Low vitamin D status has been associated with decreased bone mineral density (BMD), increased bone turnover markers, falls and bone fracture, immune dysfunction and increasing mortality(Reference Sassi, Tamone and D’Amelio1). Vitamin D deficiency is a global health concern and an epidemic not only in older populations but also in young people as well(Reference Cashman2,Reference Fischer, Haffner-Luntzer and Amling3) .
Vitamin D plays a key role in the regulation of bone metabolism(Reference Pludowski, Takacs and Boyanov4). Vitamin D deficiency can stimulate bone deposition and turnover, which may increase the risk of bone loss, fractures and osteoporosis(Reference Gilsanz, Chalfant and Mo5,Reference Kuchuk, van Schoor and Pluijm6) . Serum 25-hydroxy vitamin D (25(OH)D) level deficiency results in decreased concentrations of ionised Ca, immediately recognised by the parathyroid glands. To maintain Ca homoeostasis, parathyroid hormone (PTH) increases, which consequently results in elevated bone turnover due to PTH interacting with osteoblasts to release Ca(Reference Holick7).
Considering the high prevalence of vitamin D insufficiency and deficiency, vitamin D supplementation and food fortification have been identified as dietary strategies to promote bone homoeostasis(Reference Ghasemifard, Hassanzadeh-Rostami and Abbasi8–Reference Toxqui, Pérez-Granados and Blanco-Rojo11). A meta-analysis conducted in 2017 on twenty randomised clinical trials (RCT) showed that consumption of vitamin-fortified foods can significantly increase serum 25(OH)D and bone mineral density levels and decrease PTH concentrations, without having a beneficial effect on bone turnover markers(Reference Tangestani, Djafarian and Emamat12). In another meta-analysis of forty RCT in 2020, vitamin D fortification resulted in significant reductions in serum levels of PTH and C-terminal telopeptide of type-I collagen (CTX), with no impact on bone mineral density(Reference Emadzadeh, Mehdizadeh and Sharifan13). These two meta-analyses evaluated the effect of vitamin D fortification on bone markers regardless of biological differences in the two sexes.
Given the discrepancies in previous findings and lack of a comprehensive meta-analysis evaluating the effect of vitamin D supplementation or fortification on bone turnover markers in women, the present study was conducted to summarise the evidence of related RCT.
Methods
This systematic review and meta-analysis was developed according to the guidelines of the Cochrane Handbook for Systematic Reviews of Interventions(Reference Cumpston, Li and Page14) and the Preferred Reporting Items for Systematic Reviews and Meta-Analyses report(Reference Moher, Liberati and Tetzlaff15). The study protocol was registered in PROSPERO, an international prospective register of systematic reviews (registration number: CRD42022304099).
Search strategy
A comprehensive literature search was conducted for RCT evaluating the effects of vitamin D supplementation or fortification on bone turnover markers up to January 2023. Electronic databases, including PubMed, Web of Science and Scopus, were searched to find eligible articles. Endnote X8 software was used to screen the references and remove duplicates.
Eligibility criteria
The inclusion criteria for the studies were an open-label or single-, double- or triple-blind RCT comparing interventions that differed only in vitamin D content and measured at least one bone turnover marker in women (aged ≥ 18 years). The control groups could take a placebo, without any intervention or other dosage of vitamin D. Any other combinations of supplements and/or drug treatments were excluded unless administered in both the control and intervention groups. In this meta-analysis, bone resorption factors (including sCTX and urinary type I collagen cross-linked N-telopeptide (uNTX)) and bone formation factors (including osteocalcin (OC), bone alkaline phosphatase (BALP) and procollagen type-1 N propeptide (P1NP)) were considered as the primary outcomes.
Furthermore, non-randomised clinical trials, non-human studies (animal, in vitro and in vivo studies), review articles, observational studies, proceedings, case studies, case reports, grey literature, book chapters, abstracts in conferences, editorials, letters and seminars were excluded. No restriction was placed on the type of vitamin D, administration form (supplementation or fortification) or its dosage, as well as the participants’ baseline vitamin D levels and duration of the intervention.
Screening and data extraction
Studies were first screened for eligibility criteria based on titles and abstracts. The full texts of the included articles were subsequently reviewed for final decision by AA and NZ independently. Any disagreements were resolved by consultation with another reviewer (ShF).
Quantitative data were extracted from eligible articles by two investigators (AA and NZ). Information was collected on study identifications (first author’s name, year of publication and country in which the study was conducted), study design (type of study, duration of intervention, dosage of vitamin D supplements or fortified foods and baseline serum 25(OH)D concentrations), participants (age and comorbidities), intervention and comparator details (sample size for each treatment group, blinding and attrition) and numerical data for the outcomes of sCTX, uNTX, OC, P1NP and BALP. Furthermore, the Get Data Graph Digitizer (http://getdata-graph-digitizer.com/) was used to extract data from the figures when needed. Biochemical methods for bone turnover markers were harmonized, and differences were calculated based on the same unit for each outcome. Data extraction was verified by a third author (NN).
Quality assessment and risk of bias
The overall assessment of evidence was done using the Grading of Recommendation Assessment, Development, and Evaluation (GRADE) system. The GRADE criteria included risk of bias, inconsistency, indirectness, imprecision, publication bias and effect size, and its rating summary was generated using the GRADEpro platform (https://gdt.gradepro.org/).
The risk of bias was evaluated using a Revised Cochrane Risk of Bias tool for randomised trials (RoB2) and ROBVIS-1 framework(Reference Sterne, Savović and Page16). All included studies were assessed for sources of bias in selection, performance, detection, attrition and selective reporting. Each study was classified into one of three categories of bias: low, some concerns or high risk of bias.
Statistical analysis
Mean difference (MD) of change, as the effect size, for bone turnover markers along with the corresponding 95 % CI were calculated for each study. The pooled effect sizes were estimated thorough a random-effects model using restricted maximum likelihood method. The forest plots of the variables were sorted according to the effect size of the studies.
Cochran’s Q test and I2 statistic (I² > 50 % indicates moderate to high heterogeneity) were used to assess the extent of heterogeneity between the included studies. The I² statistic was evaluated as 0–40 % unimportant heterogeneity, 30–60 % moderate heterogeneity and 50–90 % substantial heterogeneity(17).
Subgroup analysis and meta-regression models were carried out to explore the potential sources of heterogeneity. Subgroup analysis was conducted according to the dose of supplementation/fortification (≤ 600 μg/d and > 600 μg/d)(Reference Raymond and Morrow18), intervention duration (≤ 12 weeks and > 12 weeks) and participants’ baseline vitamin D levels (≤ 20 ng/dl and > 20 ng/dl), age of participants (≤ 60 years old and > 60 years old), publication year (< 2010 and ≥ 2010), study sample size (≤ 100 participants and > 100 participants), bone health (healthy postmenopausal women and postmenopausal women with osteoporosis), country region (Asia, Europe, America and South America and Australia) and study quality score (high, some concerns and low risk of bias).
Linear meta-regression model was performed based on dosage of vitamin D administered, duration of intervention, baseline vitamin D level, participants age, publication year and study sample size.
Meta-regression model was also done based on the classifications created for subgroup analysis. In addition, fractional polynomial regression model was applied to investigate non-linear relationships between these factors and the study outcomes.
Sensitivity analysis was also conducted using the leave-one-out method to examine whether the results were robust. Publication bias evaluated using Egger’s linear regression test and visual assessment thorough the funnel plots. In Egger’s test, P value < 0·1 was considered as a significant level(Reference Egger, Smith and Schneider19). All analyses were performed using the STATA software (Stata Crop) version 17, and P value less than 0·05 was considered as statistically significant.
Results
Literature search and study selection
The initial search identified 2424 relevant publications and after removing duplicates (n 678), 1746 records were screened. Based on title and abstract screening, review articles, conference abstracts, animal studies and studies using multi-ingredient nutritional supplements were excluded (n 1626). After assessing the eligibility of full texts, eighty-eight records were excluded due to the gender of study participants (both sexes, n 22; male, n 6), presentation of results as percentage changes (n 16) or lack of eligibility criteria (n 44). Consequently, thirty-two RCT were included in the meta-analysis. The selection process is presented in Fig. 1.
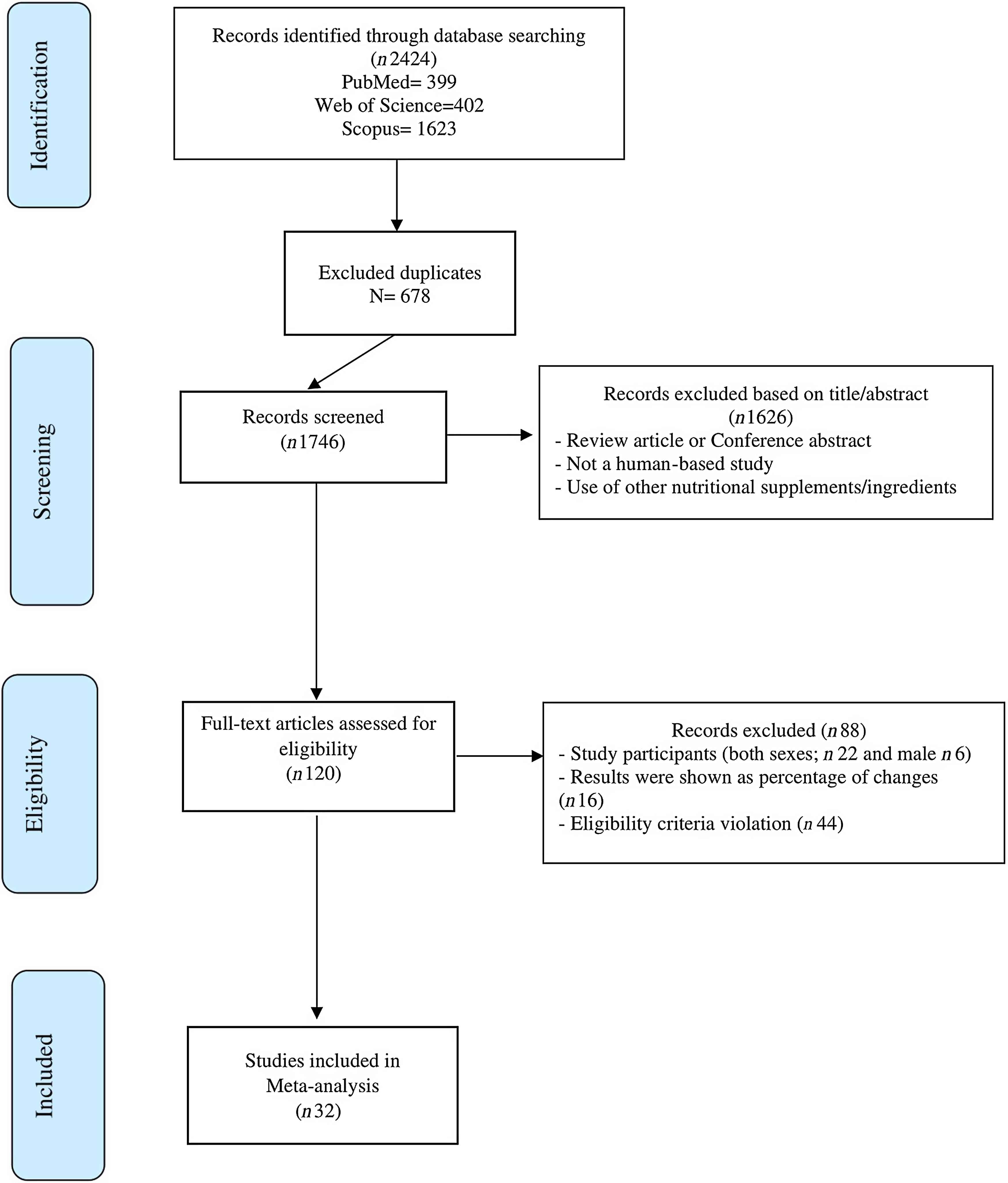
Fig. 1. Preferred Reporting Items for Systematic Reviews and Meta-Analysis (PRISMA) flow chart of the study selection process.
Study characteristics for randomised controlled trials
Detailed characteristics of the thirty-two included studies are shown in Table 1. All studies were performed exclusively on women, with mean ages ranging from 24·75 to 80·35 years. The studies were conducted between 1995 and 2022 in South Korea(Reference Lee, Baek and Chung9,Reference Cho, Chung and Kim20–Reference Son and Chun22) , Poland(Reference Rodziewicz-Flis, Kawa and Flis23), China(Reference Zhang and Wang24–Reference Gao, Zhu and Liu26), Denmark(Reference Grønborg, Tetens and Andersen27,Reference Bislev, Langagergaard Rødbro and Rolighed28) , Japan(Reference Uenishi, Tokiwa and Kato29–Reference Ushiroyama, Okamura and Ikeda37), Brazil(Reference Nahas-Neto, Cangussu and Orsatti38), Finland(Reference Välimäki, Löyttyniemi and Pekkarinen10), Spain(Reference Toxqui, Pérez-Granados and Blanco-Rojo11,Reference Olmos, Hernandez and Llorca39) , UK(Reference Macdonald, Wood and Aucott40,Reference Hunter, Major and Arden41) , USA(Reference Aloia, Dhaliwal and Shieh42), New Zealand(Reference Von Hurst, Stonehouse and Kruger43), Greece(Reference Manios, Moschonis and Panagiotakos44), Australia(Reference Zhu, Bruce and Austin45,Reference Cooper, Clifton-Bligh and Nery46) , Germany(Reference Pfeifer, Begerow and Minne47) and the Netherlands(Reference Ooms, Roos and Bezemer48). The dosage of vitamin D supplementation/fortification varied from 10 μg/d to 200 000 μg/3 months, and the intervention period ranged from 4 to 96 weeks. All studies had a parallel randomised design and were conducted on postmenopausal women with osteopenia/osteoporosis(Reference Lee, Baek and Chung9,Reference Cho, Chung and Kim20–Reference Son and Chun22,Reference Zhang and Wang24,Reference Uenishi, Tokiwa and Kato29–Reference Ushiroyama, Ikeda and Sakai34,Reference Shiraki, Kushida and Yamazaki36,Reference Olmos, Hernandez and Llorca39) , healthy postmenopausal women(Reference Välimäki, Löyttyniemi and Pekkarinen10,Reference Rodziewicz-Flis, Kawa and Flis23,Reference Cheng, Wu and Du25,Reference Gao, Zhu and Liu26,Reference Bislev, Langagergaard Rødbro and Rolighed28,Reference Gorai, Chaki and Taguchi35,Reference Ushiroyama, Okamura and Ikeda37,Reference Nahas-Neto, Cangussu and Orsatti38,Reference Macdonald, Wood and Aucott40–Reference Aloia, Dhaliwal and Shieh42,Reference Manios, Moschonis and Panagiotakos44–Reference Ooms, Roos and Bezemer48) and healthy women aged 18–49 years(Reference Toxqui, Pérez-Granados and Blanco-Rojo11,Reference Grønborg, Tetens and Andersen27,Reference Von Hurst, Stonehouse and Kruger43) . Of the thirty-two included studies, fifteen provided data on sCTX(Reference Lee, Baek and Chung9,Reference Välimäki, Löyttyniemi and Pekkarinen10,Reference Cho, Chung and Kim20,Reference Chung, Koo and Kwon21,Reference Zhang and Wang24–Reference Bislev, Langagergaard Rødbro and Rolighed28,Reference Nahas-Neto, Cangussu and Orsatti38–Reference Macdonald, Wood and Aucott40,Reference Aloia, Dhaliwal and Shieh42–Reference Manios, Moschonis and Panagiotakos44) and four reported uNTX(Reference Toxqui, Pérez-Granados and Blanco-Rojo11,Reference Uenishi, Tokiwa and Kato29,Reference Gorai, Hattori and Tanaka30,Reference Shiraki, Fukuchi and Kiriyama32) . Moreover, changes in OC(Reference Son and Chun22,Reference Rodziewicz-Flis, Kawa and Flis23,Reference Cheng, Wu and Du25–Reference Bislev, Langagergaard Rødbro and Rolighed28,Reference Shiraki, Fukuchi and Kiriyama32–Reference Ushiroyama, Okamura and Ikeda37,Reference Hunter, Major and Arden41,Reference Von Hurst, Stonehouse and Kruger43,Reference Manios, Moschonis and Panagiotakos44,Reference Cooper, Clifton-Bligh and Nery46–Reference Ooms, Roos and Bezemer48) , P1NP(Reference Välimäki, Löyttyniemi and Pekkarinen10,Reference Toxqui, Pérez-Granados and Blanco-Rojo11,Reference Zhang and Wang24,Reference Gao, Zhu and Liu26–Reference Bislev, Langagergaard Rødbro and Rolighed28,Reference Olmos, Hernandez and Llorca39,Reference Macdonald, Wood and Aucott40,Reference Aloia, Dhaliwal and Shieh42,Reference Zhu, Bruce and Austin45) and BALP(Reference Cho, Chung and Kim20,Reference Chung, Koo and Kwon21,Reference Majima, Komatsu and Shimatsu31,Reference Shiraki, Kushida and Yamazaki36,Reference Ooms, Roos and Bezemer48) were extracted from eighteen, ten and five studies, respectively.
Table 1. Characteristics of the studies included in the meta-analysis
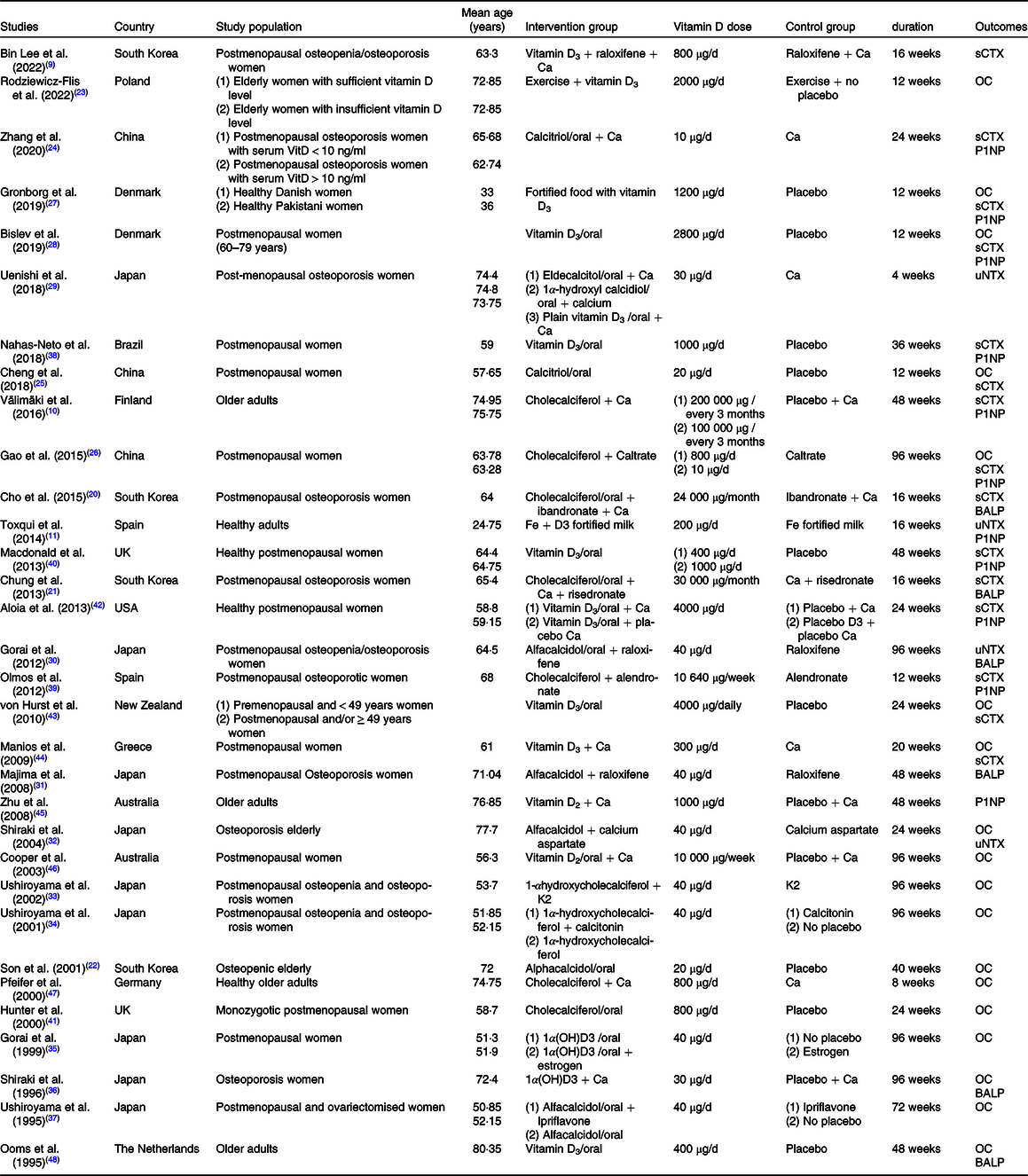
sCTX, serum C-terminal cross-linked telopeptide of type 1 collagen; OC, osteocalcin; P1NP, procollagen type 1 amino-terminal propeptide; uNTX, urinary N-terminal telopeptides of type 1 collagen; BALP, bone alkaline phosphatase.
Meta-Analysis
Effect of vitamin D supplementation/fortification on serum C-terminal telopeptide of type-I collagen
Pooling twenty-two effect sizes indicated that vitamin D consumption had a significant effect on sCTX level (MD: −0·038, 95 % CI −0·057, −0·019, n 22, Table 2, Fig. 2), with considerable heterogeneity (I2 = 64·2 % and P < 0·001).
Table 2. Summary findings of comparison of sCTX between the study treatments
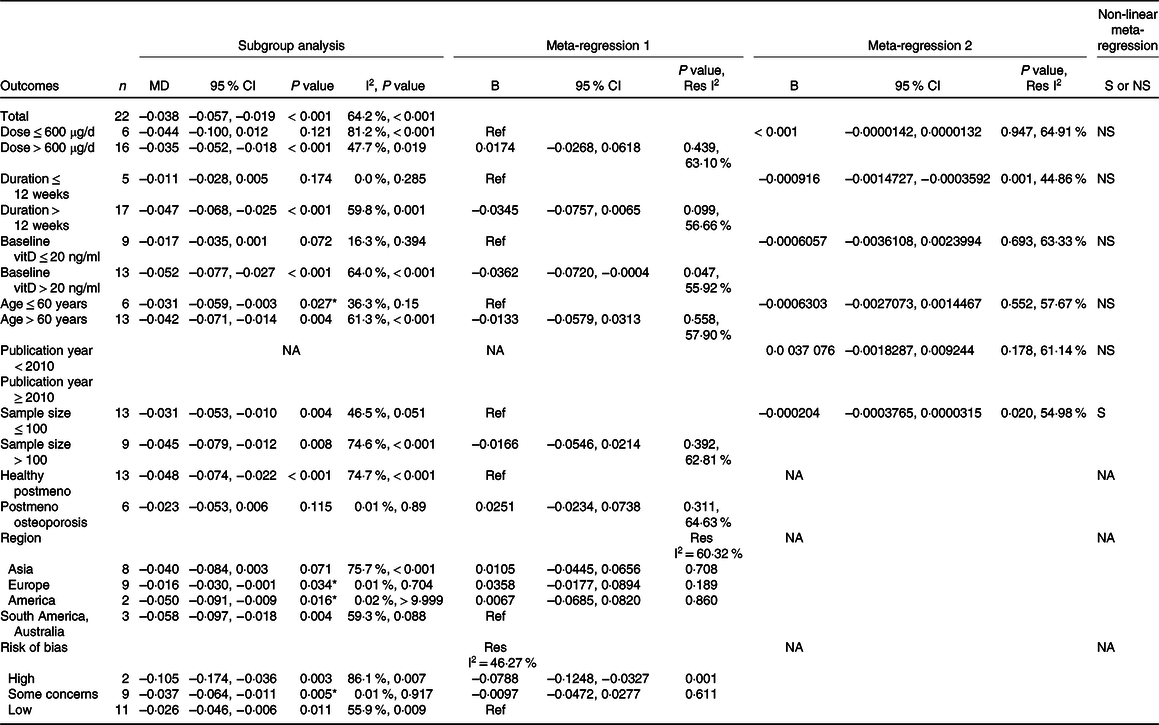
sCTX, serum C-terminal telopeptide of type-I collagen; MD, mean difference; N, number of included interventions; B, beta coefficient reflecting the effect of the subgrouping variable on the pooled effect size; vit, vitamin; postmeno, post-menopausal; Res I2, residual I2; NA, not applicable; S, significant; NS, non-significant. Italic; P values.
Meta-Regression 1: the subgrouping variable was included into the model as a categorised variable. Meta-Regression 2: the subgrouping variable was included into the model as a continuous variable.
* Significance with respect to heterogeneity.
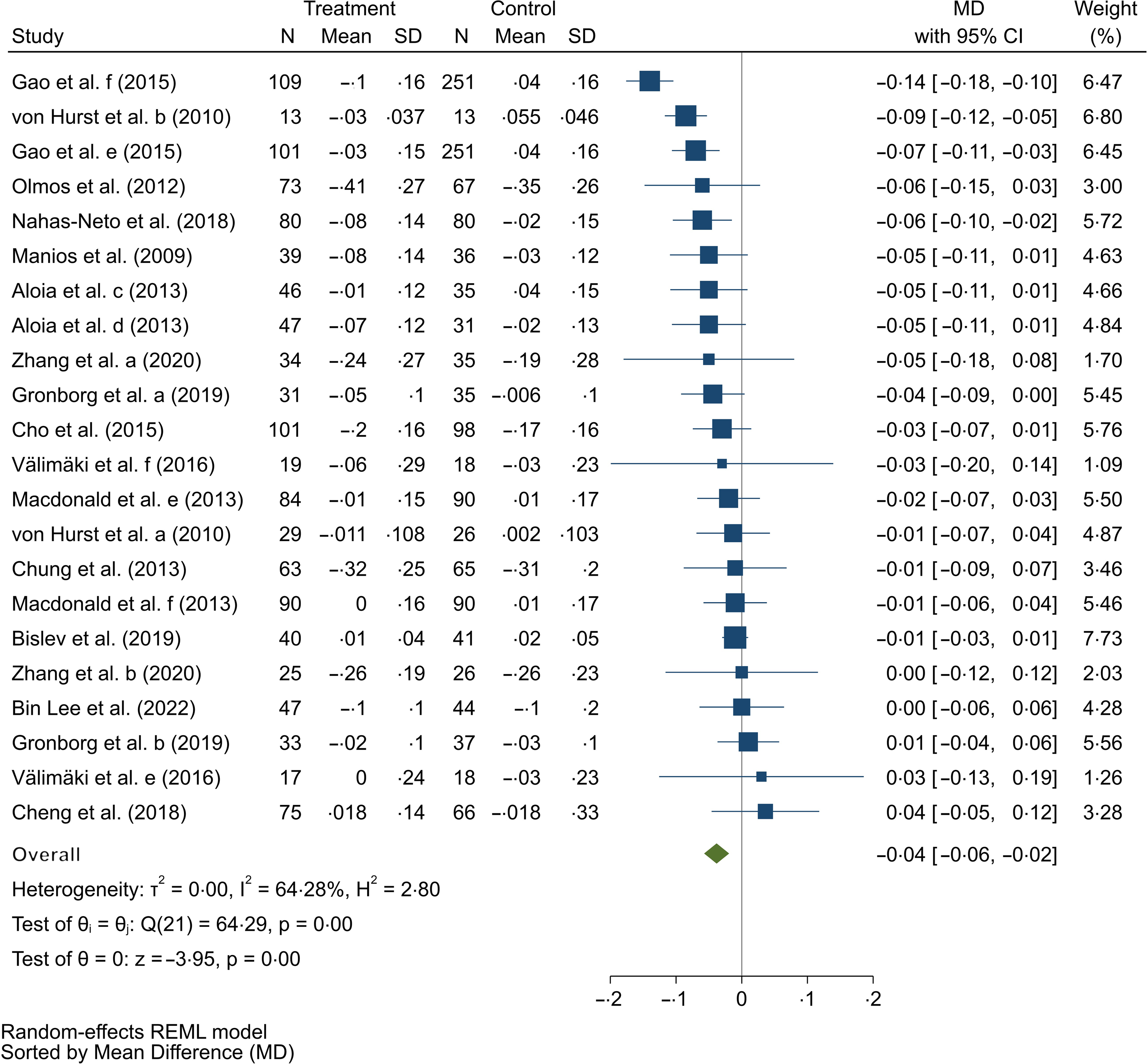
Fig. 2. Forest plot of the randomised clinical trials (RCT) examining the effect of vitamin D supplementation on sCTX. Data have been expressed as mean differences (MD) between intervention and control groups with a 95 % CI. Estimates were pooled using the random effects model. Letters between parentheses represent: a, b: different participant groups; c, d: different intervention/control groups; e, f: different dose of vitamin D.
Based on the findings of subgroup analyses and meta-regression models, duration, baseline level of vitamin D, age, sample size, region and quality of the studies could be the potential sources of heterogeneity. Accordingly, in duration less than 12 weeks, the heterogeneity was very low (I2 = 0 %, P = 0·285), and no significant difference was observed in mean level of sCTX between the treatments. Besides, 10 weeks increase in duration was found to be associated with 0·01 significant decrease in MD of sCTX level, which is in favour of the intervention group (MD: −0·000916, 95 % CI −0·0014727, −0·0003592), P = 0·001, online Supplementary Fig. S11). Regarding the baseline vitamin D level, no significant decrease was observed in sCTX level in the subgroup with low heterogeneity (≤ 20 ng/ml). However, the meta-regression model result showed that reduction in sCTX level was more pronounced in participants with sufficient levels of vitamin D compared with participant with vitamin D deficiency (MD = −0·0362, 95 % CI (–0·0720, −0·0004), P = 0·047). Moreover, subgroup analysis showed that vitamin D significantly reduced sCTX level in participants younger than 60 years (MD = −0·031, 95 % CI (–0·059, −0·003), P = 0·027, online Supplementary Fig. S1) with low heterogeneity (I2 = 36·3 %, P = 0·15). Also, significant differences were observed in studies conducted in Europe (MD = −0·016, 95 % CI (–0·030, −0·001), P = 0·034, online Supplementary Fig. S2) and America (USA) (MD = −0·050, 95 % CI (–0·091, −0·009), P = 0·016, online Supplementary Fig. S2), and studies with some concerns about risk of bias (MD = −0·037, 95 % CI (–0·064, −0·011), P = 0·005, online Supplementary Fig. S3) which all had very low heterogeneity (I2 = 0·01 %, 0·02 %, and 0·01 %, respectively). Furthermore, meta-regression model showed that the MD for sCTX was significantly reduced in studies with high risk of bias compared with studies with low risk of bias (MD = −0·0788, 95 % CI (–0·1248, −0·0327), P = 0·001), confirming that studies with high risk of bias might be a source of heterogeneity. Moreover, linear meta-regression revealed that 100 participants increase in sample size was associated with a significant reduction of 0·02 in MD level of sCTX which is in favour of the intervention group (MD = −0·0002, 95 % CI (–0·0003, −0·00003), P = 0·020, online Supplementary Fig. S12). Finally, the sample size was found to have a non-linear effect on the pooled effect size (online Supplementary Fig. S14).
Effect of vitamin D supplementation/fortification on urinary type I collagen cross-linked N-telopeptide
Results of the analysis on six effect sizes revealed a significant reduction in uNTX following vitamin D supplementation/fortification (MD: −8·138, 95 % CI −12·864, −3·413, n 6, online Supplementary Table S1 and Fig. S4), with low heterogeneity (I2 = 0·00 %, and P = 0·627). Furthermore, a significant reduction in uNTX levels was observed in both study duration subgroups (online Supplementary Fig. S5). Meta-regression analysis did not indicate any effect of possible sources of heterogeneity on the estimated effect sizes.
Effect of vitamin D supplementation/fortification on osteocalcin
Among all studies, vitamin D supplementation or fortification had a significant effect on OC level (MD: −0·614, 95 % CI −1·146, −0·081, n 24, Table 3, Fig. 3), with high heterogeneity (I2 = 79·9 % and P < 0·001).
Table 3. Summary findings of comparison of OC between the study treatments
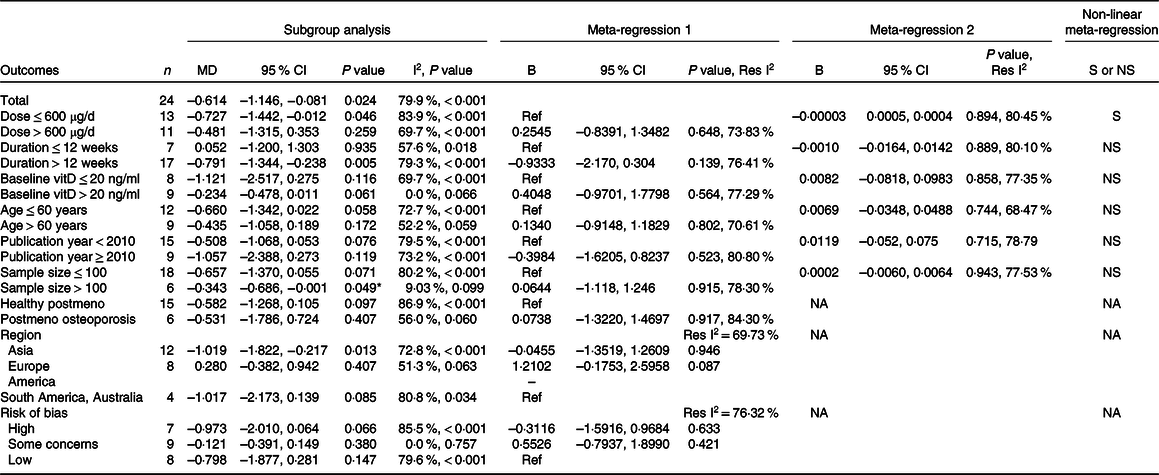
N, number of included interventions; B, beta coefficient reflecting the effect of the subgrouping variable on the pooled effect size; vit, vitamin; postmeno, post-menopausal; Res I2, residual I2; NA, not applicable; OC, osteocalcin; S, significant; NS, non-significant. Italic; P values.
Meta-Regression 1: the subgrouping variable was included into the model as a categorised variable. Meta-Regression 2: the subgrouping variable was included into the model as a continuous variable.
* Significance with respect to heterogeneity.
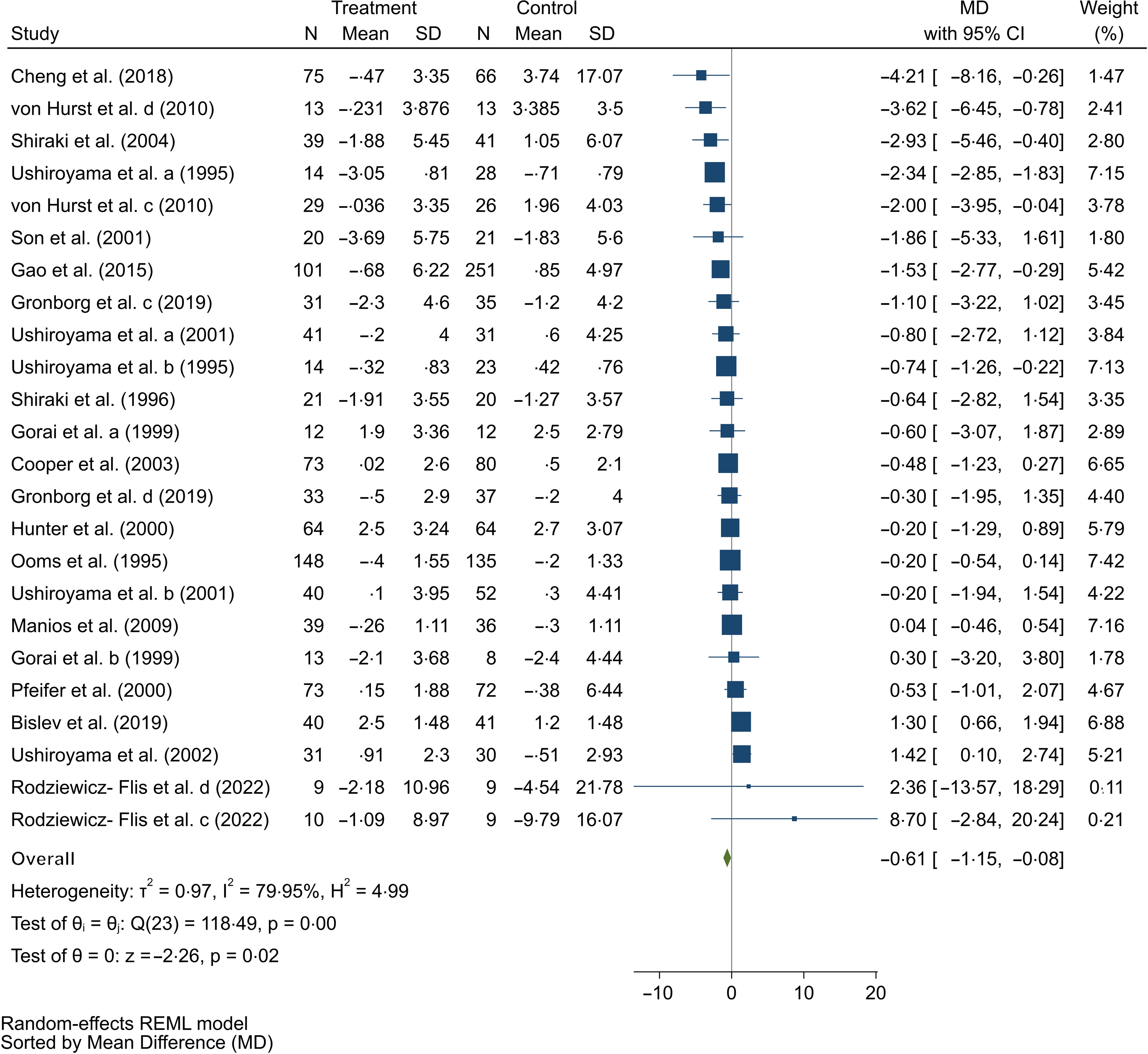
Fig. 3. Forest plot of the randomised clinical trials (RCT) examining the effect of vitamin D supplementation on OC. Data have been expressed as mean differences (MD) between intervention and control groups with 95 % CI. Estimates were pooled using the random effects model. Letters between parentheses represent: a, b: different intervention/control groups; c, d: different participant groups.
Subgroup analysis yielded that a decrease in OC level was significant in the subgroup with larger sample size (MD = 0·343, 95 % CI (–0·686, −0·001), P = 0·049, online Supplementary Fig. S6). Although there was very low heterogeneity in subgroups including sufficient baseline vitamin D level and studies with some concerns risk of bias, no significant difference was found in mean level of OC between the treatments. Meta-regression analyses indicated the pooled estimate was independent of potential sources of heterogeneity, but the dose was found to have a non-linear effect on the pooled effect size (online Supplementary Fig. S15).
Effect of vitamin D supplementation/fortification on procollagen type-1 intact N-terminal propeptide
The analysis found no change in P1NP marker with vitamin D consumption (n 17, Table 4, online Supplementary Fig. S7), with considerable heterogeneity (I2 = 60·8 % and P = 0·007). However, a significant reduction was detected in P1NP level in the vitamin D dosage ≤ 600 μg/d subgroup (MD: −3·068, 95 % CI –5·894, −0·242, P = 0·033, online Supplementary Fig. S8) and sample size more than 100 participants (MD = −2·339, 95 % CI (–4·414, −0·264), P = 0·027) without any heterogeneity (online Supplementary Fig. S9).
Table 4. Summary findings of comparison of P1NP between the study treatments
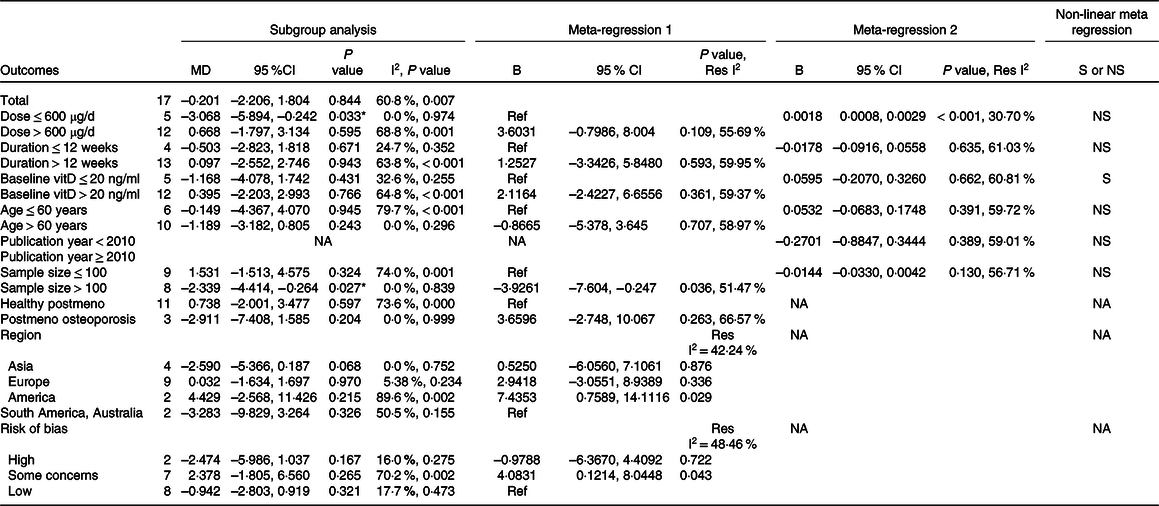
P1NP, procollagen type-1 intact N-terminal propeptide; MD, mean difference; N, number of included interventions; B, beta coefficient reflecting the effect of the subgrouping variable on the pooled effect size; vit, vitamin; postmeno, post-menopausal, Res I2, residual I2; NA, not applicable; S, significant; NS, non-significant. Italic; P values.
Meta-Regression 1: the subgrouping variable was included into the model as a categorised variable. Meta-Regression 2: the subgrouping variable was included into the model as a continuous variable.
* Significance with respect to heterogeneity.
Our analysis presented that dose, sample size, region, quality of the studies and baseline vitamin D level could be the potential sources of heterogeneity. Linear meta-regression showed that 100 μg/d increase in dose associated with 0·18 significant decrease in MD of OC level which is in favour of the intervention group (MD = 0·0018, 95 % CI (0·0008, 0·0029), P < 0·001, online Supplementary Fig. S13). Furthermore, meta-regression model revealed that the MD for OC was significantly decreased in studies with larger sample sizes compared with those with lower sample sizes (MD = −3·9261, 95 % CI (–7·604, −0·247), P = 0·036). In addition, subgroup analysis showed that all subgroups of region except America and all subgroups of study quality except some concerns risk of bias had non-significant heterogeneity, and the results of classified meta-regression were completely consistent with the results of subgroup analysis. Finally, the baseline vitamin D level was found to have a non-linear effect on the pooled effect size (online Supplementary Fig. S16).
Effect of vitamin D supplementation/fortification on bone alkaline phosphatase
According to the pooled effect size of six studies, vitamin D intake did not significantly alter BALP levels (n 6, online Supplementary Table S2 and Fig. S10), with high heterogeneity (I2 = 95·3 % and P < 0·001). No significant effect was observed in any of the subgroups. In addition, meta-regression analysis showed the pooled estimate was independent of possible confounding factors.
Risk of bias and publication bias of the included studies
A summary of the risk of bias analysis and traffic light figure of each domain of the risk of bias assessment are presented in online Supplementary Figures S17 a, b. One study had a high risk of bias and ten studies were categorised as ‘some concerns’ due to improper randomisation procedure. Nineteen studies were scored as having some concerns of deviation from intended interventions. Moreover, one study had a high risk of missing outcome data, whereas six studies had some concerns of detection bias. Measurement details were sufficiently reported in all studies. Furthermore, only one study reported some concerns regarding the selection of the results.
Funnel plots for the effects of vitamin D supplementation/fortification on bone turnover markers (including sCTX, uNTX, OC, P1NP and BALP) are presented in online Supplementary Fig. S18–S22. Based on visual inspection of the funnel plots, there was no evidence of publication bias. These findings were also confirmed by Egger’s test for sCTX (P = 0·691), uNTX (P = 0·847), OC (P = 0·675), P1NP (P = 0·251) and BALP (P = 0·946).
Sensitivity analysis and quality of evidence
Based on the results, the estimated effect size of each outcome was not affected by any single study.
The GRADE-Pro evidence profile rating results for changes in bone turnover markers are shown in online Supplementary Table S3. The GRADE rating was found to be high for sCTX, uNTX and P1NP variables. However, results showed the GRADE rating was moderate and low for OC and BALP, respectively.
Discussion
The primary objective of this systematic review and meta-analysis was to investigate the probable effect of vitamin D supplementation or fortification on bone turnover markers. In summary, the results of this meta-analysis suggest that vitamin D consumption benefits bone resorption markers, including sCTX and uNTX. Moreover, vitamin D significantly reduced OC (a bone formation marker), but not P1NP or BALP.
Vitamin D intake was found to significantly reduce sCTX, particularly at age younger than 60 years, studies conducted on Europe and America and studies with some concerns for risk of bias. Consistent with our results, Von Hurst et al. found that vitamin D supplementation could modify bone turnover, suppress bone resorption and prevent deterioration in quality of life related to ageing(Reference Von Hurst, Stonehouse and Kruger43). Conversely, Valimaki and colleagues did not find a significant effect of supplementing with 100 000 or 200 000 μg of vitamin D every 3 months on sCTX levels(Reference Välimäki, Löyttyniemi and Pekkarinen10), which may be at least partly related to such large doses of vitamin D being administered far apart. The placebo group also received Ca supplements, which may have affected the results.
There are different pathways that could explain the beneficial effects of vitamin D on bone turnover markers. In the absence of sufficient concentrations of serum 25(OH)D (< 20 ng/ml), Ca absorption from the intestine is decreased, resulting in increased PTH to compensate Ca reabsorption from the kidney, stimulating osteoclasts to release Ca into blood circulation(Reference Holick7,Reference Yamamoto, Kawanobe and Takahashi49) and deteriorating osteoblasts function due to low 25(OH)D levels(Reference Baldock, Thomas and Hodge50). Moreover, available evidence suggested that insufficient vitamin D level may cause secondary hyperparathyroidism, leading to increased bone loss, bone turnover and, consequently, greater risk of osteoporosis(Reference Ardawi, Qari and Rouzi51). Recent evidence has shown that the risk of vitamin D deficiency is considerably higher in the older adults and in the Middle East, China, Mongolia and India(Reference van Schoor, de Jongh and Lips52), for instance it has estimated that 490 million Indians have vitamin D deficiency(Reference Amrein, Scherkl and Hoffmann53). On the other hand, prevalence rate of vitamin D deficiency has reported 24 % in America (USA) and 40 % in Europe(Reference Amrein, Scherkl and Hoffmann53). These results are consistent with those obtained from this meta-analysis, which showed that groups with participants who had presumably normal baseline vitamin D levels had significantly lower sCTX levels.
Analysing all included RCT showed a significant reduction in uNTX level after vitamin D intake. Similar to our findings, intake of the vitamin D-fortified skimmed milk with 200 μg/d content of vitamin D3 for 16 weeks reduced both uNTX and P1NP, as well as increased circulating 25(OH)D in young healthy women(Reference Toxqui, Pérez-Granados and Blanco-Rojo11). However, findings from another study did not support the mentioned effect of vitamin D supplementation on uNTX during 48 weeks(Reference Majima, Komatsu and Shimatsu31), which may be due to the low dosage (40 μg/d) of alfacalcidol treatment.
This meta-analysis also found that vitamin D consumption significantly reduced OC particularly in studies with larger sample size (more than 100 participants). According to another meta-analysis, simultaneous administration of vitamins D and K could significantly improve the total BMD and reduce undercarboxylated OC(Reference Kuang, Liu and Guo54). Additionally, a beneficial effect of vitamin D on OC is supported by Shiraki et al., where OC was reduced after alfacalcidol treatment for 6 months(Reference Shiraki, Fukuchi and Kiriyama32). Another study conducted in Emirati women indicated that serum 25(OH)D concentration is negatively associated with PTH and OC levels (r = −0·13, P < 0·05)(Reference Saadi, Nagelkerke and Benedict55). Moreover, vitamin D3 supplementation in diabetic individuals also reduces undercarboxylated OC level and the undercarboxylated to carboxylated OC index (uOC/cOC)(Reference Aguayo-Ruiz, García-Cobián and Pascoe-González56). However, Bislev et al. found no significant change in bone turnover markers (CTX and P1NP), and OC levels indicated a borderline elevation due to vitamin D3 intake(Reference Bislev, Langagergaard Rødbro and Rolighed28). In addition to the PTH pathway involved in bone turnover, there is evidence that the promoter region of the OC gene has a vitamin D receptor binding site, although the exact relationship is not apparent(Reference Boucher-Berry, Speiser and Carey57).
Results of our meta-analysis revealed that lower vitamin D administration (≤ 600 μg/d) and larger sample size (> 100 participants) significantly decreased P1NP levels, but this effect was not found in the overall analysis. Zhue et. al.(Reference Zhu, Bruce and Austin45) showed that although P1NP levels significantly decreased due to vitamin D intakes of 1000 μg/d during 48 weeks, no difference was observed between the treatment and placebo groups. Moreover, Gronborg et. al. did not declare any significant changes in bone turnover markers including P1NP, OC, BALP and sCTX following daily intake of 1200 μg vitamin D for 12 weeks(Reference Grønborg, Tetens and Andersen27). Similarly, Madar et. al. investigated the effect of 10 and 25 μg/d vitamin D treatment in healthy individuals and discovered significant acceleration in 25(OH)D and reduction in PTH levels, but no changes in serum P1NP(Reference Madar, Knutsen and Stene58). Gao et al. found that simultaneous intake of Ca and calcitriol significantly decreased P1NP, while no significant effect was seen in individuals receiving cholecalciferol(Reference Gao, Zhu and Liu26). Consistent with these results, no extra effect of vitamin D was seen on P1NP when comparing a Ca group and Ca plus vitamin D group(Reference Zhu, Bruce and Austin45). We hypothesise that in subjects with adequate Ca intake, the effect of vitamin D on P1NP levels is not evident.
One reason why the role of vitamin D supplementation and its dosage is still debated might be due to limitations in trial design as most studies did not meet the basic requirements of a nutrient intervention study, including vitamin D-replete populations, too small sample sizes and inconsistent intervention methods regarding dose and metabolites(Reference Amrein, Scherkl and Hoffmann53). Our meta-analysis results clearly showed the role of larger sample size in studies.
The effect of vitamin D supplementation on BALP marker was not significant in the overall analysis nor in the subgroup, which is inconsistent with the findings of Ooms et. al. and Chung et al.(Reference Chung, Koo and Kwon21,Reference Ooms, Roos and Bezemer48) . In agreement with our results, a recent meta-analysis showed no significant changes in BALP levels following consumption of vitamin D fortified foods(Reference Tangestani, Djafarian and Emamat12). The limited number of studies included in this section of our analysis might prevent meaningful evaluation of BALP.
A strength of our analysis was the comprehensive assessment of the effect of vitamin D supplementation or fortification on several key bone turnover markers (including bone resorption and formation factors) in women, as recent evidence supports sex differences in vitamin D serum levels and metabolism(Reference Wierzbicka and Oczkowicz59). However, this meta-analysis has some limitations. First, moderate to high heterogeneity was found in some variables although we attempted to identify the source of heterogeneity. Furthermore, there are limitations to the general use of the results obtained for uNTX and BALP markers, as few studies have examined these markers (n 6) and when performing subgroup analyses, some domains had few or no studies. Moreover, considering the various types of vitamin D in form of supplements or fortified foods, it was not possible to subgroup based on the type of vitamin D prescribed.
Conclusions
In conclusion, this study found a favourable effect of vitamin D consumption on bone turnover markers in women, indicating that vitamin D supplementation or fortification might be an effective nutritional strategy for improving bone health. The meta-analysis showed that vitamin D intake significantly reduced bone resorption markers including sCTX and uNTX. A significant reduction in levels of OC, a bone formation marker, was also observed. However, there was no significant effect of vitamin D supplementation or fortification on P1NP and BALP levels. This meta-analysis suggested that age, sample size, dose, duration, baseline vitamin D level, study region and quality of studies might be sources of heterogeneity. Lastly, this meta-analysis did not find a consistent dose–response relationship between vitamin D and bone turnover markers in women.
Acknowledgements
This research was supported by a grant from Shiraz University of Medical Sciences (grant no. 27 339).
The authors’ responsibilities were as follows: N. N., Sh. F., A. A., N. Z. and M. N.: protocol development and searches; A. A. and N. Z.: screening; A. A., N. Z. and N. N.: data extraction, risk of bias assessment and synthesis; N. N. and E. S.: data harmonisation and meta-analysis; N. N.: grading the evidence; N. N. and S. J.: drafting of the manuscript; N. B.: editing the manuscript; Sh. F. and N. N.: critical revision of the manuscript for important intellectual content; Sh. F.: had primary responsibility for the final content and all authors: reviewed and commented on versions of the manuscript and read and approved the final manuscript.
The authors have no conflict of interests to declare.
Supplementary material
For supplementary material accompanying this paper visit https://doi.org/10.1017/S0007114524000060