Since its discovery in the 1930s, the importance of vitamin K in physiological processes other than blood coagulation has become increasingly clear. Several studies in adults suggest a beneficial role for vitamin K in bone mineral metabolism and bone fracture prevention, although precise mechanisms have not been elucidated(Reference Cockayne, Adamson and Lanham-New1–Reference Feskanich, Weber and Willett3). A well-recognised concept is the vital role of vitamin K as a cofactor in the post-translational γ-carboxylation of osteocalcin, a protein synthesised by osteoblasts(Reference Shearer4, Reference Vermeer, Shearer and Zittermann5). In this carboxylation process, glutamate residues are converted into γ-carboxyglutamate (Gla)(Reference Wallin, Sane and Hutson6). These Gla residues have a high affinity for Ca (Ca2+) which is an essential property of all Gla proteins. A poor vitamin K status will lead to production of undercarboxylated (i.e. inactive) osteocalcin (ucOC)(Reference Koshihara and Hoshi7). In the healthy adult population, osteocalcin is carboxylated to a variable extent, suggesting that the dietary vitamin K intake is often insufficient for full osteocalcin carboxylation(Reference Binkley, Krueger and Engelke8). Previous studies in postmenopausal women found a clear association between elevated ucOC and increased fracture risk(Reference Szulc, Chapuy and Meunier9–Reference Vergnaud, Garnero and Meunier11).
Recently, we reported that the majority of healthy children aged between 6 and 18 years have high circulating levels of ucOC compared with adults(Reference van Summeren, Braam and Noirt12). In addition, the amount of ucOC relative to the carboxylated fraction of osteocalcin (cOC) was also high in these children. This ucOC:cOC ratio (UCR) is used as an indicator for the vitamin K status(Reference Vermeer, Shearer and Zittermann5, Reference Sokoll and Sadowski13). An elevated UCR is indicative of an inferior vitamin K status of bone and relates to lower nutritional vitamin K intakes(Reference Binkley, Krueger and Engelke8, Reference Sokoll and Sadowski13). O'Connor et al. reported that in healthy girls aged 11–12 years, better vitamin K status was associated with increased bone mineral content(Reference O'Connor, Molgaard and Michaelsen14). In an observational study in healthy peripubertal children, we showed that improvement of vitamin K status over 2 years was associated with a marked increase in bone mineral density(Reference Van Summeren, Van Coeverden and Schurgers15).
These findings justify clinical intervention studies in children in whom bone quality is monitored as a function of long-term vitamin K supplementation. Before undertaking such long-term intervention studies, the effect of increased vitamin K intake in healthy children on osteocalcin carboxylation has to be established. Intervention studies in adults have already demonstrated that markedly higher osteocalcin carboxylation is obtained by intakes of vitamin K well above the current recommended dietary intake(Reference Binkley, Krueger and Kawahara16, Reference Booth, Martini and Peterson17). The two most important forms of vitamin K are phylloquinone (vitamin K1) and the group of K2 vitamins (menaquinones; MK-n). A recent report by Schurgers et al. showed that MK-7, a form of vitamin K2 which is particularly abundant in fermented soyabeans (natto), has possible advantages in comparison with phylloquinone, largely due to its long half-life time(Reference Schurgers, Teunissen and Hamulyak18). In this intervention study, adult volunteers received a daily dose of equimolar amounts (0·22 μm) of either MK-7 (150 μg) or phylloquinone (100 μg) during 6 weeks. The dosage of 100 μg phylloquinone was chosen based on the median intakes of vitamin K in adults. The extent of osteocalcin carboxylation was significantly higher in the MK-7 group compared with the K1 group. At the end of the intervention period, a still-increasing carboxylation of osteocalcin was seen in the MK-7 group, whereas the K1 group had already reached a plateau phase.
The relationship between increased MK-7 intake and enhanced osteocalcin carboxylation has never been shown in healthy children. It is reasonable to infer that MK-7 will improve osteocalcin carboxylation in children but to what extent is unclear. Therefore, we set up a randomised controlled trial in healthy children using an MK-7-containing food supplement to study its effects on the circulating levels of ucOC and cOC in healthy prepubertal children. We hypothesised that MK-7 supplementation reduces the UCR, indicating an improved vitamin K status. To exclude a potential effect of high vitamin K on haemostasis, the highly sensitive endogenous thrombin potential was monitored in all samples at baseline and at the end of the study.
Subjects and methods
Study design
The present study is a double-blind randomised placebo-controlled intervention study. Subjects were recruited from two primary schools around Utrecht, the Netherlands. Inclusion criteria were apparently healthy prepubertal children aged between 6 and 10 years, normal (3rd to 97th percentile) height and weight according to standard growth charts and parents giving written consent for the child to take part in the study. We chose this age category because these children are most likely to be in a prepubertal state when bone turnover is fairly stable. In this way, we were able to study the effect of vitamin K on osteocalcin carboxylation without possibly confounding effects from rapid changes in bone metabolic activity which may occur during puberty and may influence vitamin K status(Reference van Summeren, Braam and Noirt12, Reference Kalkwarf, Khoury and Bean19). Exclusion criteria were current or previous medical diseases such as metabolic or gastrointestinal disease, soya allergy, chronic inflammatory diseases and current use of systemic corticoid treatment, vitamin supplements containing vitamin K or oral anticoagulants. The exclusion criterion ‘soya allergy’ was introduced because the vitamin K-containing capsules used in the study may contain traces of soya proteins originating from the natto extract. The study protocol was approved by the Central Committee on Research involving Human Subjects (CCMO; The Hague, the Netherlands).
Subjects and study procedures
The total eligible group consisted of 240 children. A total of 55 children met the criteria for participation, and were included in the present study. Enrolment took place in January 2007. One investigator was responsible for the intake of participants and supervision of the study. Participants were randomised into two groups by computer-generated random permutation procedures: those receiving a placebo (n 27) and those receiving a supplement containing 45 μg MK-7 (n 28) during 8 weeks. Based on previous observations from studies in adults in whom the maximal effect on osteocalcin carboxylation is seen after an intervention period ranging from 3 to 8 weeks, we chose an intervention period of 8 weeks(Reference Binkley, Krueger and Kawahara16, Reference Booth, Martini and Peterson17). The randomisation schedule was generated by an investigator not involved in the coordination of the study and kept apart during the trial. A block randomisation was performed for sex, in order to avoid unequal distribution of boys and girls in the two intervention groups.
After randomisation, subjects and their parents were invited for a study visit in which the study capsules were handed out. Participants were instructed to take one capsule per d during 8 weeks during the main (evening) meal. During this visit, a short physical examination was performed and blood was taken by venepuncture. Furthermore, body height and weight were measured in a standardised manner without shoes and heavy clothing, to the nearest 1 cm and 100 g, respectively. From these values, the BMI (weight (m)/height (kg)2) was calculated. The values of height, weight and BMI were compared with reference values for healthy subjects matched for age and sex, and standard deviation scores were calculated(Reference Frederiks, van Buuren and Burgmeijer20). Participants and parents were advised to continue their usual activities and maintain their normal diets during the study period. Co-medication was allowed with the exception of other vitamin supplements, oral anticoagulants and corticosteroid treatment. At 2, 4 and 6 weeks, short phone calls were scheduled to inform about the occurrence of adverse events and possible side-effects of the study capsules. At 8 weeks, the final visit was planned in which the second venepuncture was performed. Compliance was monitored using a calendar on which the daily intake of the study supplement had to be recorded. Also, the parents were requested to hand in the remaining capsules and capsule container at the end of the study.
Intervention
Placebo- and vitamin K-containing capsules (MenaQ7) provided by NattoPharma (Oslo, Norway) were similar in taste and appearance, and were thus indistinguishable for participants and investigators. Both capsules contained equal amounts of linseed oil (210 mg). The dosage of 45 μg and explicit choice for the vitamer MK-7 are based on results from a previous study in which the effects of MK-7 on bioavailability and osteocalcin carboxylation were compared with phylloquinone(Reference Schurgers, Teunissen and Hamulyak18). In this study, a dosage of 150 μg MK-7 was used for adults (estimated weight 80 kg). This dosage was calculated to the body weight of children (aged 6–10 years; estimated average weight 25 kg), leading to a dosage of 45 μg MK-7 for children.
Collection and preparation of samples
Non-fasting blood samples were obtained in fifty-three children at baseline and at the end of the study after 8 weeks. For each child the two venepunctures were performed at the same time of the day, i.e. morning or afternoon. After blood sampling and serum preparation, all samples were frozen and kept at − 80°C until use.
Experimental techniques
Serum carboxylated and undercarboxylated osteocalcin
ucOC and cOC were measured in serum by ELISA (Takara Shuzo Co. Ltd, Shiga, Japan). Levels of MK-7 were measured in serum by HPLC as described previously by Schurgers & Vermeer(Reference Schurgers and Vermeer21).
Bone markers
To confirm the stability of bone metabolism over the study period, two biochemical markers of bone turnover were measured. Bone alkaline phosphatase (Hybritech, Liège, Belgium) was measured as a marker of bone formation by RIA. As a marker of bone resorption, N-telopeptide cross-links of collagen breakdown (Ostex International, Inc., Seattle, WA, USA) were determined.
Coagulation parameters
The hypothesis that supplementation of MK-7 in healthy children has no procoagulant effect and is therefore safe was tested by monitoring the course of thrombin in time as described in detail by Hemker & Beguin(Reference Hemker and Beguin22). By this method two variables are determined: the endogenous thrombin potential, representing the total amount of thrombin which can be generated in plasma through the extrinsic pathway, and the thrombin maximal peak height, representing the maximal thrombin concentration reached after activating the coagulation cascade. The principle of the technique is that a fluorogenic substrate is added to clotting plasma, whereby the thrombin formed converts the substrate into a fluorophore, the signal of which is measured continuously by a fluorometer; the signal is than calibrated using the thrombin calculator.
All samples were analysed using the same assay lot run to reduce inter-assay variation.
Outcome parameters and power calculation
The primary outcome of the study was the percentages of change in UCR from baseline (0 weeks) to endpoint (8 weeks) in both treatment groups. Secondary outcomes were the percentage of change in the absolute concentrations of circulating ucOC, cOC and MK-7. The desired effect of the vitamin K treatment in the present study was to reduce the UCR in children to the average level of young adults. The expected difference in UCR between the treatment and control group is based on reference values found in a previous study in which the average UCR level was 0·8 in young adults and 2·3 in children(Reference van Summeren, Braam and Noirt12). The sample size was calculated on the assumption that the expected effect was a minimum reduction of 20 % in UCR from baseline to endpoint (8 weeks) in the treatment group compared with the placebo group with a 90 % power and a 0·05 level of significance. With the assumption of a drop-out rate of 10 % over the total study period, we calculated that at least twenty-five subjects had to be included in each group.
Statistical methods
Normality of distribution for all subjects was checked for all study parameters using histograms. Normally distributed baseline characteristics of both the placebo and treatment groups were compared using independent t tests. A χ2 test was performed to compare the distribution of sex across groups. Differences at baseline as well as percentage of change in ucOC, cOC and UCR from baseline to endpoint between groups were assessed using Mann–Whitney tests. In the placebo and treatment groups separately, differences in ucOC, cOC and UCR from baseline to follow-up were examined using Wilcoxon signed-ranks tests for paired variables. Statistical analyses were performed using SPSS Base 12.0.2 for Windows (SPSS, Inc., Chicago, IL, USA) with two-sided significance levels of 5 %.
Results
Figure 1 shows the flowchart for treatment of participants in the study. All included children completed the intervention period of 8 weeks. However, we had missing data in several children because the venepuncture failed and no or insufficient blood could be drawn.

Fig. 1 Flow chart of participants in the VitaKids study. * There were missing laboratory data because venepuncture failed or insufficient blood was gained in subjects who completed the total study.
The majority of children (66·7 % of the placebo group and 71·4 % of the treatment group) reported to have never missed their daily capsule. In the placebo group, nine children missed on average two capsules (range one to three) during the study period. In the treatment group, eight children missed on average three capsules (range one to seven) during the study period. The compliance as reported on the calendar by the children was in accordance with the remaining capsules in the containers, and confirmed by the parents. No side-effects were reported during the study.
The baseline characteristics for the placebo and treatment groups are shown in Table 1. The groups were comparable in age, height, weight, BMI and distribution of sex. Markers representing the vitamin K status of bone (UCR, ucOC and cOC) were also similar across groups. Also, the level of MK-7 was comparable between the placebo and the vitamin K groups. A large range of ucOC, cOC and the UCR was found in both groups at baseline.
Table 1 Baseline characteristics of the placebo and vitamin K groups
(Mean values and standard deviations or median values and ranges)
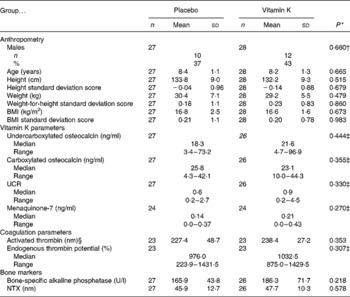
UCR, undercarboxylated osteocalcin:carboxylated osteocalcin ratio; NTX, N-telopeptide cross-links of collagen breakdown.
* P values are presented for differences in variables between placebo and vitamin K groups at baseline. P values are based on independent t tests.
† χ2 Test.
‡ Mann–Whitney test.
§ Peak height activated thrombin in the thrombogram.
Figure 2 shows the absolute values of ucOC, cOC, UCR and MK-7 at baseline and after 8 weeks in the placebo and treatment groups. Within the treatment group, ucOC and UCR decreased significantly from baseline to endpoint. The cOC increased slightly but this was a near-significant increase, whereas the level of MK-7 increased significantly. The increased level of MK-7 in the vitamin K group also demonstrates the compliance rate with the treatment.

Fig. 2 Vitamin K parameters at baseline and after 8 weeks in the placebo and vitamin K groups. Differences from baseline to follow-up in each group were examined using Wilcoxon signed-ranks tests. (a) Carboxylated osteocalcin. The differences from baseline to follow-up in the placebo and vitamin K groups were NS (P = 0·657 and P = 0·067 respectively). (b) Undercarboxylated osteocalcin. The difference from baseline to follow-up in the placebo group was NS (P = 0·228). The difference from baseline to follow-up in the vitamin K group was significant (P < 0·001). (c) Undercarboxylated osteocalcin:carboxylated osteocalcin ratio (UCR). The difference from baseline to follow-up in the placebo group was NS (P = 0·451). The difference from baseline to follow-up in the vitamin K group was significant (P < 0·001). (d) Serum menaquinone-7 (MK-7). The difference from baseline to follow-up in the placebo group was NS (P = 0·244). The difference from baseline to follow-up in the vitamin K group was significant (P < 0·001).
Within the placebo group, ucOC, cOC and UCR did not change over time. In Table 2, the median and range of the percentages of change (%Δ) in ucOC, cOC, UCR and MK-7 from baseline to endpoint in the placebo and treatment group are shown. The %Δ in ucOC, UCR and MK-7 in the treatment group was significantly different from the placebo group whereas the difference in %Δ in cOC between groups was nearly significant.
Table 2 Changes in vitamin K parameters and other parameters in placebo and vitamin K groups*
(Median percentage changes from baseline to follow-up at 8 weeks and ranges)
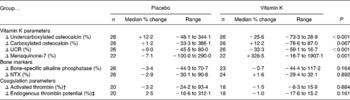
UCR, undercarboxylated osteocalcin:carboxylated osteocalcin ratio; NTX, N-telopeptide cross-links of collagen degradation.
* A positive value indicates an increase from baseline to endpoint; a negative value indicates a decrease. P values are presented for differences in the percentage of change in variables between placebo and vitamin K groups. P values are based on based on Mann–Whitney tests.
† Peak height activated thrombin in the thrombogram.
‡ Area under the curve in the thrombogram.
The median and range of the percentages of change in non-vitamin K-dependent variables are also shown in Table 2. No significant differences were observed for any of the parameters measured.
Discussion
The present study shows that supplementation with 45 μg MK-7 during 8 weeks in healthy prepubertal children improves vitamin K status as indicated by the UCR. In the MK-7-supplemented group, the circulating concentration of the inactive fraction of osteocalcin, ucOC, decreased. Furthermore, no increase of procoagulant activity was noted in the treatment group, indicating that supplementation with MK-7 is safe. The present study represents the first randomised controlled trial using MK-7 and its effect on osteocalcin carboxylation in healthy children.
Until now, only three studies in children, all paediatric patients, have reported on vitamin K supplementation and all three showed a positive effect on osteocalcin carboxylation(Reference Nicolaidou, Stavrinadis and Loukou23–Reference Panis, Vermeer and van Kroonenburgh25). In a 4-week cross-over study in eighteen cystic fibrosis patients (aged 13–35 years) using 5 mg phylloquinone weekly, Beker et al. noted an increase in serum ucOC when patients were off supplementation(Reference Beker, Ahrens and Fink24). Nicolaidou et al. studied twenty cystic fibrosis patients (aged 6–17 years) who received 10 mg phylloquinone per week during 1 year and they reported a decrease in ucOC concentrations that was even under the ucOC level of twenty-five healthy controls (aged 8–17 years)(Reference Nicolaidou, Stavrinadis and Loukou23). Findings from a randomised controlled trial in forty children with classical galactosaemia (aged 3–18 years) by Panis et al. showed a significant increase in cOC and decrease in ucOC after 1 year in children receiving a daily dose of 1·0 mg phylloquinone together with Ca and vitamin D3(Reference Panis, Vermeer and van Kroonenburgh25). The dosage of phylloquinone used in these studies is quite high, probably because these children had an established risk of (subclinical) vitamin K deficiency due to malabsorption associated with their underlying condition.
In the present study, we used a supplement containing an amount of vitamin K approximating the median intakes for adults, in addition to habitual dietary vitamin K intakes. At present, the estimated average requirement of 30–75 μg vitamin K/d for children (1–18 years) in the USA is based on the median intakes of vitamin K in adults(26). These intakes are aimed at the maintenance of normal concentrations of the hepatic vitamin K-dependent protein prothrombin, and not of the other vitamin K-dependent proteins (for example, osteocalcin). Thus, dietary vitamin K levels that are sufficient to maintain the hepatic production of normal blood coagulation factors may be too low for optimal carboxylation of the extra-hepatic Gla proteins(Reference Sokoll and Sadowski13). In the present study, levels of prothrombin and endogenous thrombin potential in both groups did not change over the study period, indicating sufficient habitual dietary vitamin K intake for the generation of normal blood coagulation factors in the liver. The absolute amount of circulating ucOC is generally used as a marker of extrahepatic vitamin K status, but is mainly determined by two factors: bone turnover and bone vitamin K stores(Reference Sokoll and Sadowski13, Reference Collins, Cashman and Kiely27, Reference Knapen, Hamulyak and Vermeer28). Vitamin K status in children is preferably based on the UCR or the quantity of ucOC relative to the total osteocalcin, in order to minimise possible influences of bone metabolism(Reference van Summeren, Braam and Noirt12, Reference Kalkwarf, Khoury and Bean19). In healthy adult volunteers, vitamin K supplementation reduced the circulating level of ucOC and increased the level of cOC(Reference Binkley, Krueger and Engelke8, Reference Binkley, Krueger and Kawahara16, Reference Knapen, Hamulyak and Vermeer28, Reference Sokoll, Booth and O'Brien29). Also in the present study, vitamin K supplementation resulted in reduction of ucOC levels and improved UCR. Until recently, non-carboxylated osteocalcin was presumed to be a non-functional protein since its Ca-binding Gla residues are lacking. A study conducted by Lee et al. demonstrated a possible novel function for non-carboxylated osteocalcin, namely its action as a hormonal regulator of energy metabolism(Reference Lee, Sowa and Hinoi30). However, these findings from in vitro studies have not yet been confirmed in human subjects.
The children included in the present intervention study had a better vitamin K status at baseline in comparison with the children from another study in healthy children conducted by our department(Reference van Summeren, Braam and Noirt12). In the latter study, a pronounced elevation (as compared with adults) of ucOC together with a marked elevation of the UCR was found in the majority of children. The UCR was on average 2·3 (range 0·3–6·8) in children v. 0·8 (range 0·1–2·2) in adults. In the present study, only six children (11 %) had a UCR above 2·3 at baseline in comparison with 35 % of children from the previous cohort. This observation can be explained by the fact that in the present study we examined vitamin K supplementation in prepubertal children with steady bone metabolic activity, as shown by constant levels of bone markers in the present study. As was shown in previous studies in children, suboptimal vitamin K status is most evident in puberty when the bone metabolic activity is high, leading to increased vitamin K demand(Reference van Summeren, Braam and Noirt12, Reference Van Summeren, Van Coeverden and Schurgers15). The present study illustrates that vitamin K supplementation has an effect on osteocalcin carboxylation, even in prepubertal children in the lowest UCR ranges.
Studies in the elderly population indicate that supplementation with vitamin K has a beneficial effect on bone mass and bone strength, most probably by improved carboxylation of osteocalcin(Reference Cockayne, Adamson and Lanham-New1, Reference Price, Williamson and Lothringer31). A growing body of evidence on the relationship between vitamin K, osteocalcin carboxylation and bone health is also emerging in healthy children. O'Connor et al. demonstrated that better vitamin K status, expressed as percentage ucOC, was associated with increased bone mineral content in a large cohort of healthy girls aged 11–12 years(Reference O'Connor, Molgaard and Michaelsen14). Although both phylloquinone and menaquinones serve as cofactors for the carboxylation of osteocalcin(Reference Schurgers, Teunissen and Hamulyak18), menaquinones (notably MK-4 and MK-7) may be preferable in food supplements because of their pleiotropic effects on bone(Reference Shearer and Newman32). In vitro models have shown that MK-7 affects the function of the osteoblasts by inducing the expression of osteoblast-specific genes (for example, osteocalcin, osteoprotegerin, receptor activator of NF-κB (RANK) and RANK ligand (RANKL)). In addition, MK-7 promotes the differentiation of the osteoblasts, probably by interaction with the steroid and xenobiotic receptor (SXR)(Reference Tabb, Sun and Zhou33, Reference Katsuyama, Otsuki and Tomita34). Furthermore, MK-7 prevented ovariectomy-induced bone loss in rats(Reference Yamaguchi, Kakuda and Gao35, Reference Yamaguchi, Taguchi and Gao36).
A limitation of the present study may be that it was not designed as a dose-finding study. The results only indicate that MK-7 improves osteocalcin carboxylation in healthy children and can therefore not answer the question ‘What is the amount of vitamin K that is needed for maximal or optimal carboxylation of osteocalcin in children?’. Another limitation is that we did not collect information on vitamin K intake from habitual diet. However, FFQ are not mandatory to study the effect of additional vitamin K on osteocalcin carboxylation when the study is designed as a randomised placebo-controlled trial.
In conclusion, the present study indicates that supplementation with MK-7, one of the vitamin K2 species, during 8 weeks reduces the amount of circulating ucOC and thereby improves vitamin K status in healthy prepubertal children. Additional dose-finding studies are needed to investigate optimal osteocalcin carboxylation in children. Thereafter, large longitudinal supplementation paediatric trials are required to confirm the beneficial effect of vitamin K supplementation via osteocalcin carboxylation on improved bone mass and diminished fracture risk.
Acknowledgements
The authors thank Kirsten Teunissen for her excellent laboratory work and Ilse Binnekamp for her indispensable assistance in the practical execution of the study. All authors contributed to the interpretation of data and writing of the article and participated in the design of the study.
The present study was supported by non-restrictive grants from NattoPharma ASA and the Research Fund of the Wilhelmina Children's Hospital (University Medical Centre (UMC) Utrecht).
The authors declare that they have no conflicts of interest.