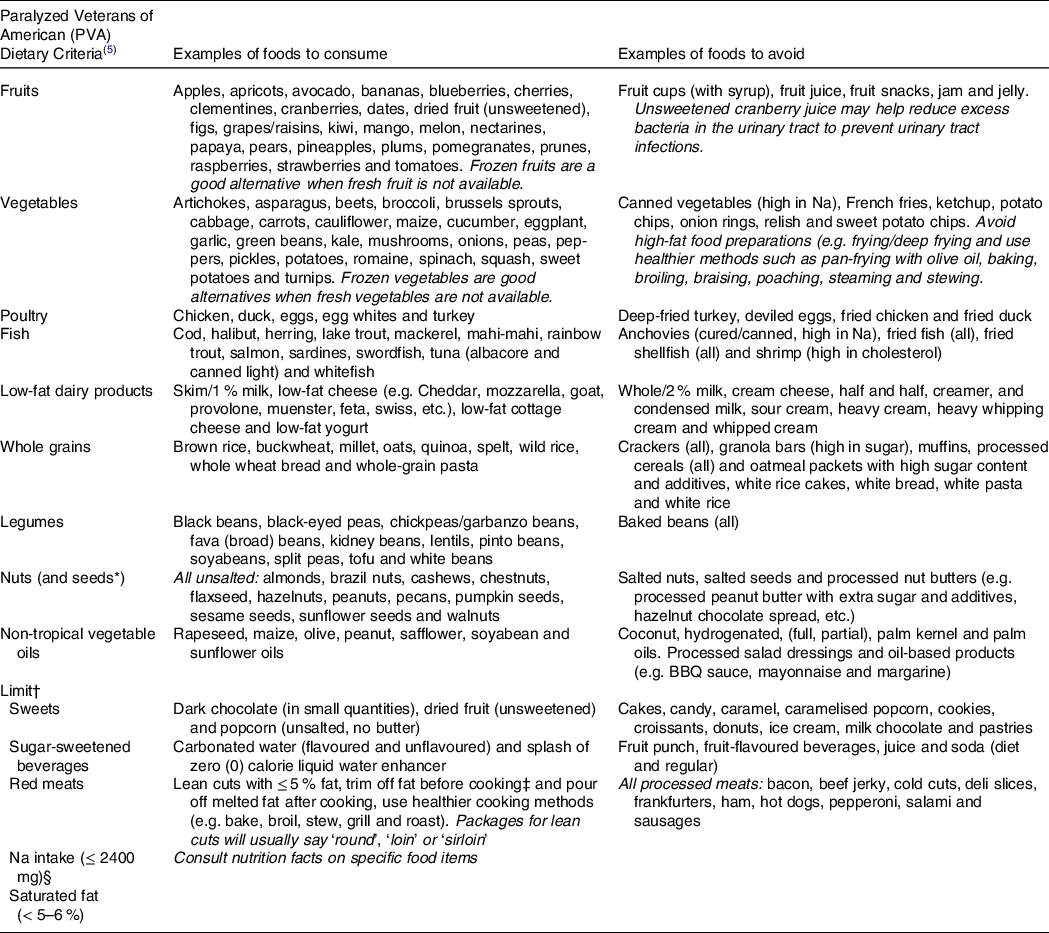
* The PVA guidelines do not mention seeds; however, the authors are including seeds in the nut category.
† Limit to special occasions (i.e., birthdays, weddings, holidays, etc.)
‡ For persons with limited upper extremity function, ask the butcher to trim the fat at the supermarket.
§ For persons with hypertension, although the authors recommend adopting ≤ 2400 mg of Na for all individuals regardless of hypertension status given the elevated consumption of Na-dense foods reported in the literature.

Fig. 2. Sequential dietary recommendations for persons with a spinal cord injury (SCI). First, BMR or RMR should be annually measured with indirect calorimetry or estimated using SCI-specific predictions equations (Nightingale and Gorgey(Reference Nightingale and Gorgey116), Chun et al. (Reference Chun, Kim and Shin105) or Buchholz et al. (Reference Buchholz, McGillivray and Pencharz79)) when indirect calorimetry is unavailable. Second, total daily energy expenditure should be estimated as the product of BMR or RMR and 1·15 for persons with SCI using the Farkas et al. (Reference Farkas, Gorgey and Dolbow9) equation. Third, a registered dietician should oversee a healthy dietary pattern following the Clinical Practice Guidelines on Identification and Management of Cardiometabolic Risk after SCI and additional recommendations provided in this review(Reference Nash, Groah and Gater10).
Across the chronic SCI literature, energetic intake ranges from 1212 to 2732 kcal/d (Table 5)(Reference Farkas, Gorgey and Dolbow9,Reference Monroe, Tataranni and Pratley34,Reference Gorgey, Caudill and Sistrun84,Reference Gorgey, Khalil and Gill86,Reference Liu, Spungen and Fink92,Reference Gorgey, Mather and Cupp108,Reference Gorgey, Martin and Metz109,Reference Nightingale, Williams and Thompson115,Reference Perret and Stoffel-Kurt117,Reference Mollinger, Spurr and el Ghatit130,Reference Groah, Nash and Ljungberg135,Reference Sabour, Soltani and Latifi141,Reference Allison, Beaudry and Thomas147–Reference Barboriak, Rooney and El Ghatit157) , seemingly appearing to be in energy balance when evaluating TDEE (1332 to 2728 kcal/d). At face value, an energy balance does not appear to be congruent with the reported high rates of obesity(Reference Nash, Groah and Gater8,Reference Farkas, Gorgey and Dolbow9,Reference Gater, Farkas and Berg11,Reference Gater, Farkas and Dolbow29,Reference Farkas, Gorgey and Dolbow60) . However, in a recent meta-analysis by Farkas et al. (Reference Farkas, Pitot and Berg19), the authors reported in a sample of 606 persons with chronic SCI, a pooled energetic intake of 1876 kcal/d and a pooled RMR of 1492 kcal/d. Estimating a TDEE of 1716 kcal/d (using RMR × 1·15(Reference Farkas, Gorgey and Dolbow9)), there is a positive energy balance of over 150 kcal/d. This is further supported by the additional work by Farkas and colleagues(Reference Farkas, Gorgey and Dolbow9). The authors reported a greater energetic intake in persons with tetraplegia compared with paraplegia when adjusting energy intake by body weight, thereby accounting for body composition that is significantly different by injury level(Reference Farkas, Gorgey and Dolbow9,Reference Gorgey and Gater107,Reference Inskip, Plunet and Ramer158,Reference Spungen, Adkins and Stewart159) . The authors concluded that their findings may explain why persons with tetraplegia had significantly great percentage body fat relative to paraplegia(Reference Farkas, Gorgey and Dolbow9). Collectively, these data provide support that persons with chronic SCI may overconsume relative to their need, and this may contribute to the high rates of neurogenic obesity. However, the findings are subject to how energetic intake was operationalised.
While dietary recalls, dietary diaries/records/logs and food frequency questionnaire (FFQ) have demonstrated a strong agreement amongst themselves, research has identified systematic misreporting errors for all of the self-reported dietary instruments(Reference Ravelli and Schoeller160). Most of the literature in both acute and chronic SCI describe using dietary assessments without indicating self-report or whether a registered dietitian administered or reviewed the instrument (Table 5). In fact, 40 % of the investigators specify the use of self-report dietary assessment techniques, whereas 11 % and 9 % indicated measuring the food consumed off the plate or if a registered dietitian performed the assessment, respectively (the remaining studies provided insufficient detail to clearly determine assessment methods). It is well established that dietary assessment methods underreport true energetic consumption in persons without SCI(Reference Ravelli and Schoeller160,Reference Subar, Freedman and Tooze161) , and a similar phenomenon is likely present in the population with SCI(Reference Farkas, Gorgey and Dolbow9,Reference Gorgey, Caudill and Sistrun84) . Moreover, self-report after SCI becomes a challenge, especially with higher levels of injury. Persons with tetraplegia may have difficulty writing down, especially in detail, intake data and may limit, and in some cases omit, what they ate or drank on their assessment instruments. Portion size, food preparation and cooking details may be omitted and are details that can greatly influence the energy content of food. Family members or caregiver(s) may introduce a source of error by recording their food. Accordingly, it is likely that, as with the population without SCI(Reference Subar, Freedman and Tooze161), energetic intake is being underreported. Future large-scale studies with more stringent dietary assessment and testing methods are needed to examine the energetic need relative energy expenditure after SCI.
Macronutrient intake
Macronutrients are dietary constituents that provide energy. They include protein, carbohydrates, fats and alcohol. Although alcohol is considered a macronutrient and provides energy, it is not needed for survival.
Protein
Proteins (4 kcal/g) are considered the most abundant macronutrient; they are composed of amino acids, of which nine are essential and cannot be synthesised by the body but must be acquired in the diet. The quality of dietary protein is characterised by the protein’s digestibility and its amino acid profile in relation to requirements as determined by repair, maintenance and growth(12). Several studies report protein ingestion in persons with SCI is within or exceeds recommended daily values for the population without SCI (Tables 5 and 6)(Reference Farkas, Pitot and Berg19,Reference Gorgey, Mather and Cupp108,Reference Nightingale, Williams and Thompson115,Reference Perret and Stoffel-Kurt117,Reference Groah, Nash and Ljungberg135,Reference Iyer, Beck and Walton136,Reference Silveira, Winter and Clark150–Reference Sabour, Nazari and Latifi154,Reference Levine, Nash and Green162–Reference Beal, Gorgey and Moore167) . Approximately 15 to 19 % of the total daily energy intake came from protein for persons with SCI(Reference Gorgey, Mather and Cupp108,Reference Nightingale, Williams and Thompson115,Reference Perret and Stoffel-Kurt117,Reference Allison, Beaudry and Thomas147,Reference Abilmona and Gorgey148,Reference Edwards, Bugaresti and Buchholz151,Reference Lieberman, Goff and Hammond164,Reference Tomey, Chen and Wang165) . In persons with chronic SCI, Farkas et al. (Reference Farkas, Pitot and Berg19) reported consumption of 319 kcal/d of dietary protein surpassed the DGA recommendation of 184 to 224 kcal/d, representing 17 % of their total daily intake, even though fat-free (protein) body mass is markedly reduced. The value reported by Farkas et al. (Reference Farkas, Pitot and Berg19) also exceeded the PHE guidelines on protein consumption and was below recommendations by ADG (Table 6). For most individuals with or without SCI, it is not uncommon to meet or exceed total protein recommendations. The sources of dietary protein largely remain unknown. Silveira et al. (Reference Silveira, Winter and Clark150) identified seafood consumption was low in persons with SCI, although Lieberman et al. (Reference Lieberman, Goff and Hammond164) reported more meat and fish/seafood were consumed compared with non-disabled controls. These conflicting findings may result from the location (Houston, TX(Reference Silveira, Winter and Clark150) v. Charlotte, NC(Reference Lieberman, Goff and Hammond164)) and/or the race/ethnicity (racially/ethnically diverse(Reference Silveira, Winter and Clark150) v. Black/White(Reference Lieberman, Goff and Hammond164)) of the participants and requires additional research.
Many individuals with SCI are not meeting the recommendations for specific amino acids(12,Reference Farkas, Pitot and Berg19) . Sabour et al. (Reference Sabour, Nazari and Latifi154) reported that lysine, leucine, valine and isoleucine were the major constituents of total protein intake in persons with SCI, while arginine, alanine and aspartic acid had the lowest daily intake. Groah et al. (Reference Groah, Nash and Ljungberg135) demonstrated that amino acid intake after SCI approached, or met, DGA dietary recommendations except for lysine, leucine, threonine, methionine and cysteine. The same authors noted that men with paraplegia consumed a greater amount of every amino acid compared with men with tetraplegia(Reference Groah, Nash and Ljungberg135). This evidence suggests that while protein consumption remains high among persons with SCI, some may still be missing key essential amino acids that can result in malnutrition and health consequences. Moreover, these essential amino acids are necessary for vital functions such as protein synthesis and tissue repair, which is particularly important in this population group that is prone to pressure injuries.
Pressure injuries after SCI precipitously deplete the limited protein stores as the body attempts to heal the wound, generating a rapid transition to malnutrition. This occurs in the presence of already markedly diminished protein reserves (i.e.. skeletal muscle mass). The AND recommends that in the presence of a pressure injury for persons with SCI, albumin and prealbumin laboratory values should be measured(140). Prealbumin (also known as transthyretin) and albumin have traditionally been utilised as biomarkers of protein nutrition and nutritional status, respectively. A 2012 consensus statement from the AND and the American Society for Parenteral and Enteral Nutrition discouraged the use of prealbumin and albumin as ‘sole’ indicators of undernutrition due to their susceptibility to systemic inflammation(Reference White, Guenter and Jensen168). In their place, the panel recommended the identification of two or more of the following six characteristics for a malnutrition diagnosis: insufficient energetic intake, weight loss, loss of muscle mass, loss of subcutaneous tissue, localised or generalised fluid accumulation that may sometimes mask weight loss, and diminished functional status as measured by handgrip strength(Reference White, Guenter and Jensen168). For persons with SCI, lower extremity fluid accumulation is common and due to paralysis, handgrip strength cannot be measured in tetraplegia, thus potentially limiting their utility. The Global Leadership Initiative on Malnutrition recently published guidelines that favoured reinstituting the application of prealbumin as a contributing element to monitor undernutrition in conjunction with C-reactive protein under 15 mg/dl (denoting asymptomatic infection), as prealbumin levels above that are uninterpretable(Reference Delliere and Cynober169–Reference Keller171). This is supported by recent evidence that suggests prealbumin can supplement other markers such as anthropometrics and clinical history to assess and monitor undernutrition(Reference Ranasinghe, Biswas and Vincent172). In a retrospective chart review of 170 SCI patients with pressure injuries, Lussi et al. (Reference Lussi, Frotzler and Jenny173) reported 15·3 % and 34 % of the patients only had pathologic laboratory values of prealbumin and albumin, respectively. Poor protein blood levels, however, were observed in 41 % of the patients(Reference Lussi, Frotzler and Jenny173), suggesting protein blood levels may be a promising measure to assess protein health and pressure injury risk after SCI. Because the use of prealbumin and albumin remain controversial, laboratory examinations, nutritional assessments and anthropometric measures are collectively needed to detect, correct, and treat pressure injuries and protein nutritional deficits after SCI.
Carbohydrate
Carbohydrates (4 kcal/g) are organic compounds in the form of sugars, starches and fibres. The energy source is rich in simple or complex carbohydrates(12). Simple carbohydrates are largely consumed by persons with SCI(Reference Iyer, Beck and Walton136,Reference Sabour, Javidan and Vafa153) , while added sugars surpass consumption by non-disabled individuals and the DGA, PHE, IOM, and ADG recommendations(Reference Silveira, Winter and Clark150). Moreover, simple carbohydrates and added sugars (i.e., processed foods) have a high glycaemic index, meaning consumption of these foods cause a rapid hyperglycemia and insulin release that is difficult to control in persons with SCI and prediabetes and type 2 diabetes mellitus.
Several studies identified that about half of the energy content consumed by persons with SCI were from carbohydrates (Table 5)(Reference Gorgey, Mather and Cupp108,Reference Iyer, Beck and Walton136,Reference Allison, Beaudry and Thomas147,Reference Silveira, Winter and Clark150–Reference Walters, Buchholz and Martin Ginis152,Reference Lieberman, Goff and Hammond164,Reference Tomey, Chen and Wang165) , whereas Nightingale et al. (Reference Nightingale, Williams and Thompson115) identified 44 % of the daily energy content came from carbohydrates, respectively. Perret and Stoffel-Kurt(Reference Perret and Stoffel-Kurt117) observed that persons with acute SCI consume a greater percentage of carbohydrates compared with persons with chronic SCI(Reference Perret and Stoffel-Kurt117). Iyer et al. (Reference Iyer, Beck and Walton136) and Sabour et al. (Reference Sabour, Javidan and Vafa153) reported higher consumption of carbohydrates in men compared with women with SCI. The latter authors also identified that time since injury, education, and sex were significant predictors for carbohydrate intake in persons with SCI(Reference Sabour, Javidan and Vafa153). Farkas et al. (Reference Farkas, Pitot and Berg19) calculated the average carbohydrate intake for persons with long-standing SCI as 969 kcal/d, a value that exceeds the DGA of 520 kcal/d. This equates to over 50 % of ingested energy content coming from carbohydrates(Reference Farkas, Pitot and Berg19). The DGA, IOM and ADG recommend that carbohydrates make up 45 to 65 % of an individual’s total daily energy content when consuming a 2000 kcal/d diet. Although, on average, persons with SCI are consuming less than 2000 kcal/d according to Farkas et al. (Reference Farkas, Pitot and Berg19), suggesting 45 to 65 % of the total daily energy content coming from carbohydrates should be reduced for persons with SCI.
Carbohydrate consumption should come from complex carbohydrates with a low glycaemic index, such as whole grains. It is well established that whole grains inherently control blood sugar and increase micronutrients, satiation/satiety and fibre consumption(Reference Della Pepa, Vetrani and Vitale174). For persons with SCI, data supporting whole-grain consumption are limited. Silveira et al. (Reference Silveira, Winter and Clark150) reported that whole grains made up 15 % of the total grains in the diets of individuals with SCI compared with 19 % in non-disabled controls. Similarly, Lieberman et al. (Reference Lieberman, Goff and Hammond164) reported significantly lower daily servings of whole grains in SCI compared (1·20) with non-disabled controls (2·44); however, refined grains did not significantly differ (SCI: 5·42 ± 3·45 v. controls: 6·44 ± 6·45), likely to a large variance in the control group. The same authors also showed that 9 % of persons with SCI adhered to consuming ≥ 3 ounces of whole grains compared with 21 % of age- and sex-matched non-disabled controlled(Reference Lieberman, Goff and Hammond164). The factors contributing to reduced whole-grain consumption in persons with SCI warrant further investigation because of their cardioprotective effects against the risk of heart disease, type 2 diabetes mellitus and obesity, co-morbidities with a high occurrence in the SCI population.
Fat
Fats (9 kcal/g) are a type of lipid and a dense source of energy. Fats are combinations of SFA and unsaturated fats, such as MUFA, PUFA, n-3PUFA, n-6PUFA and trans-fatty acids(12). Fats serve various functions throughout the body, including vitamin transport, organ insulation, maintenance of body temperature, formation of the lipid bilayer of a cell and energy storage.
An abundance of evidence indicates that individuals with SCI ingest amounts of dietary fat that are within or surpass DGA, PHE, ADG and IOM recommendations(Reference Farkas, Gorgey and Dolbow9,12,Reference Farkas, Pitot and Berg19,Reference Perret and Stoffel-Kurt117,Reference Aquilani, Boschi and Contardi120,Reference Groah, Nash and Ljungberg135,Reference Iyer, Beck and Walton136,Reference Sabour, Soltani and Latifi141,Reference Silveira, Winter and Clark150,Reference Edwards, Bugaresti and Buchholz151,Reference Sabour, Javidan and Vafa153–Reference Sabour, Norouzi-Javidan and Soltani155,Reference Levine, Nash and Green162,Reference Tomey, Chen and Wang165,Reference Sabour, Larijani and Vafa175,Reference Moussavi, Ribas-Cardus and Rintala176) , indicating after carbohydrate consumption, a substantial number of energy content are derived from dietary fat (Table 6). Approximately 34 % to 40 % of daily energy comes from fat in persons with chronic SCI (Table 5)(Reference Gorgey, Mather and Cupp108,Reference Nightingale, Williams and Thompson115,Reference Allison, Beaudry and Thomas147,Reference Abilmona and Gorgey148,Reference Silveira, Winter and Clark150,Reference Edwards, Bugaresti and Buchholz151,Reference Levine, Nash and Green162,Reference Lieberman, Goff and Hammond164,Reference Tomey, Chen and Wang165,Reference Moussavi, Ribas-Cardus and Rintala176) where the upper range is characteristic of a typical US diet(Reference Levine, Nash and Green162). In the meta-analysis by Farkas et al. (Reference Farkas, Pitot and Berg19), the authors reported that fat intake made up 35 % (663 kcal/d) of the total energetic intake for persons with chronic SCI. The authors noted that fat intake was within the DGA; however, the analysis did not account for the DGA sex- and age-specific ranges for fat intake because of limited power(Reference Farkas, Pitot and Berg19). Therefore, these findings should be interpreted with caution under the notion that age and sex were not considered.
High-fat diets often induce greater food intake and weight gain(Reference Golay and Bobbioni177), and high saturated fat consumption negatively influences cardiometabolic health and chronic disease risk(Reference Willett, Koplan and Nugent178). Intake of saturated fat in persons with SCI is close to the limit or exceeds the recommended daily amount of < 10 to 11 % of total energy content according to DGA, IOM, WHO, PHE and ADG (Table 6)(Reference Groah, Nash and Ljungberg135,Reference Iyer, Beck and Walton136,Reference Allison, Beaudry and Thomas147,Reference Chen, Henson and Jackson149,Reference Silveira, Winter and Clark150,Reference Sabour, Javidan and Vafa153,Reference Sabour, Norouzi-Javidan and Soltani155,Reference Levine, Nash and Green162,Reference Tomey, Chen and Wang165) . Tomey et al. (Reference Tomey, Chen and Wang165), Moussavi et al. (Reference Moussavi, Ribas-Cardus and Rintala176) and Groah et al. (Reference Groah, Nash and Ljungberg135) reported that saturated fat intake in persons with SCI is higher than the recommended maximum of 10 % of total daily energy content by the USDA’s Food Guide Pyramid, 10 % by the National Cholesterol Education Program and 7 % by the AHA, respectively. The DGA, WHO, PHE and ADG recommend limiting saturated fat to < 10 % to 11 % of daily energetic intake, AHA to 7 %, and IOM to as low as possible (Table 6). The PVA guidelines(Reference Nash, Groah and Gater10) limit saturated fat to 5 to 6 % of total energetic intake. Persons with SCI exceed recommended values of saturated fat, despite an overall reduced energetic intake(Reference Silveira, Winter and Clark150). The total energy requirements after SCI are less than a non-disabled individual’s, and dietary consumption of saturated fats should mimic the reduced energetic intake, by limiting foods discussed in Table 7.
The DGA, AHA, WHO and ADG recommend replacing saturated fats with unsaturated fatty acids as they provide a cardioprotective effect (Table 6)(12). n-3 and n-6 PUFA are essential in the diet because they cannot be synthesised by humans. Allison et al. (Reference Allison, Beaudry and Thomas147) reported MUFA consumption did not change following an anti-inflammatory diet, while n-3 and n-6 fatty acids increased and decreased, respectively. Farkas et al. (Reference Farkas, Pitot and Berg19) showed greater consumption of MUFA and PUFA in persons with chronic tetraplegia compared with paraplegia; however, this was not a significant finding. Sabour et al. (Reference Sabour, Javidan and Vafa153) examined dietary fats by injury completeness and noted persons with incomplete injuries consumed more MUFA than those with a complete SCI. According to Silveira et al. (Reference Silveira, Winter and Clark150), MUFA were within healthy ranges, while Groah et al. (Reference Groah, Nash and Ljungberg135) reported that men and women with paraplegia had lower than the DGA recommended adequate intake of n-6 linoleic acid. According to Sabour et al. (Reference Sabour, Norouzi-Javidan and Soltani155) linoleic acid consumption exceeded recommended values in persons with SCI. Iyer et al. (Reference Iyer, Beck and Walton136) and Groah et al. (Reference Groah, Nash and Ljungberg135) also observed that women with SCI exceeded or approached the recommended intake of n-3 linolenic acid, while men with SCI had lower than the recommended intake(Reference Groah, Nash and Ljungberg135). The latter finding mirrors the results reported by Sabour et al. (Reference Sabour, Norouzi-Javidan and Soltani155) for men and women with SCI. Silveira and colleagues(Reference Silveira, Winter and Clark150) noted that linoleic and linolenic acids were within normal ranges according to IOM recommendations. These data indicate additional research is needed on MUFA and PUFA and their health-promoting influence after SCI.
Alcohol
Consumed alcohol (7 kcal/g) is known as ethanol and is not a vital macronutrient(12). Ethanol is passively absorbed in the digestive system and metabolised mainly in the liver, although some are also metabolised in the stomach(Reference Cederbaum179). Allison et al. (Reference Allison, Beaudry and Thomas147) and Nightingale et al. (Reference Nightingale, Williams and Thompson115) reported that 1·4 % and 3 % of the daily energetic intake came from alcohol in persons with SCI, respectively. Groah and colleagues(Reference Groah, Nash and Ljungberg135) showed that mean alcohol consumption was overall low (< 10 g/d) among persons with SCI but greater for men than for women with SCI (6·43 v. 2·24 g/d). Another study reported persons with SCI did not consume any alcohol at home(Reference Iyer, Beck and Walton136). Contrary to these data, other studies report high alcohol consumption in persons with SCI(Reference Garrison, Clifford and Gleason180–Reference Saunders and Krause183). Study participants with SCI are likely to underreport their true alcohol intake on dietary recalls/logs given the stigma that is often related to alcohol consumption and its effects on body weight and physical and mental health(Reference Tate, Forchheimer and Krause182). Asking if individuals are current drinkers (i.e. how much they drank in the last month) rather than how much they drink may be a better indicator of alcohol intake in this population.
Fruits and vegetables
Five studies reveal the consumption of fruits and vegetables among individuals with SCI is below the recommended intake according to DGA, ADG, PHE and IOM guidelines(Reference Iyer, Beck and Walton136,Reference Silveira, Winter and Clark150,Reference Lieberman, Goff and Hammond164,Reference Tomey, Chen and Wang165,Reference Knight, Buchholz and Martin Ginis184) . These data coincide with the reduced consumption of fruits and vegetables in the population without SCI(12–14). Silveria et al. (Reference Silveira, Winter and Clark150) recently revealed that consumption of fruits and vegetables were not only below DGA recommended values, but below the persons without SCI. Lieberman et al. (Reference Lieberman, Goff and Hammond164) and Tomey(Reference Tomey, Chen and Wang165) demonstrated similar results. Knight et al. (Reference Knight, Buchholz and Martin Ginis184) observed that fruit and vegetable consumption was greatest among persons with SCI with a high activity level of about 30 min/d compared with lower activity levels. However, the authors did not differentiate between fruit and vegetable intake(Reference Knight, Buchholz and Martin Ginis184). Interestingly, 80% of the studies that reported fruit and vegetable intake reported vegetable intake was greater than fruit intake(Reference Silveira, Winter and Clark150,Reference Lieberman, Goff and Hammond164,Reference Tomey, Chen and Wang165) . This finding is intriguing given many fruits contain a high amount of simple carbohydrates (i.e. monosaccharides and disaccharides), and as reported by the present review, persons with SCI primarily consume simple carbohydrates v. complex carbohydrates(Reference Sabour, Javidan and Vafa153).
Fibre
Fibre (about 1·5–2·5 kcal/g) consists of soluble and insoluble complex carbohydrates and lignin that are intrinsic to, and intact in, plants, such as whole grains, vegetables and fruits. Soluble fibre undergoes bacterial degradation in the large intestine to generate volatile free fatty acids that are then absorbed and used as energy(16). Several studies have identified fibre intake in persons with SCI is low independent of sex and injury characteristics(Reference Farkas, Pitot and Berg19,Reference Perret and Stoffel-Kurt117,Reference Aquilani, Boschi and Contardi120,Reference Groah, Nash and Ljungberg135,Reference Allison, Beaudry and Thomas147,Reference Chen, Henson and Jackson149,Reference Silveira, Winter and Clark150,Reference Walters, Buchholz and Martin Ginis152,Reference Sabour, Javidan and Vafa153,Reference Levine, Nash and Green162,Reference Tomey, Chen and Wang165,Reference Sabour, Larijani and Vafa175) . Iyer et al. (Reference Iyer, Beck and Walton136) was the only study to report a high fibre consumption of 30 to 33 g/d, while all other studies report an average intake of 12 to 22 g/d(Reference Allison, Beaudry and Thomas147,Reference Sabour, Javidan and Vafa153,Reference Levine, Nash and Green162,Reference Tomey, Chen and Wang165,Reference Sabour, Larijani and Vafa175) . Levine et al. (Reference Levine, Nash and Green162) showed that dietary fibre intake in men with SCI was a third less than the average intake in the non-disabled population. Farkas et al. (Reference Farkas, Pitot and Berg19) quantified fibre intake in the population with chronic SCI as 17 g/d, which is below the recommendations of the DGA, PHE, ADG, IOM and AHA (Table 6). Low intakes of dietary fibre are likely due in part to the low intake of vegetables and fruits, and potentially whole grains.
Conversely, high fibre diets after SCI may cause negative consequences on neurogenic bowel and bladder conditions. Diets high in fibre (> 20 g/d) may instigate unfavourable changes in bowel function and bowel care programmes that do not occur in the non-disabled population(140). Cameron et al. (Reference Cameron, Nyulasi and Collier185) reported that high dietary fibre before a bowel movement does not have the same effect on bowel function in motor complete SCI as in non-disabled individuals. Furthermore, fibre consumption that is too high without commensurate fluid intake can lead to constipation with an already decreased bowel motility(Reference Holmes41). The excess fluid intake that is required with high fibre diets may also require additional urethral cathing or lead to bowel/bladder accidents. Consequently, the effects on bowel and bladder care can make high dietary fibre diet recommendations inappropriate for individuals living with SCI(Reference Sciarra, Anna Lucia and Elisabetta186). Therefore, it is important to develop SCI guidelines on fibre that account for their bowel and bladder programmes (e.g., timing), fluid intake, and their reduced energetic needs relative to an individual without an SCI.
Micronutrients
Micronutrients include vitamins and minerals that are required for cellular communication, water and nutrient transport, the structural integrity of bones, wound healing, and acid–base balance(Reference Gater5). Several micronutrient intakes are within inadequate ranges in persons with SCI according to the recommended guidelines established by the DGA, IOM, PHE and ADG (Table 6)(Reference Farkas, Pitot and Berg19,Reference Perret and Stoffel-Kurt117,Reference Groah, Nash and Ljungberg135,Reference Walters, Buchholz and Martin Ginis152,Reference Levine, Nash and Green162,Reference Lieberman, Goff and Hammond164–Reference Krempien and Barr166) . Although others have reported below recommended intake values of vitamins A, B5, B7, C, D and E in individuals with chronic SCI(Reference Perret and Stoffel-Kurt117,Reference Groah, Nash and Ljungberg135,Reference Walters, Buchholz and Martin Ginis152,Reference Doubelt, de Zepetnek and MacDonald163,Reference Tomey, Chen and Wang165,Reference Sabour, Larijani and Vafa175,Reference Javidan, Sabour and Latifi187) , as well as below-recommended intake in the minerals Ca, Mg and K(Reference Perret and Stoffel-Kurt117,Reference Groah, Nash and Ljungberg135,Reference Walters, Buchholz and Martin Ginis152,Reference Levine, Nash and Green162,Reference Doubelt, de Zepetnek and MacDonald163,Reference Sabour, Larijani and Vafa175,Reference Javidan, Sabour and Latifi187) . In the meta-analysis by Farkas et al. (Reference Farkas, Pitot and Berg19), the authors reported below recommended intakes for vitamins A, B5, B7, B9, D and E, and the minerals K and Ca in persons with chronic SCI according to the DGA report. The authors also found excess intake of vitamins B1, B2, B3, B12 and K, and the minerals Cu, P, Zn and Na according to recommendations(Reference Farkas, Pitot and Berg19). Na is one of the most widely studied micronutrients after SCI with an average consumption ranging from 2402 to 4300 mg(Reference Perret and Stoffel-Kurt117,Reference Groah, Nash and Ljungberg135,Reference Silveira, Winter and Clark150,Reference Walters, Buchholz and Martin Ginis152,Reference Levine, Nash and Green162,Reference Tomey, Chen and Wang165,Reference Krempien and Barr166) . PVA guidelines recommend Na consumption ≤ 2400 mg/d for all persons with SCI and hypertension. We argue that the high consumption of Na and the prevalence of hypertension in the population(Reference Nash, Groah and Gater8,Reference Gater, Farkas and Berg11,Reference Gater, Farkas and Dolbow29) necessitate the need to implement a Na intake ≤ 2400 mg/d for all with SCI.
Three studies have evaluated vitamin and mineral supplementation after SCI. Opperman et al. (Reference Opperman, Buchholz and Darlington188) reported that nutritional supplementation was common in individuals with long-standing SCI, but no common characteristics (e.g., sex, LOI, age, education, etc.) distinguished users from non-users. According to the authors, 71 % of the sample reported using supplements at least once, with approximately 51 % being classified as consistent supplement users at least twice across the three time points assessed in the study. Both Opperman et al. (Reference Opperman, Buchholz and Darlington188) and Walters et al. (Reference Walters, Buchholz and Martin Ginis152) reported that participants with SCI consumed a micronutrient supplement in the form of Ca, a multivitamin, or vitamin D. The latter authors also observed vitamin C supplementation in their participants(Reference Walters, Buchholz and Martin Ginis152). Similarly, Wong and colleagues(Reference Wong, Graham and Green189) reported that the three most prescribed supplements for persons with SCI were multivitamins, vitamins B and vitamin D at an SCI centre. The same authors noted that micronutrient supplementation was significantly associated with age, nutrition risk and serum albumin concentration(Reference Wong, Graham and Green189). Ca and vitamin D supplementation are important for bone health given the high prevalence of osteopenia and osteoporosis in persons with SCI(Reference McMillan, Nash and Gater190). Furthermore, vitamins B and C deficiencies are linked to anaemia and impaired wound healing(Reference Bird, Murphy and Ciappio191), both of which are reported at high rates after SCI(Reference Frisbie192,Reference Kruger, Pires and Ngann193) . Multivitamin and mineral supplementation can correct deficiencies, but if there are no deficiencies, they can potentially be harmful. Persons with SCI should be prescribed vitamin and mineral supplements only if specific deficiencies have been detected or to prevent them (such as vitamin D and Ca for bone density), minimising toxicity risk. Health care professionals should place a greater emphasis on following a healthy dietary pattern (described below) to naturally consume vitamins and minerals rather than relying on supplements that may not completely correct deficiencies and carry some risk (e.g., toxicity).
Dietary recommendations after spinal cord injury
Persons with SCI are instructed to adopt a healthy diet(Reference Gorgey, Caudill and Sistrun84,Reference Khalil, Gorgey and Janisko194) . But what is a healthy diet for this population? Evidence-based guidelines to ameliorate the risks of obesity and CMS did not exist for the SCI population until recently. The PVA guidelines are the first comprehensive publication to provide data-driven recommendations on healthy eating for individuals with SCI. When considering the PVA guidelines, one should note the small sample sizes in SCI literature relative to non-disabled research in diet and nutritional status. This inherent delimitation of clinical research targeting a niche patient population restricts the depth of conclusions that can be drawn from a truly evidence-based approach. Therefore, the inaugural iteration of the PVA guideline recommendations corresponds to the several current recommendations for identifying and managing CMS in the non-disabled population. However, the guidelines also factor in the alterations in the body composition and the unique endometabolic physiology that accompany SCI.
The PVA guidelines recommend energetic assessment utilising indirect calorimetry to determine energy expenditure and assess energy needs to implement a heart-healthy nutrition plan focusing on vegetables, fruits, poultry, fish, low-fat dairy products, whole grains, legumes, nuts and non-tropical vegetable oils, while limiting sweets, sugar-sweetened beverages and red meats (Table 7; Fig. 2). The PVA report also limits dietary saturated fat to 5 % to 6 % of the total energetic intake and limits daily Na intake to ≤ 2400 mg for individuals with hypertension (Table 7; Fig. 2)(Reference Nash, Groah and Gater10).
A reduced emphasis should be placed on limiting macronutrients in diets with persons with SCI, but rather focus on providing a healthy dietary pattern (Table 7; Fig. 2). The authors of this review further recommend adopting ≤ 2400 mg of Na for all individuals, regardless of hypertension status given the elevated consumption of Na-dense foods reported in the literature. We also emphasise the importance of lean poultry consisting of a moderate 3 to 4 oz portion, and the consumption of fish two times per week. Vegetables should be consumed between three and four servings per d. They should consist of the five vegetable subgroups (including dark green, red and orange, legumes (beans/peas), starchy, and others). Fruits should favour whole fruits at three least servings per d and significantly limit 100 % fruit juice because of their added/high sugar content and limited fibre content. Emphasis should be placed on low-fat dairy products in the form of milk, yogurt and cheese in small amounts while limiting saturated fat intake below 5–6 %. High-fat, sugary-based sweets and drinks should be replaced with fresh fruit and water, respectively. Flavoured and unflavoured carbonated water and zero energy liquid water enhancers can be used to provide variety and flavour to drinks. Red meat and sweets should be consumed only on special occasions such as birthdays, weddings, holidays, etc. By following the above-referenced dietary patterns, persons with SCI will naturally limit their intake of refined/simple carbohydrates, Na, and saturated fat and increase the consumption of unsaturated fats and fibre. Such dietary patterns will also promote optimal ingestion of micronutrients.
We recommend and recognise the significance of annual dietary assessments (minimally) and nutrition education with a registered dietitian as part of the medical assessment and standard of care for individuals with SCI (Fig. 2). It is our recommendations that in addition to assessing body composition by 3- or 4-compartment modelling(Reference Gater, Farkas and Dolbow29), registered dietitians should: (1) assess resting metabolism through indirect calorimetry, or, when unavailable, assess RMR/BMR with the Nightingale and Gorgey(Reference Nightingale and Gorgey116), Chun et al. (Reference Chun, Kim and Shin105) or Buchholz et al. (Reference Buchholz, McGillivray and Pencharz79) SCI-specific prediction equation (2) calculate TDEE using the prediction equation and correction factor by Farkas et al. (Reference Farkas, Gorgey and Dolbow9) to determine accurate energy needs, (3) encourage adherence to the SCI dietary guidelines detailed above and balanced nutrition (Table 7, Fig. 2) as an overall healthy lifestyle choice, (4) prescribe dietary supplements when specific vitamin and/or mineral deficiencies have been detected or to prevent them when appropriate nutrition/healthy dietary patterns are unsucessful, and (5) explore dietary anomalies specific to SCI, such as avoidance of food groups that may affect bowel/bladder function post-injury. Periodic assessments with the health care team, including a registered dietitian, should be implemented to manage neurogenic obesity, cardiometabolic risk and nutrition status after SCI and allow the individual themselves to take an active role in their overall health.
Future research
Expert advice advocates for pairing diet and physical activity to mitigate neurogenic obesity and reduce the cardiometabolic risk after SCI. However, the interaction of feeding and activity in SCI has only recently begun to be studied and is likely unique in this population. Furthermore, several barriers, such as transportation, overuse injuries, pain, access to facilities, financial restraints, educational knowledge, disability/SCI-specific resources on exercise, and fear of musculoskeletal or integumentary injury, can limit exercise/physical activity engagement. To mitigate barriers to physical exercise and facilitate improvements in overall health, research focused exclusively on dietary intervention may provide a large-scale ‘cure’ to chronic diseases in persons with SCI.
Rather than a nutrient (i.e., low-fat diets) focus, nutrition research is turning its attention to dietary patterns as we consume a whole range of foods and food groups, not just nutrients. Evidence suggests that vegetarian and/or plant-based diets create a negative energy balance and decrease the risk of obesity, diabetes and other chronic health ailments(Reference Tonstad, Butler and Yan195,Reference Fardet and Boirie196) . Fardet and Boirie(Reference Fardet and Boirie196) examined diet and chronic disease risk from over 300 meta-analyses and systematic reviews published in the last 63 years. The authors reported that plant-based foods were more protective against the risk of developing chronic disease compared with animal-based foods, reinforcing fundamental dietary patterns for good health. Among plant-based foods, whole-grain-based foods had a small edge over fruits and vegetables, while for animal-based foods, dairy products overall were considered neutral on health, and fish was considered protective. Red and processed meats were correlated with elevated chronic disease risk as were sugar-sweetened beverages and highly refined low-fibre grains(Reference Fardet and Boirie196). These data were further supported by evidence published by the AHA that diets higher in plant-based foods and lower in animal-based products were associated with a lower risk of cardiovascular morbidity and mortality(Reference Kim, Caulfield and Garcia-Larsen197). Plant-based diets may provide an alternative nutritional plan for cardiometabolic risk factors after SCI and minimise overeating by enhancing satiety relative to their need. Future research should focus on the effects of such diets in the SCI population but also attend to energy balance.
Final considerations
In summary, the loss of metabolically active tissue below the LOI, endometabolic pathophysiology, environmental and physical barriers, and poor dietary choices contribute to the suboptimal dietetics, poor body habitus and cardiometabolic risk in SCI(Reference Nash and Gater198). Individuals with SCI meet several evidence-based recommendations published by authoritative guidelines for non-disabled individuals, although they are likely underestimated or overestimated for this population. These guidelines do not account for SCI-induced changes in body composition(Reference Gorgey, Dolbow and Dolbow199,Reference Gater, Farkas and Taylor200) , reduced metabolic requirements(Reference Farkas, Pitot and Berg19,Reference Monroe, Tataranni and Pratley34,Reference Buchholz, McGillivray and Pencharz79,Reference Bauman, Spungen and Wang87,Reference Buchholz, McGillivray and Pencharz121) , gut dysmotility(Reference Holmes41,Reference Keshavarzian, Barnes and Bruninga201) and sympathetic nervous system dysfunction(Reference Stjernberg, Blumberg and Wallin33,Reference Monroe, Tataranni and Pratley34) and need to be interpreted and used with caution. The PVA guidelines are currently the strongest evidence-based dietary guidelines for the population with SCI and should be followed by persons with SCI and their health care team. Stakeholders and practitioners should have an understanding that the currently limited evidence-based means that guidelines contain might not fully capture the unique nutritional needs of persons with SCI. Therefore, clinical nutritional strategies should also rely on strong inferences from existing studies and use routine monitoring of individual responses to interventions. Because diet-related comorbidities are related to anatomical and physiological changes after SCI, annual nutritional analysis and indirect calorimetry (or, minimally using an SCI-specific BMR/RMR prediction method) with a registered dietitian are encouraged in clinical practice. Future multicentre controlled trials are needed (with less reliance on cross-sectional study design) to collect large data sets on energy expenditure, energy needs and total energetic intake after SCI. These data are needed to help develop comprehensive, evidence-based dietary reference values for nutrients specific for persons with SCI aimed at reducing the secondary complications of the injury.
Acknowledgements
The work is supported by a Postdoctoral Fellowship from the American Association for Anatomy (GJF).
G. J. F. devised the project framework and the conceptual ideas, conducted the literature search, extracted the data, wrote the initial and revised the manuscript, and developed guidelines. D. R. G. assisted with devising the project and revising the manuscript. AS assisted with developing guidelines. G. J. F., A. S. and D. W. M. created the figures. A. S. and D. W. M. assisted with data extraction from the studies and helped to build the tables. E. T., M. S. N. and D. R. G. critically appraised the manuscript. All authors reviewed and approved the final version of the manuscript.
The authors attest that they have no financial or other conflicts of interest.