The cost of rearing replacement heifers is one of the highest investments in the dairy industry. Thus, proper nutritional and management practices must be placed to maximise the return of investment. Particularly, during the preweaning and early weaning period, the most critical management goals are to minimise the incidence of diseases and mortality and maximise growth. Several studies have concluded that the benefits of proper preweaning management, leading to maximise average daily gain (ADG), have not only short but also lifelong impacts on heifers’ performance(Reference Soberon and Van Amburgh1,Reference Gelsinger, Heinrichs and Jones2) .
The discovery of the essentiality of fatty acids (FA), namely linoleic(Reference Burr and Burr3) and α-linolenic acids(Reference Duttaroy4–Reference Anderson and Connor6), led the livestock scientific community to further investigate the role of these essential FA (EFA) and their derivatives arachidonic acid (C:20 : 4n-6) and EPA (20 : 5n-3) and DHA (22 : 6n-3), respectively. FA are more than structural components of TAG; as components of cell membranes, they regulate signal transduction(Reference Simons and Ikonen7). Several studies in humans and rodents have reported that n-3 compared with n-6 FA are more potent activators of genes involved in lipid oxidation, more potent reducers of genes encoding enzymes for lipid synthesis and important regulators of immune response(Reference Clarke8,Reference Sampath and Ntambi9) .
Studies have confirmed that the strategic feeding of FA enriches tissues and body fluids with the corresponding supplemented FA. In dairy cattle, dams supplemented with prepartum diets enriched in linoleic acid(Reference Garcia, Greco and Favoreto10) or EPA and DHA(Reference Jolazadeh, Mohammadabadi and Dehghan-banadaky11) produced colostrum with greater concentrations of the respective supplemented FA compared with colostrum from non-supplemented cows. Furthermore, plasma of newborn dairy calves born from dams fed diets enriched in EPA and DHA(Reference Moallem and Zachut12) or linoleic acid(Reference Garcia, Greco and Favoreto13) had increased concentrations of the FA fed to their dams. In beef cattle, plasma (30 or 60 d of age) and adipose tissue (60 d of age) of calves suckling dams supplemented with high-oleic or high-linoleic safflower seeds had increased concentrations of the supplemented FA in plasma and adipose tissue, compared with calves suckling non-supplemented dams(Reference Lake, Scholljegerdes and Weston14). Furthermore, preweaned dairy calves fed milk replacer (MR) supplemented with EFA also reported increased concentrations of the corresponding EFA and/or that of their derivatives in plasma(Reference Garcia, Greco and Favoreto13,Reference Jenkins, Kramer and Sauer15,Reference Jenkins and Kramer16) and liver(Reference Jenkins and Kramer16,Reference Garcia, Shin and Schlaefli17) .
Previous studies have reported the benefits of feeding diets enriched with EFA during late uterine life on performance of newborn calves. Salehi et al. (Reference Salehi, Ambrose and Oba18) reported better concentrations of colostrum IgG and Garcia et al. (Reference Garcia, Greco and Favoreto13) reported better efficiency of IgG absorption of calves born from dams fed diets enriched with EFA. Adequate passive transfer of IgG is crucial to minimise neonatal morbidity and mortality and strengthen calf immunity(Reference Quigley and Drewry19). Calves born from dams supplemented with long-chain FA during the prepartum may have enterocytes with a particular FA profile that could benefit passive transfer of immunoglobulins(Reference Garcia, Greco and Favoreto10). Furthermore, strategic supplementation of EFA in MR(Reference Garcia, Greco and Favoreto13,Reference Hill, Aldrich and Schlotterbeck20) or calf starter (CS)(Reference Hill, Bateman and Aldrich21,Reference Hill, Bateman and Aldrich22) fed to preweaned calves has been reported to impact calf metabolism, improve growth, feed efficiency (FE), health, and immune status.
The hypothesis of this study was that supplementing prepartum diets with Ca-salts of soyabean oil (CSO) or Ca-salts of fish oil (CFO) would improve immune status, health and growth of newborn calves compared with calves born from dams non-fat-supplemented. Another hypothesis was that calves born from dams supplemented with EFA or their derivatives would benefit to a greater extent from eating a CS supplemented with Ca salts of unsaturated FA. Therefore, the objective was to evaluate the effect of supplementing diets enriched in linoleic acid or EPA and DHA to late- gestation Holstein cattle on metabolic profile, immune status, health and growth of calves consuming starter diets enriched in oleic and linoleic acids during pre- and postweaning periods.
Methods
Dam management and dietary treatments
The experiment was conducted from July 2016 through October 2016, at a commercial dairy farm (Zarin Khoshe Dairy Farm). All experimental procedures were conducted according to The Care and Use of Agricultural Animals in Research and Teaching(23) guidelines. The Animal Experiment Committee at Tehran University, Karaj, Iran, approved all procedures and guidelines involving animals. The present study was part of a large research project, with a portion of the results (prepartum and postpartum performance of calves’ dams) reported elsewhere(Reference Jolazadeh, Mohammadabadi and Dehghan-banadaky11). Briefly, pregnant nulliparous (n 42) and previously parous (n 78) Holstein cattle were blocked by parity and enrolled in the study starting at 3 week before their calculated parturition date. The basal prepartum diet was formulated to have low concentrations of total FA (1·87 % of DM), linoleic acid (0·84 % of DM) and α-linolenic acid (0·36 % of DM). Prepartum cattle were fed one of the following three diets: (1) no fat supplement (CON), (2) 1·15 % of dietary DM as CSO (140 g/cattle per d) supplement (Persiafat; Kimiya Danesh Alvand Co.) or (3) 1·15 % of dietary DM as CFO (140 g/cattle per d) supplement (Persiafat; Kimiya Danesh Alvand Co.). The CSO and SFO diets were isoenergetic but had different FA profiles (linoleic acid: 1·30 and 0·87 %; EPA and DHA: 0 and 0·15 % for CSO and SFO, respectively). All diets were isonitrogenous. Ingredient and chemical composition of the prepartum diets and specific details on prepartum feeding and management were reported previously(Reference Jolazadeh, Mohammadabadi and Dehghan-banadaky11).
Calving management and colostrum, milk and starter feeding
Calves were born in a sod-based pen. All dams were monitored for signs of calving initiation every 30 min between 05.30 and 15.30 hours and then every 2 h between 15.30 and 05.30 hours. Within 2 h of birth, calves were weighed and ear-tagged, and the umbilical cord was disinfected with 10 % Betadine solution (Purdue Frederick Co.). Calves were housed temporarily in individual pens (1 × 1 m) equipped with a heat lamp before moving to individual wired pens (1 × 1·5 m) bedded with sand between 6 and 16 h of age. Cows were milked with a cow-side vacuum pump within 1 h after calving. Colostrum from each cow (4 litres) was fed to their corresponding calf, regardless of IgG concentration. Remnant colostrum (>1 litre and >50 g of IgG/l) was stored at –20°C to fed calves born from dams fed the same dietary treatment but producing low amounts of colostrum. Calves were considered to have adequate passive transfer if the plasma concentration of total IgG was ≥10 g/l after 24 h of colostrum feeding(Reference Tyler, Hancock and Parish24). Whole colostrum samples were collected and frozen (−20°C) for later analyses of IgG concentration and FA profile, and results were reported elsewhere(Reference Jolazadeh, Mohammadabadi and Dehghan-banadaky11). Briefly, the FA profile of colostrum resembled that of the prepartum diets fed to dams during late gestation(Reference Jolazadeh, Mohammadabadi and Dehghan-banadaky11). Furthermore, given that an extensive body of research has confirmed that the FA profile of tissues and body fluids resembles that of the supplemented diets, coupled with budgetary restrictions, we did not measure the FA profile of tissues or body fluids of calves in the present study. Plasma samples of newborn calves (at birth and 24 h after colostrum feeding) were measured for bovine total IgG concentration using radioimmunodiffusion(Reference Garcia, Greco and Favoreto10). Intake of IgG was calculated using the concentration of colostrum IgG reported previously(Reference Jolazadeh, Mohammadabadi and Dehghan-banadaky11). The apparent efficiency of IgG absorption (AEA, %) was calculated according to Quigley et al.(Reference Quigley, Drewry and Martin25) using the following equation: IgG concentration in plasma at 24 h of life (g/l) × (0·099 × body weight (BW) (kg) at birth)/IgG intake (g). Plasma volume was estimated at 9·9 % of birth weight(Reference Quigley, Drewry and Martin25). Eighty-four Holstein calves were used in the present study (n 14 calves per factorial treatment, ten males and four females) in a completely randomised design, with dietary treatments in a 3 × 2 factorial arrangement (three dam diets (DD) and two CS; n 6). Calves were fed warm (38°C) pasteurised tank milk twice per d (08.00 and 17.00 hours) using steel buckets, starting at 3 d of age, and always drank all their offered milk. Volumes of milk fed decreased with age: 4 litres from day 4 to 7, and then 5, 6, 7, 6·5, 5·5, 4 and 2 litres during weeks 2 to 8, respectively. The average nutritional composition of milk was 11·50 % DM, 2·98 % crude protein (CP), 3·35 % fat and 4·51 % lactose. Calves had ad libitum access to one of two CS: (1) no fat supplement (FC-0) or (2) supplemented with 2 % fat Ca-salt rich in unsaturated FA, primarily oleic and linoleic acids (FC-2; Persiafat, Kimiya Danesh Alvand Co.; Table 1). All calves were weaned at 56 d but the experimental CS were fed until 77 d of age. CS were isonitrogenous, and the ingredients and composition are shown in Table 2. All calves had free access to clean and freshwater at all times. The offered starter was adjusted daily to achieve 5–10 % refusals (i.e. the portion of the starter not consumed over a 24-h period); and refusals were collected and weighed daily at 08.00 hours. Chopped high-quality alfalfa hay (5 % of CS) was included in the starter diets once calves were 30 d of age. The starter diets (CS or CS + alfalfa hay) were offered once daily at 09.00 hours.
Table 1. Fatty acid (FA) profile (g/100 g of total FA) of calf starters and fat supplement used

FC, fat Ca-salts; FC-0, calf starter with no fat supplement; FC-2, calf starter supplemented with 2 % fat Ca-salt; UFA, unsaturated FA.
* Fat Ca-salt rich in unsaturated FA (Persiafat, Kimiya Danesh Alvand Co.).
Table 2. Ingredients and nutrient composition (g/kg DM unless otherwise noted) of calf starters

FC-0, calf starter with no fat supplement; FC-2, calf starter supplemented with 2 % fat Ca-salt; CP, crude protein; NDF, neutral-detergent fibre; ADF, acid-detergent fibre; NFC, non-fibre carbohydrates; ME, metabolisable energy, NEm, net energy for maintenance; NEg, net energy for gain.
* Fat Ca-salts, Persiafat, Kimiya Danesh Alvand Co., contained 85 % fat and 9 % Ca (1 % C14.0, 28 % C16 : 0; 3 % C16 : 1; 5 % C18 : 0, 26 % C18 : 1, 30 % C18 : 2; 3 % C18 : 3, 4 % other).
† Vitamin–mineral premix contained per kg: Ca 160 g; Mg 40 g; Na 30 g; Fe 3 g; Cu 3 g; Zn 7 g; Mn 4 g; I 0·08 g; Co 0·05 g; Se 0·06 g; vitamin A 800 000 IU; vitamin D 150 000 IU; vitamin E 2000 IU.
‡ Calculated as DM – (NDF + CP + ether extract + ash) (National Research Council, 2001).
§ Estimated using National Research Council (2001) equations.
Data collection and sampling
All calves (n 84) were weighed at birth, 56 d and 77 d of age to measure changes in BW and calculate ADG. In addition, all calves were weighed once a week to observe weekly growth patterns. Body measurements of all calves were taken at 3 d of age, weaning (d 56) and the end of the study (d 77), in the morning and before feeding according to Lesmeister & Heinrichs(Reference Lesmeister and Heinrichs26). Body measurements included heart girth (circumference of the chest), hip width (distance between the points of hook bones), body length (distance between the points of shoulder and rump), body barrel (circumference of the belly before feeding), wither height (distance from the base of the front feet to wither) and hip height (distance from the base of the rear feet to the hook bones).
Amount of starter diets offered and refused per calf were recorded daily throughout the experiment. Feed samples were collected weekly and stored at –20°C for chemical analyses. Subsamples of feeds and refusals were mixed thoroughly, dried at 55°C for 48 h and ground to pass through a 1 mm screen in a hammer mill (Arthur Hill Thomas Co.) before chemical analyses for DM (method 934.01), CP (method 988.05) and ether extract (method 920.39)(27). The neutral-detergent fibre contents were analysed according to Van Soest et al.(Reference Van Soest, Robertson and Lewis28), assayed with sodium sulphite, without α-amylase, and were expressed with residual ash. Eight representative samples of milk were collected throughout the study and analysed for DM, fat, CP and lactose content by infrared spectroscopy (Foss Electric). FE (kg of BW gain/kg of total DM intake (DMI)) was calculated for the preweaning period, days 57–77 and for the extent of the study. Total DMI corresponds to the amount of DMI contributed by the starter diets (CS and alfalfa hay) and by the fixed amounts of milk provided to each calf.
Total tract apparent digestibility of DM, organic matter, CP, neutral-detergent fibre, acid-detergent fibre and ether extract were determined using acid-insoluble ash as an internal marker(Reference Van-Keulen and Young29). Faecal samples were obtained from each calf twice per d (in the morning and afternoon) during four consecutive days (from days 74 to 77). Faecal samples were dried at 55°C in a forced air oven, ground to pass a 1-mm screen in a Wiley mill (Arthur Hill Thomas Co.) and then mixed thoroughly.
Samples of ruminal fluid (approximately 150 ml) were collected from each calf on days 30 and 70, about 3 h after the morning feeding, using a stomach tube attached to an Erlenmeyer flask and vacuum pump; the first 50 ml were discarded to avoid possible saliva contamination. The ruminal fluid was filtered through four layers of cheesecloth, and pH was determined immediately using a portable pH meter (Sentron, model A102-003). Approximately 25 ml of filtrate were then preserved by adding 5 ml of 25 % metaphosphoric acid solution for later determination of volatile FA (VFA), and approximately 20 ml of filtrate were combined with 20 ml 0·2 M HCl for later measurement of NH3-N concentration. The prepared samples were stored at –20°C and then thawed for analysis of respective compounds. Stored samples were centrifuged at 1200 g for 10 min, and the supernatant fluid was analysed for VFA by GC (model 5890; Hewlett-Packard). Ruminal NH3-N concentration was determined according to the procedures described by Crooke & Simpson(Reference Crooke and Simpson30). Blood samples were collected from each calf immediately after birth, 24 h after colostrum feeding and then 3 h after the morning meal on days 28, 56 and 77 of age. Blood was taken from the jugular vein by evacuated tubes containing K2 EDTA (10·5 mg, Monoject; Sherwood Medical). Samples were immediately chilled and then plasma was obtained by centrifugation at 3000 g for 15 min and stored at –20°C. Plasma concentrations of glucose, total protein, albumin, alkaline phosphatase (ALP), cholesterol and TAG were determined using an automatic microplate Reader (EON-BIOTEK) and commercial kits (Pars Azmoon). Enzymatic assays were used for analyses of plasma insulin (DRG), blood urea N (Pars Azmoon) and NEFA (Randox Laboratories Ltd.) in an automatic spectrophotometer (Clima Plus; RAL) following the manufacturer’s instructions. Behavioural data (eating, ruminating, standing, lying and non-nutritive oral behaviour) were monitored by direct observations of each calf in the time (minutes) devoted to each monitored behaviour, once at the last week of the preweaning period (35–42 d) and once at the last week of the postweaning period (70–77 d)(Reference Castells, Bach and Araujo31). Behavioural data were monitored for 8 consecutive hours following the morning milk feeding (at 08.00 hours) and the CS (09.00 hours) during the last week of the pre- and postweaning periods, respectively.
Calf scoring for health assessment and incidence of health disorders
Attitude, faecal consistency and nasal discharge were scored daily for each calf after the first daily feeding of CS by a single observer using a scale from 0 to 3 (http://www.vetmed.wisc.edu/dms/fapm/fapmtools/8calf/calf_health_scoring_chart.pdf). Attitude scores recorded were (0) responsive, (1) non-active, (2) depressed or (3) moribund. Faecal consistency scores recorded were (0) firm, no diarrhoea; (1) moderate, soft no diarrhoea; (2) runny faeces, mild diarrhoea; or (3) watery faeces, diarrhoea. Nasal score scale of calves was also scored on a 0–3 scale, with 0 being normal serous discharge, 1 being a small amount of unilateral cloudy discharge, 2 being bilateral cloudy or excessive mucus discharge and 3 being copious bilateral mucopurulent discharge. Calves given a faecal score >1 were considered to have diarrhoea, and those with a score of 3 were considered to have severe diarrhoea. Calves given a score > 0 for other criteria were considered as having the disorder. Weekly averages of each score were calculated for statistical analysis. Incidences of health disorders were recorded daily for individual calves. Respiration rate (RR) was measured at 14.00 hours on days 30, 45 and 70 by visual observation for three 1-min periods (not consecutive), according to the methods described by Yari et al.(Reference Yari, Nikkhah and Alikhani32). Rectal temperature (RT) was measured weekly before morning meals using a rectal thermometer (Yasa Teb Co.), and calves with RT over 39·5°C were categorised as febrile. Calves were vaccinated according to the herd vaccination protocol. Calves with digestive and respiratory problems were treated by farm personnel according to protocols established by the herd veterinarian. All calves remained healthy and exhibited no signs of illness during the experiment.
Statistical analyses
Statistical analyses were conducted for four periods: the first day of life (passive immunity parameters), preweaning (days 0–56), postweaning (days 57–77) and the entire experimental period (days 0–77). Except for starter intake and measures of structural growth, the first measure was performed on day 3 of life. The study was a complete randomised design, with dietary treatments in a factorial arrangement 3 × 2 (3 DD and 2 CS; n 6 treatments). The mixed model equation for passive immune variables was defined as Yijk = μ + α i + β j + (αβ)ij + e ijk, where Yijk is the dependent variable, μ is the population mean, α i is the treatment effect, β j is the fixed effect of parity, (αβ)ij is the interaction effect of treatment and parity and e ijk is the residual error. The passive immunity variables were analysed using the PROC MIXED procedure of SAS(33). Contrasts testing the effects of DD were: (1) FAT: control v. CFO + SFO and (2) SFO: CSO v. CFO, (3) effect of parity (primiparous v. multiparous), and the contrasts testing the interactions of DD and parity (4) FAT × parity (contrasts 1 and 3) and (5) SFO × parity (contrasts 2 and 3).
For other continuous variables (rumen fermentation parameters, plasma metabolites, BW, skeletal growth, behaviour data, RT, respiratory rate and apparent nutrient digestibility), the data were analysed using the PROC MIXED procedure of SAS(33). Faecal and health scores were analysed using a multivariable logistic mixed model (GLIMMIX procedure of SAS). The following model was used for variables with repeated-measures, for those with non-repeated-measures the effect of time and its interaction were omitted:

where Y ijklm is the observation; μ is overall mean; D i is the fixed effect of DD (CON, CSO or CFO); C j is the fixed effect of CS (FC-0 or FC-2); T k is the effect of time; DC ij is the interaction of DD × CS; Dt ik is the effect of the interaction between DD and week; Ct jk is the effect of the interaction between CS and week; DCt ijk is the three-way interaction of DD, CS and time; and ε ijk is the residual error. Five orthogonal contrasts of DD, CS and interactions were tested for variables measured after initiation of starter feeding. Contrast testing the effects of DD were (1) FAT: control v. CFO + SFO, (2) SFO: CSO v. CFO, contrast for the effect of CS, (3) FC-0 v. FC-2, and the contrast testing the interactions of DD and CS, (4) FAT × CS (contrasts 1 and 3) and (5) SFO × CS (contrasts 2 and 3).
The denominator df were computed using the approximation described by Kenward & Roger(Reference Kenward and Roger34). Significance was declared at P ≤ 0·05 and trends at 0·05 < P < 0·10. Tukey’s adjustment was used for multiple comparison tests.
Results
Calf absorption of colostral IgG
Total IgG intake was greater in calves born for dams fed fat (CSO or CFO) compared with calves born from dams fed CON (504 v. 392 g, P < 0·01; Table 3). Concentrations of total IgG in plasma of calves born from dams fed fat supplements compared with calves born from CON-fed dams were 43 and 30 % greater (P < 0·01) when expressed as g/l and % total protein, respectively. Calves born from dams fed fat supplements had better (P < 0·01) AEA than calves born from CON-fed dams (27·7 v. 22·2 %).
Table 3. Passive immunity in calves born from Holstein cows fed prepartum diets non-supplemented (CON) or supplemented with calcium-salts of soyabean oil (CSO) or calcium-salts of fish oil (CFO) fatty acids, during the last 3 weeks before expected parturition
(Mean values and pooled standard errors)

CSO, supplementation with 140 g/d Ca-salts of soyabean oil; CFO, supplementation with 140 g/d Ca-salts of fish oil during the last 3 weeks before expected parturition; TP, total protein; AEA, apparent efficiency of IgG absorption; BW, body weight.
* FAT = CON v. fat supplement; SFO = CSO v. CFO. Interactions of FAT and SFO with parity were not significant for any parameter reported in this table (P > 0·40).
† IgG intake was calculated using IgG concentration in colostrum, reported in our previous paper(Reference Jolazadeh, Mohammadabadi and Dehghan-banadaky11) and the amount offered colostrum was 4 litres, which was similar for all calves.
‡ AEA (%) = (IgG concentration in serum at 24 h of life (g/l) × (0·099 × BW at birth (kg))/IgG intake (g)) × 100.
Starter intake and growth performance
BW at birth was not impacted by prepartum diets, but BW at weaning (56 d) and 77 d were impacted by prepartum diets (Table 4). Weekly BW, as affected by prepartum diets, started to differentiate at 8 weeks of age, and indeed calves born from dams fed either CSO or CFO were heavier than those born from dams fed CON (Fig. 1(a)). For the preweaning period, calves born from dams fed fat supplements had greater ADG (542 v. 497 g/d; P = 0·01; Table 4) compared with calves born from dams fed CON prepartum. Although ADG during the postweaning period was not impacted by prepartum diets, the effect observed during the preweaning period carried on so that the ADG for the total experimental period was also greater for calves born from dams fed fat supplements compared with those born from dams fed CON (597 v. 558 g/d; P = 0·02). Regardless of DD, calves fed FC-2 had consistently greater (P < 0·01) ADG compared with calves fed the FC-0 CS during the pre-weaning (561 v. 493 g/d), postweaning (781 v. 690 g/d) and overall (621 v. 547 g/d) periods. The better ADG of calves fed CF-2 is consistent with their heavier BW, which became significantly different after 6 weeks of age (Fig. 1(b)). Starter intake was not affected by prepartum or starter diets at any evaluated period. FE tended (P = 0·06) to be better for preweaned calves born from dams fed fat supplements compared with those born from CON-fed dams (0·55 v. 0·51). Regardless of DD, calves fed FC-2 had better (P < 0·01) FE compared with calves fed FC-0 CS, during the preweaning (0·58 v. 0·50), postweaning (0·53 v. 0·46) and overall experimental (0·45 v. 0·39) periods. Neither DD nor CS or their interactions affected the total tract apparent digestibility of DM, CP, organic matter, EE, acid-detergent fibre and neutral-detergent fibre measured from 74 to 77 d of age.
Table 4. Effects of dietary treatments on body weight, starter intake, average daily gain (ADG), gain:feed ratio and apparent digestibility of Holstein calves (n 14 per treatment) fed a calf starter (CS) containing no fat supplement (FC-0) or supplemented with 2 % fat calcium-salt (FC-2) in pre- and postweaning periods
(Mean values and pooled standard errors)

CON, no fat supplement (control); CSO, supplementation with 140 g/d Ca-salts of soyabean oil; CFO, supplementation with 140 g/d Ca-salts of fish oil during the last 3 weeks before expected parturition; CP, crude protein; OM, organic matter; NDF, neutral-detergent fibre; ADF, acid-detergent fibre; DMI, DM intake.
* Starter was offered from 3 d of age. Preweaning: 0–56 d. Postweaning: 57 to 77 d old.
† P values for orthogonal contrasts: FAT = control v. CSO + CFO; SFO = CSO v. CFO; CS = FC-0 v. FC-2. Interactions of FAT or SFO with CS were not significant for any parameter reported in this table (P ≥ 0·20).
‡ Fat Ca-salts, Persiafat, Kimiya Danesh Alvand Co., contained 85 % fat and 9 % Ca (1 % C14·0, 28 % C16 : 0; 3 % C16 : 1; 5 % C18 : 0, 26 % C18 : 1, 30 % C18 : 2; 3 % C18 : 3, 4 % other).
§ Feed efficiency as calculated from dividing ADG (g) by average daily DMI. DMI includes the DM provided by the milk and starter. The DMI provided by milk was similar among treatments.

Fig. 1. Effect of prepartum dam diets (a) and calf starter (CS) diets (b) on weekly body weights (kg) of Holstein dairy calves fed CS containing no fat supplement (FC-0) or supplemented with 2 % fat Ca-salt (FC-2). Calves were born from dams fed diets supplemented with no fat (control; CON), Ca-salts of soyabean oil (CSO) or Ca-salts of fish oil (CFO) starting at 3 weeks before expected calving date. Standard errors are 0·76 and 0·62 for prepartum dam diets and CS diets, respectively. Contrast of FAT = (CSO + CFO) v. CON, SFO = CSO v. CFO and CS = FC-0 v. FC-2. Effects in model: FAT: P = 0·02; CS: P < 0·01; week: P < 0·001; dam diets × week: P = 0·03; CS × week: P < 0·001. * Difference between treatments at a given week (P ≤ 0·05). † Trend between treatments at a given week (P ≤ 0·10). (a) (), CON; (
), CSO; (
), CFO. (b) (
), FC-0; (
), FC-2.
Data for body measurements are presented in Table 5. Body length, heart girth, hip width, and body barrel were not affected by DD, CS, or their interaction. At 3 d of age, none of the parameters of structural growth was affected by diets or their interaction. Calves born from dams fed fat supplements, compared with those born from dams fed CON, had greater (P ≤ 0·05) hip (+1 and +0·8 cm, at weaning and the end of the study, respectively) and wither (+0·9 and +1 cm, at weaning and the end of the study, respectively) heights. Regardless of DD, calves fed FC-2, compared with those fed FC-0, had greater (P < 0·01) hip (+2·1 and +1·2 cm, at weaning and the end of the study, respectively) and wither (+2·0 and +1·9 cm, at weaning and the end of the study, respectively) heights.
Table 5. Effect of dietary treatments on structural growth of Holstein calves fed a calf starter (CS) containing no fat supplement (FC-0) or supplemented with 2 % fat calcium-salt (FC-2) during experimental periods
(Mean values and pooled standard errors)

CON, no fat supplement; CSO, supplementation with 140 g/d Ca-salts of soyabean oil; CFO, supplementation with 140 g/d Ca-salts of fish oil during the last 3 weeks before expected parturition.
* Calves were weaned at 56 d of age.
† P values for orthogonal contrasts: FAT = control v. CSO + CFO; SFO = CSO v. CFO; CS = FC-0 v. FC-2. Interactions of FAT or SFO with CS were not significant for any parameter reported in this table (P ≥ 0·13).
Animal behaviour
The total times devoted to different behaviours in an 8-h evaluation period are presented in Table 6. Neither DD nor CS or their interactions affected time spent eating, ruminating, standing, lying or any non-nutritive oral behaviour during the pre- or postweaning periods.
Table 6. Total time devoted to perform different behaviours during 8 h of observation of Holstein calves fed a calf starter (CS) containing no fat supplement (FC-0) or supplemented with 2 % fat calcium-salt (FC-2) in pre- and postweaning periods
(Mean values and pooled standard errors)

CON, no fat supplement; CSO, supplementation with 140 g/d Ca-salts of soyabean oil; CFO, supplementation with 140 g/d Ca-salts of fish oil during the last 3 weeks before expected parturition.
* Starter was offered from 3 d of age. Preweaning: 3–56 d. Postweaning: 57–77 d old.
† P values for orthogonal contrasts: FAT = control v. CSO + CFO; SFO = CSO v. CFO; CS = FC-0 v. FC-2. Interactions of FAT or SFO with CS were not significant for any parameter reported in this table (P ≥ 0·13).
‡ Tongue rolling, licking surfaces or eating wood shavings.
Incidence of diarrhoea and diseases
During the preweaning period, calves born from dams fed fat supplements had a slightly lower (P < 0·01) RT compared with those born from CON-fed dams (39·0 v. 39·2°C, respectively; Table 7), but the effect did not extend to the postweaning period. Regardless of DD, calves fed FC-2 had lower (P ≤ 0·04) RT compared with calves fed the FC-0 CS during both the pre- (38·97 v. 39·13°C) and postweaning (38·95 v. 39·27°C) periods. Mean scores for attitude and nasal discharge were 0·28 and 0·10 and were not affected by treatments. During the study, calves fed FC-2 had two fewer days with diarrhoea (5·6 v. 7·36 d) compared with those fed FC-0.
Table 7. Effect of dietary treatments on rectal temperature, respiratory rate, health scores, and percentage of days with poor attitude, fever, diarrhoea, and nasal discharge, faecal score and days with diarrhoea of Holstein calves fed a calf starter (CS) containing no fat supplement (FC-0) or supplemented with 2 % fat calcium-salt (FC-2) during experimental periods
(Mean values and pooled standard errors)

CON, no fat supplement; CSO, supplementation with 140 g/d Ca-salts of soyabean oil; CFO, supplementation with 140 g/d Ca-salts of fish oil during the last 3 weeks before expected parturition.
* Starter was offered from 3 d of age. Preweaning: 3–56 d. Postweaning: 57–77 d old.
† P values for orthogonal contrasts: FAT = control v. CSO + CFO; SFO = CSO v. CFO; CS = FC-0 v. FC-2. Interactions of FAT or SFO with CS were not significant for any parameter reported in this table (P ≥ 0·24).
‡ Attitude score scale: 0 = responsive, 1 = non-active, 2 = depressed, and 3 = moribund. Faeces score scale: 0 = firm faeces, no diarrhoea; 1 = soft faeces, no diarrhoea; 2 = mild diarrhoea; 3 = watery, severe diarrhoea. Nasal score scale: 0 = normal serous discharge; 1 = small amount of unilateral cloudy discharge; 2 = bilateral cloudy or excessive mucus discharge; 3 = copious bilateral mucopurulent discharge.
§ Percentage of days with health issue over a 77-d period unless indicated. Poor attitude and nasal discharge if score > 0, fever if temperature ≥ 39·5°C and diarrhoea if score > 1.
Blood metabolites
DD did not affect the mean plasma concentrations of any measured metabolite (Table 8), except cholesterol for which concentrations were greater in 28-d-old calves born from dams fed fat supplements compared with those born from dams fed CON (3·29 v. 3·14 mmol/l; P = 0·02). Mean plasma concentrations of glucose, total protein, NEFA, insulin and TAG were not different between calves fed FC-0 or FC-2 (P > 0·10). Nevertheless, calves fed FC-2 had greater (P < 0·001) concentrations of albumin (28 d: 35·2 v. 28·9 g/l and 77 d: 39·1 v. 31·9 g/l) and cholesterol (28 d: 3.30 v. 3·17 mmol/l and 77 d: 2·26 v. 2·12 mmol/l) compared with those fed FC-0. Plasma urea N at 28 and 77 d of age was lower (P < 0·001) for calves fed FC-2 compared with those fed CF-0. At the end of the study, alkaline phosphatase was greater (600 v. 537 units/l) (P < 0·001) for calves fed FC-2 compared with calves fed CF-0.
Table 8. Effect of dietary treatments on blood metabolites of Holstein calves fed a calf starter (CS) containing no fat supplement (FC-0) or supplemented with 2 % fat calcium-salt (FC-2) during experimental periods
(Mean values and pooled standard errors)
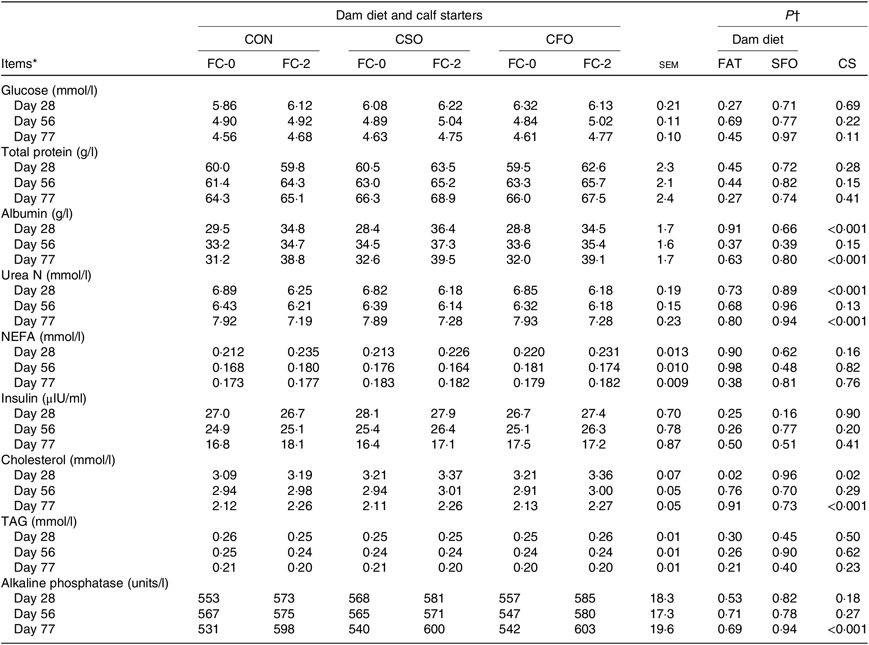
CON, no fat supplement; CSO, supplementation with 140 g/d Ca-salts of soyabean oil; CFO, supplementation with 140 g/d Ca-salts of fish oil during the last 3 weeks before expected parturition.
* Calves were weaned at 56 d of age.
† P values for orthogonal contrasts: FAT = control v. CSO + CFO; SFO = CSO v. CFO; CS = FC-0 v. FC-2. Interactions of FAT or SFO with CS were not significant for any parameter reported in this table (P ≥ 0·22).
Rumen fermentation parameters
Concentrations of ruminal pH, ammonia and individual and total VFA were not affected by DD, CS or their interactions (Table 9).
Table 9. Effect of dietary treatments on ruminal parameters of Holstein calves fed a calf starter (CS) containing no fat supplement (FC-0) or supplemented with 2 % fat calcium-salt (FC-2) during experimental periods
(Mean values and pooled standard errors)
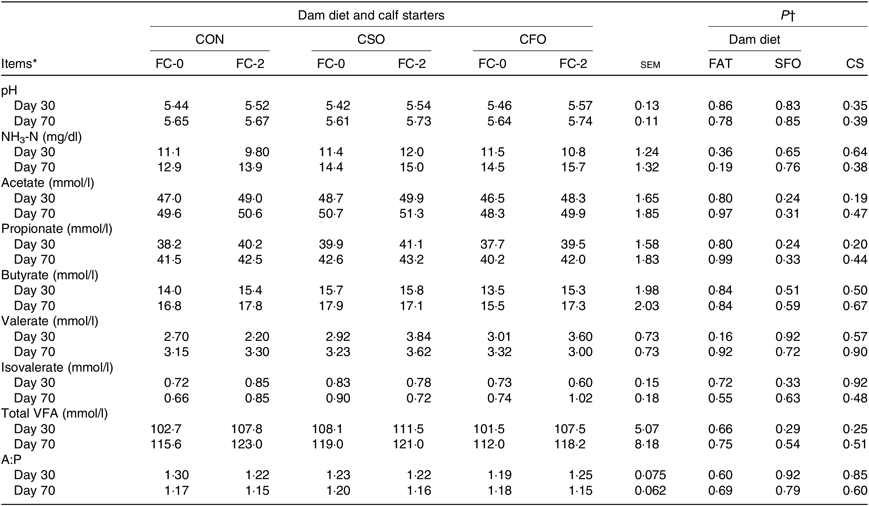
CON, no fat supplement; CSO, supplementation with 140 g/d Ca-salts of soyabean oil; CFO, supplementation with 140 g/d Ca-salts of fish oil during the last 3 weeks before expected parturition; VFA, volatile fatty acids; A:P, acetate:propionate ratio.
* Calves were weaned at 56 d of age.
† P values for orthogonal contrasts: FAT = control v. CSO + CFO; SFO = CSO v. CFO; CS = FC-0 v. FC-2. Interactions of FAT or SFO with CS were not significant for any parameter reported in this table (P ≥ 0·21).
Discussion
Effect of prepartum diets
Contrary to our hypothesis, the type of FA fed prepartum did not have an impact on calf performance. Nevertheless, the supplementation of fat regardless of the type of FA (rich in linoleic acid (CSO) or EPA and DHA (CFO)) had an impact on calf immune status and performance.
As expected, plasma concentrations of total IgG at birth were very low (≤ 0·32 g/l) and in most cases undetectable. Only one calf in the present study failed to attain at least 10 g/l IgG after colostrum feeding, and it was born from a dam fed CON. Because calves were fed colostrum from their corresponding dams, calves born from dams fed fat supplements consumed more IgG as their dams produced colostrum with higher IgG contents compared with dams fed CON(Reference Jolazadeh, Mohammadabadi and Dehghan-banadaky11). The greater IgG intake of calves born from dams fed fat supplements may have affected the observed better AEA and greater plasma IgG of those calves compared with the ones born from dams fed CON. In contrast, in beef cattle, calves suckling mature cows supplemented with oilseeds enriched in C18 : 2n-6 have similar serum IgG concentrations compared with calves suckling cows fed no oilseeds(Reference Dietz, Hall and Whittier35). Our findings support those of others who reported that calves born from dams fed fat supplements (saturated or rich in n-6 FA) tended to have greater serum concentrations of total IgG and better AEA after colostrum feeding compared with calves born from dams fed no supplemental fat(Reference Garcia, Greco and Favoreto10). Factors that may influence AEA include (1) time after birth when colostrum is fed, (2) amount of IgG fed, (3) rate of gastric emptying and (4) absorptive capacity of enterocytes for IgG(Reference Garcia, Greco and Favoreto10). In the present study, feeding time was constant and adequate. Quigley et al.(Reference Quigley, Kost and Wolfe36) recommended the ingestion of 150–200 g of IgG in the first 24 h to reduce the risk of failure of passive transfer. In the present study, calves born from dams fed fat supplements received 28 % more IgG compared with those born from dams fed CON. The present study did not aim to investigate the specific absorptive capacity of enterocytes but a potential benefit of prepartum FA feeding on changing FA profile of enterocytes’ membrane and consequently their absorptive capacity should not be ruled out.
In the present study, fat supplementation during late gestation did not affect CS intake but calves born from dams fed fat compared with those born from CON-fed dams had a better ADG preweaning, and it was maintained across the 77 d of the study. Therefore, the better BW gain observed in calves born from dams fed fat supplements resulted in a trend for an improved FE. The improved ADG and FE observed in calves born from dams fed fat may be due to meeting the metabolic demands for elongated PUFA(Reference Garcia, Shin and Schlaefli17) and may not be due to a better digestibility of nutrients, as DD did not impact apparent nutrient digestibility and VFA production. Although hip and wither heights at birth were similar among calves born from dams fed any prepartum diet, calves born from dams fed fat supplements were taller than those born from dams fed CON at weaning (56 d) and the end of the study (77 d). This delayed impact of an in utero FA exposure on growth (skeletal and BW gain) maybe reflects a better health and immune status (greater plasma IgG and lower RT during the preweaning period) of calves born from dams fed fat supplements. Although prepartum feeding did not alter birth weight, a potential epigenetic effect of DD (e.g. fat supplementation) on post-birth calf metabolism cannot be ruled out. Indeed, others have also reported this potential mechanism with strategic feeding of FA during uterine life in dairy(Reference Garcia, Greco and Lock37,Reference Garcia, Greco and Lock38) and beef cattle(Reference Marques, Cooke and Rodrigues39,Reference Funston, Larson and Vonnahme40) .
Previous studies have reported that plasma concentrations of cholesterol can be altered by fat and/or FA supplementation in humans(Reference Chan, Bruce and McDonald41), rodents(Reference Vijaimohan, Jainu and Sabitha42) and in dairy cows(Reference Drackley, Klusmeyer and Trusk43). In addition, we recently found that plasma cholesterol was greater in prepartum cows fed fat (CSO or CFO) compared with cows fed CON(Reference Jolazadeh, Mohammadabadi and Dehghan-banadaky11). In the present study, cholesterol concentrations were higher in 28-d-old calves born from dams fed fat supplements compared with those born from dams fed CON. In contrast, Garcia et al. (Reference Garcia, Greco and Favoreto13) reported that plasma concentrations of cholesterol of calves born from dams fed fat (SFA or EFA) or specific types of FA did not differ from that of calves born from dams fed no fat supplement. Researchers have reported that cholesterol is transferred across the placenta, reaches fetal circulation, and thus substantially contributes to the fetal cholesterol pool in animals and humans(Reference Woollett44,Reference Yoshida and Wada45) . Similar transfer mechanism may occur in calves born from dams fed fat supplements, and it may carry over the first weeks of life.
Effect of preweaning diets
In the present study, adding fat supplements to the CS did not affect intake of starter diets. Hill et al.(Reference Hill, Bateman and Aldrich22) reported that preweaning calves fed a starter diet containing tallow or soyabean oil had similar starter intake; however, postweaning, calves fed soyabean oil consumed less starter. These results are in accordance with Kadkhoday et al.(Reference Kadkhoday, Riasi and Alikhani46) who found that feeding starter diets with different ratios of linoleic and α-linolenic acids did not affect starter intake of Holstein dairy calves. Therefore, the better BW gain observed in the present study, without changes in starter or milk intake, resulted in an improved FE of calves fed FC-2 compared with those fed FC-0. The improved ADG and FE may be due to meeting the metabolic demands for elongated PUFA in FC-2 CS-fed calves(Reference Garcia, Shin and Schlaefli17). Similarly, Hill et al. (Reference Hill, Bateman and Aldrich21) reported that dairy calves fed starter diets supplemented with Ca-salts of flaxseed oil, rich in α-linolenic acid, had increased ADG and FE compared with non-supplemented calves.
Wither and hip heights at weaning and the end of the study were also greater for calves fed FC-2 compared with those fed FC-0. Recent studies reported that n-3 FA have a regulatory role in neonatal bone turnover in humans and different animal models(Reference Lau, Cohen and Ward47,Reference Kajarabille, Peña and Díaz-Castro48) . Kadkhoday et al.(Reference Kadkhoday, Riasi and Alikhani46) found that calves fed a lower ratio of n-6 to n-3 FA had greater hip height compared with calves fed no supplemental fat. In contrast, others have reported that preweaned calves fed n-6 FA had similar skeletal growth compared with those fed SFA(Reference Ghasemi, Azad-Shahraki and Khorvash49).
Several authors have reported that moderate concentrations of FA have beneficial effects on health and immune system function of dairy calves(Reference Garcia, Shin and Schlaefli17,Reference Ballou, Cruz and Pittroff50,Reference Hill, VandeHaar and Sordillo51) . The acute inflammatory response is protective; however, an unresolved inflammation could lead to amplified inflammation and chronic diseases. Currently, it is well-understood that an acute inflammation has a temporal peak and should be resolved to restore tissue function(Reference Serhan and Levy52). Calves fed FC-2 had lower RT and lower overall faecal scores, compared with calves fed FC-0, which resulted in those calves having a fewer number of days with diarrhoea (–2 d) compared with calves fed FC-0. These findings support the hypothesis that one or more FA provided with the CF-2 diet (e.g. oleic or linoleic acid) have an anti-inflammatory effect (lower RT) and speed up the resolution of inflammation (fewer days with diarrhoea). Others have also reported that days with abnormal faecal scores were reduced by the inclusion of different FA blends ((butyrate, coconut oil and flax oil) or (butyrate, medium-chain FA and linolenic acid)) in the MR(Reference Hill, VandeHaar and Sordillo51,Reference Esselburn, O’Diam and Hill53) . Similarly, others have reported a reduction in faecal scores when feeding starters with flaxseed oil or FA blend (coconut oil and canola oil) with the MR(Reference Hill, Aldrich and Schlotterbeck20,Reference Kadkhoday, Riasi and Alikhani46) . These beneficial effects of health-related parameters observed in calves fed FC-2 may have elicited a positive impact on their growth rate and FE.
Dairy cattle responses to different feed ingredients and feeding strategies differ. Indeed, it has been reported that fat content can affect eating behaviour(Reference Albright54). In the present study, animal behaviour was similar across treatments, and all calves spent similar times eating, ruminating, standing, lying or non-nutritive oral behaviour during both pre- and postweaning periods. To the best of our knowledge, animal behaviour has not been measured in experiments where fat supplements were fed in utero and preweaning and early postweaning simultaneously. Nevertheless, Kadkhoday et al.(Reference Kadkhoday, Riasi and Alikhani46) reported that adding palm fat powder or flaxseed oil or both to starter diets had no adverse effect on the feeding behaviours of Holstein calves during pre- and postweaning periods. Furthermore, Benson et al.(Reference Benson, Reynolds and Humphries55) reported that dairy cows infused, via the abomasum, with a mixture of sunflower and rapeseed oils did not alter their daily time spent engaged in eating, ruminating and idling activities. Similarly, McDonnell et al.(Reference McDonnell, O’Doherty and Earley56) found that feeding behaviour of bull calves was not altered with fish oil supplementation during the preweaning period.
Studies have reported that strategic feeding of fat and FA alter the plasma metabolic profile of supplemented animals(Reference Garcia, Greco and Favoreto13,Reference Garcia, Shin and Schlaefli17) and the present findings are in support of those studies. Indeed, the feeding of a CS rich in unsaturated FA (FC-2) compared with a starter without supplemental fat (FC-0) altered the plasma metabolic profile of calves. The liver plays a key role in nutrient metabolism and utilisation due to its strategic position of integration within the circulatory system(Reference Garcia, Greco and Lock38). Garcia et al.(Reference Garcia, Greco and Lock38) reported that calves fed diets rich in n-6 compared with SFA up-regulated the expression of hepatic genes involved in protein synthesis. In the present study, calves fed FC-2, compared with those fed FC-0, had higher plasma albumin concentrations, which may suggest a better hepatic function. Similarly, the addition of an FA blend to MR showed an increase in serum albumin concentration(Reference Esselburn, O’Diam and Hill53). The blood urea N-reducing effect of feeding FC-2 compared with FC-0 may be due to an improvement in protein deposition and reduction in protein oxidation, which is supported by higher concentrations of albumin and better ADG and skeletal growth of calves fed FC-2. Moreover, Le Roith et al.(Reference Le Roith, Hammond and Todd57) reported that lower urea N could be related to changes in protein synthesis and protein oxidation, which are confirmed by our present findings.
Cholesterol is synthesised primarily in the liver of preruminant calves as products of lipid metabolism(Reference Drackley, Burrin and Mersmann58). The observed higher concentrations of cholesterol in plasma of calves fed FC-2 may simply reflect the preferential esterification of cholesterol esters with high intake of unsaturated FA compared with that of calves fed FC-0. Previous studies in preweaned calves reported different impacts of FA supplemented with MR, i.e. a linoleic acid-enriched MR reduced plasma cholesterol concentrations compared with a lauric acid-enriched MR(Reference Garcia, Greco and Favoreto13), whereas a soyabean oil-enriched MR quadratically increased plasma concentrations of cholesterol(Reference Garcia, Shin and Schlaefli17).
Hill et al.(Reference Hill, Aldrich and Schlotterbeck59,Reference Hill, Aldrich and Schlotterbeck60) reported that the increase in ALP is a good index of bone formation in young calves. Also, other researchers reported that adding flaxseed oil in the starter diet increased ALP(Reference Hill, Bateman and Aldrich21,Reference Woollett44) . In our study, plasma concentrations of ALP were greater in 77-d-old calves fed FC-2, compared with those fed CF-0. The use of ALP as an index of bone formation is supported by our findings of better skeletal growth (hip and wither heights) of calves fed FC-2 compared with those fed CF-0.
Several authors have reported that high amounts of PUFA in the rumen of mature cattle have adverse effects on micro-organism function and led to disturbed ruminal fermentation(Reference Liu, Bu and Wang61,Reference Ivan, Petit and Chiquette62) . The lack of effect of CS on rumen fermentation parameters observed in the present study supports that of Kadkhoday et al.Reference Kadkhoday, Riasi and Alikhani(46) who reported that CS supplemented with low levels of palm or flaxseed oil (less than 3 % starter diet) had no adverse effect on ruminal fermentative parameters. On the other hand, Ghasemi et al.(Reference Ghasemi, Azad-Shahraki and Khorvash49) reported that feeding a starter diet with different types of fats had minor impacts on rumen fermentation parameters, e.g. ruminal pH, ammonia, or total VFA concentration were not altered. However, rumen fluid from calves fed a mixture of fats (palm fat, soybean oil and fish oil), compared with calves fed no fat or a single fat source, had increased molar concentrations of propionate and lower acetate:propionate ratio in ruminal fluid of 35-d-old Holstein calves(Reference Ghasemi, Azad-Shahraki and Khorvash49). Furthermore, preweaned dairy calves fed a low-fat starter (2·9 %) tended to have a reduction in the molar proportion of rumen butyrate but not in the other VFA(Reference Kazemi-Bonchenari, Mirzaei and Jahani-Moghadam63). The mean ruminal pH values were within normal physiological values and ranged from 5·4 to 6·2, reflecting no adverse effect of diets on ruminal fermentation. Others also reported that extruded full-fat soyabeans did not alter ruminal pH in dairy calves(Reference ZeidAli-Nejad, Ghorbani and Kargar64). In the present study, ether extract content in CF-2 (rich in palmitic, oleic and linoleic acids) was 5·1 % compared with 3·35 % in CF-0 and these differences did not alter ammonia, specific VFA, or total VFA concentrations, which may suggest that cellulolytic bacterial populations were not affected by the difference in ether extract content and FA profile.
Finally, as consumer awareness for nutraceutical food has risen, nutritionists should focus on strategic feeding aiming to produce food from animal origin rich in nutrients conferring health benefits. Indeed, n-3 and n-6 FA are well known to have an impact on human health and incidence of chronic diseases. Therefore, feeding PUFA to dairy cattle will not only benefit the performance of supplemented animals but their food products (e.g. milk, meat) would also have a FA profile with beneficial effects on human health.
Conclusions
Strategic feeding of diets enriched in PUFA (n-6 or n-3 FA) to pregnant non-lactating dams (1·15 % of dietary DM) or a starter rich in unsaturated FA to preweaned Holstein calves (2 % of CS DM) impacted calf performance. Prepartum supplementation of fat improved calves’ passive immunity, overall ADG, weaning weight, final weight, hip height and wither height of calves without changing DMI. However, prepartum diets did not influence the effect of CS on calf performance or other measured parameters in the present study. Calves fed a CS enriched in unsaturated FA compared with non-supplemented calves had better ADG and FE, greater hip height, lower RT, fewer days with diarrhoea and higher plasma albumin and ALP. Further research is needed to increase the understanding of the effects of fat supplemented during the last weeks of uterine life and preweaning period on inflammatory status and response of immune cells of preweaned calves.
Acknowledgements
This research was supported by funds from the Agricultural Sciences and Natural Resources University of Khuzestan and University of Tehran. The authors thank the manager (Dr M. A. Moshayedi) and staff of the Zarin Khoshe dairy farm in the central part of Iran (Arak Province).
The authors’ contributions were as follows: T. M., M. D., M. G. and M. C. designed the experiment; A. R. J. performed the research, laboratorial analyses and analysing the data. A. R. J. and M. G. wrote the manuscript. All authors reviewed and edited the manuscript.
The authors declare that there are no conflicts of interest.