Enterotoxigenic Escherichia coli (ETEC) K88 is a major cause of diarrhoea in neonates and recently in weaned pigs( Reference Nagy and Fekete 1 , Reference Francis 2 ). It results in reduced growth rate, increased morbidity and mortality, and great economic loss in pig production worldwide( Reference Madec, Bridoux and Bounaix 3 , Reference Fairbrother, Nadeau and Gyles 4 ). Post-weaning (PW) diarrhoea due to ETEC K88 generally occurs in pigs 3–10 d PW( Reference Carstensen, Ersbøll and Jensen 5 , Reference Lallès, Bosi and Smidt 6 ). ETEC K88 colonises in the small intestine and releases specific enterotoxins that impair intestinal barrier function, which indirectly induces fluid losses( Reference Fairbrother, Nadeau and Gyles 4 – Reference Lallès, Bosi and Smidt 6 ). Several factors, such as stress of weaning, lack of milk and dietary changes, contribute to the severity of the disease. Furthermore, weaning is often associated with undesirable morphological and physiological changes in the gastrointestinal (GI) tract that is related to a reduced voluntary feed intake and, subsequently, susceptibility to intestinal health imbalance( Reference Pluske, Hampson and Williams 7 , Reference Lallès, Boudry and Favier 8 ). The ban on the inclusion of antibiotic growth promoters in weaning pig diets in Europe (European Community Regulation no. 1831/2003) has been associated with an increase in diarrhoea, weight loss and mortality due to E. coli in pigs PW( Reference Casewell, Friis and Marco 9 ). Traditional measures to ameliorate or reduce PW disorders have centred on dietary manipulations PW( Reference Pierce, Sweeney and Brophy 10 – Reference Heim, Walsh and Sweeney 13 ). However, recent research has indicated that maternal dietary supplementation with seaweed extracts (SWE) containing laminarin and fucoidan has beneficial effects on weaning-associated intestinal dysfunction and growth depression immediately after weaning( Reference Leonard, Sweeney and Bahar 14 ). Furthermore, pigs weaned from SWE-supplemented sows had a higher average daily gain (ADG) between days 0 and 21 PW compared with those weaned from untreated sows( Reference Leonard, Sweeney and Bahar 14 ).
Experiments on the effect of maternal dietary supplementation with SWE on growth performance and indicators of GI health of weaned piglets after challenge with ETEC K88 PW are limited. The objective of the present study was to investigate the effect of maternal supplementation with SWE from day 83 of gestation until weaning (day 28) on pig growth performance, faecal score, ETEC K88 toxin quantification, intestinal architecture and gene expression profiles of cytokines of both unchallenged and ETEC K88-challenged weaned piglets. The hypothesis of the present study is that maternal supplementation with SWE would enhance the growth performance and improve the aspects of GI health and immune status of weaned piglets, making them more immune competent to deal with the PW adversities, represented in the present experiment by an ETEC K88 challenge.
Materials and methods
All procedures described in the present experiment were conducted under experimental license from the Irish Department of Health in accordance with the Cruelty to Animals Act 1876 and the European Communities (Amendments of the Cruelty to Animals Act, 1876) Regulations.
Experimental design and animal diets – gestation and lactation periods
A total of twenty cross-bred pregnant gilts (Large White × Landrace genetic lines, Hermitage) were assigned to one of two dietary treatments (n 10 sows per treatment): basal gestation/lactation diet (T1) and basal gestation/lactation diet supplemented with 10·0 g SWE/d (T2) from day 83 of gestation until weaning (day 28). The quantity of SWE (BioAtlantis Limited) used in the present study was based on previous work by Leonard et al. ( Reference Leonard, Sweeney and Bahar 14 ). The SWE supplement (10·0 g/d) contained laminarin (1·0 g), fucoidan (0·8 g) and ash (8·2 g), and was extracted from a Laminaria spp. according to the procedure described by Lynch et al. ( Reference Lynch, Sweeney and Callan 15 ). The gestation diet was formulated to contain 140 g/kg of crude protein, 13·0 MJ/kg of digestible energy and 5·5 g/kg of total lysine. The lactation diet was formulated to contain 190 g/kg of crude protein, 14·5 MJ/kg of digestible energy and 10·0 g/kg of total lysine. All amino acids requirements were met relative to lysine( 16 ). The ingredients and chemical analysis of the experimental diets are presented in Table 1.
Table 1 Ingredients and chemical analysis of the experimental diets (g/kg, unless otherwise indicated)

DE, digestible energy.
* T1, basal diet; T2, basal diet supplemented with 10·0 g seaweed extract/d.
† Gilt/sow diet provided (per kg diet): 250 mg choline chloride; 140 mg Fe; 120 mg Zn as ZnO; 67 mg α-tocopherol; 47 mg Mn as MnO; 25 mg Cu as CuSO4; 12 mg nicotinic acid; 10 mg pantothenic acid; 4 mg phytylmenaquinone; 2 mg riboflavin; 2 mg thiamin; 1·8 mg retinol; 0·6 mg iodine as calcium iodate on a calcium sulphate/calcium carbonate carrier; 0·3 mg Se as sodium selenite; 0·025 mg cholecalciferol; 0·01 mg cyanocobalamin; 0·015 mg pyridoxine.
‡ Weaner diet provided (per kg diet): 100 mg Zn as ZnO; 40 mg α-tocopherol; 25 mg Mn as MnO; 25 mg Cu as CuSO4; 0·3 mg retinol; 0·2 mg iodine as calcium iodate on a calcium sulphate/calcium carbonate carrier; 0·3 mg Se as sodium selenite; 0·05 mg cholecalciferol.
§ Calculated from amino acid and DE values of ingredients( Reference Sauvant, Perez and Tran 46 ).
Animals and management
Before day 83 of gestation, the gilts were housed in groups of ten. From day 83 until day 106 of gestation, they were housed individually in crates (2·0 × 0·6 m) in the gestation house. In the farrowing house, the gilts were housed individually in farrowing pens (2·2 × 2·4 m). The gestation house and farrowing room temperature was maintained at 20°C throughout the experiment. The experimental supplement (SWE) was top-dressed on the gestation and lactation diets each morning (09.00 hours) to ensure consumption. The dams received specific amounts of feed in the following quantities: 2·5 kg/d of gestation diet from day 83 until day 106 of gestation. They were fed 2 kg/d of lactation diet from day 107 of gestation until the day of farrowing, and then the feed supply was increased by 1·0 kg/d until day 3 post-farrowing and by 0·5 kg/d until day 6 post-farrowing. Afterwards, the sows were allowed semi-ad libitum consumption of the lactation diet, which was adjusted for each sow depending on daily intake. The sows were fed in three equal meals provided at 09.00, 13.00 and 17.00 hours. The sows had ad libitum access to drinking-water throughout the experimental period.
Farrowing and piglet management
All farrowings were supervised. At parturition, each piglet (meat-line boars × Large White × Landrace genetic lines) was individually weighed and tagged. Between 6 and 12 h after the birth of the last piglet, litter size was adjusted by cross-fostering piglets within dietary treatments to ensure that sows nursed a similar number of piglets (n 12 piglets/sow), and this was maintained throughout the suckling period. The individual piglet body weight (BW) was recorded at birth, on day 7 and at weaning, and ADG calculated from these data. Piglets received an intramuscular injection of Fe-dextran (Ferdex 100; Medion Farma Jaya) on day 7 after birth. No creep feed was offered to the piglets throughout the lactation period.
Post-weaning period – experimental design and animal management
At weaning, a total of forty male pigs (n 2 pigs/litter) with an average BW of 8·4 (sd 0·14) kg were selected. The selection was based on the average BW of the litter. The pigs were individually penned in a fully slated pen (1·7 m × 1·2 m). The pigs were fed ad libitum with a standard diet for this age of pig until day 13 PW (Table 1). The diet was formulated to contain 210 g/kg of crude protein, 15·0 MJ/kg of digestible energy and 14·5 g/kg of ileal digestible lysine. Water was available from nipple drinkers at all times. Feed was available up to weighing of the pigs, and then weighed back for the purpose of calculating feed intake. Representative feed sample was taken on day 0 (day of weaning) for chemical analysis. The ingredient composition and chemical analysis of the PW diet are presented in Table 1.
House temperature was maintained at 30°C during the first 7 d and then reduced to 28°C until day 13 PW. On days 9 and 10 PW, twenty pigs (ten pigs weaned from the basal-fed sows and ten pigs weaned from the SWE-supplemented sows) were housed in unsanitised rooms and orally challenged with ETEC K88 to induce PW diarrhoea and the other twenty pigs were housed in sanitised rooms and received only PBS (Oxoid), resulting in four groups: pigs weaned from the basal-fed sows and unchallenged (control pigs, n 10; BC); pigs weaned from the basal-fed sows and ETEC k88-challenged on days 9 and 10 PW (n 10; BE); pigs weaned from the SWE-supplemented sows and unchallenged (n 10; SC); pigs weaned from the SWE-supplemented sows and ETEC k88-challenged on days 9 and 10 PW (n 10; SE). Each treatment was housed in separated rooms, to avoid cross-contamination between the treatments. The unsanitised rooms were uncleaned following a previous batch of weaned pigs, while the sanitised rooms were cleaned and disinfected following the previous batch of weaned pigs. The pigs were weighed at weaning (day 0), day 9 (before the ETEC K88 challenge) and at the end of the experiment (day 13). The pigs were humanely killed on day 13 by lethal injection with pentobarbital sodium (Euthatal Solution, 200 mg/ml; Merial Animal Health Limited) at a rate of 0·71 ml/kg BW to the cranial vena cava, and the entire GI tract was immediately removed.
Enterotoxigenic Escherichia coli K88 challenge – bacterial culture, oral challenge, health status and sample collection
The ETEC K88 strain (Abbotstown, O149:K91:K88), obtained from Animal Health and Veterinary Laboratories Agency (AHVLA, Addlestone, Surrey, UK), was grown in Luria-Bertani broth. The pathogen was derived from clinical cases of PW colibacillosis and characterised as having the required virulence factors to induce PW colibacillosis. The expanded culture of ETEC K88, approximately 1 × 108 colony-forming units/ml, was further prepared for oral dosing as described by Zhang et al. ( Reference Zhang, Xu and Liu 17 ). The suspension was calculated on the basis of optical density established by serial dilution before bacterial cell count. Pigs were not tested for susceptibility to these adhesion factors, but were from a herd where persistent PW ETEC K88 shedding was recorded. At 08.00 hours on days 9 and 10 PW, the pigs (n 20) were orally given a dose of 10 ml PBS containing approximately 1 × 108 colony-forming units of ETEC K88 using a syringe attached to a polyethylene tube held in the back of the oral cavity. The unchallenged control pigs received 10 ml of sterilised PBS only. The bacterial solution and PBS was slowly dribbled into the pig's throat so that the swallowing reflex was triggered and passage of the inoculant into the lungs was minimised.
The pigs were checked for diarrhoea to evaluate their status before and after challenging with ETEC K88. Severity of diarrhoea was characterised by using the following faecal consistency score system described by Pierce et al. ( Reference Pierce, Callan and McCarthy 18 ): (1) hard firm faeces; (2) slightly soft faeces; (3) soft, partially formed faeces; (4) loose, semi-liquid faeces (mild diarrhoea); (5) watery, mucous-like faeces (severe diarrhoea). Faecal scores were taken before the ETEC challenge (day 9, 0 h) and every 12 h after the challenge until day 13 (72 h). Faecal consistency score was performed by two trained personnel with no prior knowledge of the sow's dietary treatment.
Fresh faecal samples were collected directly from the rectum of each pig at 0, 24 and 48 h after ETEC K88 challenge and stored at − 20°C for quantification of ETEC K88 toxin genes (heat-labile enterotoxin – LT, heat-stabile enterotoxin b – STb and enteroaggregative E. coli heat-stable enterotoxin 1 – EAST1). Diarrhoea caused by ETEC K88 is attributable to the action of one or more enterotoxins produced by the bacteria that have colonised the small intestine, such as LT, STb and EAST1 ( Reference Fairbrother, Nadeau and Gyles 4 , Reference Nataro, Deng and Cookson 19 ).
Chemical analysis
The feed samples (gestation, lactation and PW diets) were analysed for N, DM, ash, gross energy and neutral-detergent fibre). The feed samples were milled through a hammer mill provided with a 1 mm screen (Christy and Norris Hammer Mill; Christy Turner Limited). The nutrient contents (N, DM, ash, gross energy and neutral-detergent fibre) in the diets were determined as described by Heim et al. ( Reference Heim, Walsh and Sweeney 13 ). The total laminarin content of the SWE was determined using a Megazyme kit (Megazyme International Ireland Limited). Fucoidan levels were determined using the method described by Usov et al. ( Reference Usov, Smirnova and Klochkova 20 ).
Collection of tissue samples
Immediately after slaughter, the entire digestive tract was removed by blunt dissection, and sections of the duodenum (10 cm from the stomach), the jejunum (60 cm from the stomach) and the ileum (15 cm from the caecum) were excised and fixed in 10 % phosphate-buffered formalin for villus height (VH) and crypt depth (CD) measurements. Ileum and colon tissues (second loop of the proximal colon) were excised, emptied by dissecting them along the mesentery and rinsed using sterile PBS (Oxoid). Tissue sections of 1 cm2, which was stripped of the overlying smooth muscle, were cut from each tissue and stored in 15 ml RNAlater® solution (Applied Biosystems) overnight at 4°C. RNAlater® was then removed before storing the samples at − 80°C.
Microbiology – DNA extraction and quantitative PCR from faecal samples
Microbial genomic DNA was extracted from faecal samples using a QIAamp DNA stool kit (Qiagen) in accordance with the manufacturer's instructions. Quantity and quality of DNA was assessed using a Nanodrop ND1000 spectrophotometer (Thermo Scientific). Standard curves were generated as described by O'Shea et al. ( Reference O'Shea, Sweeney and Bahar 21 ). Briefly, genomic DNA from all faecal samples was pooled and amplified through routine PCR using ETEC K88 toxin primers (LT, STb and EAST1). The ETEC K88 toxin primers are presented in Table 2. All primers were designed using Primer Express™ software (Applied Biosystems) and synthesised by MWG Biotech. Serial dilutions of these amplicons served to generate standard curves using quantitative real-time PCR (qPCR – ABI 7500 Real-Time PCR System; Applied Biosystems Limited), allowing estimations of absolute quantification based on gene copy number (GCN)( Reference Lee, Kim and Shin 22 ). qPCR were performed in a final reaction volume of 20 μl containing 3 μl of template DNA, 1 μl of forward (100 pm) and 1 μl of reverse primers (100 pm), 10 μl SYBR Green PCR Master Mix (Applied Biosystems) and 5 μl nuclease-free water. The thermal cycling conditions involved an initial denaturation step at 95°C for 10 min followed by forty cycles of 95°C for 15 s and 65°C for 1 min. Dissociation analyses of the qPCR product were performed to confirm the specificity of the resulting qPCR products. All samples were prepared in duplicate. The mean cycle threshold (C t) values of duplicates of each sample were used for calculations.
Table 2 Porcine oligonucleotide primers used for real-time PCR

LT, heat-labile enterotoxin; F, forward; R, reverse; STb, heat-stabile enterotoxin b; EAST1, enteroaggregative Escherichia coli heat-stable enterotoxin 1; IFN-γ, interferon-γ; TGF-β1, transforming growth factor-β; FOXP3, forkhead box P3; PPIA, peptidylprolyl isomerase A; BM, β2-microglobulin.
* Zhang et al. ( Reference Zhang, Zhao and Ruesch 47 ).
† Metzler-Zebeli et al. ( Reference Metzler-Zebeli, Hooda and Pieper 48 ).
Duodenal, jejunal and ileal morphology
The preserved segments (duodenum, jejunum and ileum) were prepared using standard paraffin-embedding techniques. The samples were sectioned at 5 μm thickness and stained with haemotoxylin and eosin( Reference Liu, Piao and Thacker 23 ). Measurements of fifteen well-orientated and intact villi and crypts were taken for each segment. The VH and the CD were measured as described by Heim et al. ( Reference Heim, Walsh and Sweeney 13 ). The results are expressed as mean VH or CD in μm. The VH:CD ratio was calculated.
RNA extraction, complementary DNA synthesis and quantitative real-time PCR
RNA was extracted from approximately 50 mg of tissue samples using the GenElute Mammalian Total RNA Miniprep Kit (Sigma-Aldrich Corporation), according to the manufacturer's instructions. Total RNA was quantified using 1·5 μl of total RNA on a NanoDrop Spectrophotometer ND1000 (Thermo Fisher Scientific, Inc.), and samples with a 260:280 ratio ≥ 2·0 were considered suitable for complementary DNA (cDNA) synthesis. Total RNA integrity (i.e. quality and quantity) was assessed by analysing 1 μl of total RNA using the Agilent 2100 Bioanalyser version A.02.12 (Agilent Technologies, Inc.) using RNA Nano LabChips® (Caliper Technologies Corporation). cDNA synthesis was performed using 1 μg of total RNA and oligo(dT)20 primers in a final reaction volume of 20 μl using Superscript™ III First-Strand synthesis kit (Invitrogen Life Technologies) following the manufacturer's instructions. The final reaction volume of 20 μl was then adjusted to 250 μl using nuclease-free water. All primers for the selected cytokine genes IL-1, IL-2, IL-6, IL-8, IL-10, IL-12A (p35), IL-17, TNF-α, interferon-γ (IFN-γ), transforming growth factor-β1 (TGF-β1), forkhead box P3 (FOXP3) were designed using Primer Express™ (PE Applied Biosystems) and synthesised by MWG Biotech. Primer sequences are presented in Table 2. A panel of pro- and anti-inflammatory cytokines chosen was selected based on previous work by Walsh et al. ( Reference Walsh, Sweeney and O'Shea 12 ) and Leonard et al. ( Reference Leonard, Sweeney and Bahar 24 ). The efficiencies of all primer sets were established using a semi-log curve of quantity v. control, of two-fold serial dilutions of cDNA as reported previously by Smith et al. ( Reference Smith, O'Doherty and Reilly 25 ). The following two porcine reference genes were used as described previously by Ryan et al. ( Reference Ryan, Collins and O'Doherty 26 ): β2-microglobulin (BM) and peptidylprolyl isomerase A (PPIA). qPCR was then carried out on cDNA using the ABI PRISM 7500 Fast Sequence Detection System for ninety-six-well plates (Applied Biosystems). All samples were prepared in duplicate using the SYBR Green Fast PCR Master Mix (Applied Biosystems), cDNA as the template and specific primers for the genes selected. For each reaction, 5 μl cDNA, 1·2 μl forward and reverse primer mix (300 nm), 3·8 μl nuclease-free water and 10 μl Fast SYBR Green PCR Master Mix (PE Applied Biosystems) were added and made up to a final volume of 20 μl. The two-step PCR programme was as follows: 95°C for 10 min for one cycle, followed by 95°C for 15 s and 60°C for 1 min for forty cycles.
The mean C t values of duplicates of each sample were used for calculations. Normalised relative quantities were obtained using qbase PLUS software (Biogazelle).
Statistical analysis
All data were analysed using the general linear model procedure of Statistical Analysis System (SAS; SAS Institute, Inc.)( 27 ). All data were initially checked for normality using the univariate procedure in SAS( 27 ). For pig performance (BW, ADG, average daily feed intake (ADFI) and gain:feed ratio (G:F)), faecal score, ETEC K88 toxin gene expression and cytokine gene expression, the data were analysed by repeated-measures analysis using the PROC MIXED procedure of SAS( Reference Littell, Henry and Ammerman 28 ). The model included the effects of sow dietary treatment, ETEC K88 challenge and time, and the associated two-way and three-way interactions. Gene copy estimates of ETEC K88 toxins were log-transformed before statistical analysis. The statistical model used for cytokine gene expression included both GI sites (ileum v. colon), sow dietary treatment and ETEC K88 challenge and the associated two-way and three-way interactions. Small intestinal morphology data were analysed as a 2 × 2 factorial arrangement, with the pig as the experimental unit. The statistical model used included the effects of sow dietary treatment and ETEC K88 challenge and the associated interaction. Contrast statements were used to compare (1) basal-fed sows v. SWE-supplemented sows (lactation effect), (2) non-challenge v. ETEC K88 challenge PW effect and (3) the interaction between the lactation effect and the PW effect. The sow data for the lactation period were analysed as a complete randomised block design, with the sow as the experimental unit. The pdiff function of SAS was used to separate means.
All data presented in the tables are expressed as least-square means with their standard errors of the mean. The probability level that denotes significance is P< 0·05.
Results
Lactation period
Gilts offered the SWE extracts had a longer gestation period than basal-fed gilts (114·5 v. 113·5 d, sem= 0·27, P< 0·05). Litter size (14·4 (sd 0·72) piglets) and number of live-born piglets (12·9 (sd 0·62)) were not influenced by sow dietary treatments (P>0·10). Sow dietary treatment had no effect on piglet BW at birth (1·76 (sd 0·66) kg), on day 7 after birth (2·70 (sd 0·09) kg) and at weaning (8·33 (sd 0·22) kg), and had no effect on ADG from birth to day 7 of lactation (0·189 (sd 0·010) kg/d) and from birth to weaning (0·240 (sd 0·069) kg/d) (P>0 10). Piglets suckling SWE-supplemented sows had higher ADG compared with those suckling basal-fed sows (0·249 v. 0·230 kg/d; sem= 0·064; P< 0·05) from day 8 of lactation to weaning.
Post-weaning period
Growth performance
The effect of sow dietary treatment and ETEC K88 challenge on pig growth performance PW is presented in Table 3. No difference was recorded in pig BW at weaning (day 0) among the dietary treatments (P>0·10). There was a sow dietary treatment × ETEC K88 challenge (SWE × ETEC) interaction effect on pig BW (days 9 and 13), ADG (days 0–9 and 9–13) and G:F (days 0–9) (P< 0·05). The BE pigs had a decreased BW, ADG and G:F compared with the SE pigs (P< 0·05). However, there was no difference in BW, ADG and G:F between the BC and SC pigs (P>0·10). There was a SWE × ETEC × time interaction effect on pig ADFI (P< 0·05). The BE pigs had a decreased ADFI compared with BC pigs during 0–9 and 9–13 d (P< 0·05). However, there was no difference in ADFI between the SC and SE pigs during 9–13 d (P>0·10), and the SE pigs had decreased ADFI compared with the SC pigs during 0–9 d (P< 0·05).
Table 3 Effect of sow dietary treatment on weaned pig performance following an enterotoxigenic Escherichia coli (ETEC) K88 challenge* (Least-squares mean values with their standard errors)

BC, pigs weaned from the basal-fed sows and unchallenged; BE, pigs weaned from the basal-fed sows and ETEC K88-challenged on days 9 and 10 post-weaning (PW); SC, pigs weaned from the seaweed extracts (SWE)-supplemented sows and unchallenged; SE, pigs weaned from the SWE-supplemented sows and ETEC K88-challenged on days 9 and 10 PW; BW, body weight; ADG, average daily gain; ADFI, average daily feed intake; G:F, gain:feed ratio.
* ETEC K88 oral challenge: days 9 and 10 PW.
Faecal score
The effect of sow dietary treatment and ETEC K88 challenge on pig faecal score from 0 h (immediately before the ETEC K88 challenge) to 72 h post-challenge is presented in Fig. 1. There was a SWE × ETEC × time interaction effect on pig faecal score from 0 to 72 h post-challenge (P< 0·05). The BE pigs had higher faecal score at 0, 12, 36, 48, 60 and 72 h post-challenge compared with the SE pigs (P< 0·05). However, there was no difference in faecal score at 0, 12 and 36 h post-challenge between the BC and SC pigs (P>0·10); at 24 h, the BC pigs had lower faecal score compared with the SC pigs, and at 48, 60 and 72 h post-challenge, the BC pigs had higher faecal score compared with the SC pigs (P< 0·05).

Fig. 1 Differences in pig faecal score over time at 0 (immediately before the enterotoxigenic Escherichia coli (ETEC) K88 challenge), 12, 24, 36, 48, 60 and 72 h post-challenge. Values are means, with their standard errors represented by vertical bars. BC, pigs weaned from basal-fed sows and unchallenged ( − SWE − ETEC K88; ); BE, pigs weaned from basal-fed sows and ETEC K88-challenged on days 9 and 10 post-weaning (PW) ( − SWE +ETEC K88;
); SC, pigs weaned from SWE-supplemented sows and unchallenged (+SWE − ETEC K88;
); SE, pigs weaned from SWE-supplemented sows and ETEC K88-challenged on days 9 and 10 PW (+SWE +ETEC K88;
). There was an effect detected for the SWE × ETEC × time interaction (P< 0·001).
Gene expression of enterotoxigenic Escherichia coli K88 toxins in the faeces
The effect of sow dietary treatment and ETEC K88 challenge on EAST1, LT and STb log GCN/g faeces at 0, 24 and 48 h post-challenge is presented in Table 4. There was a time effect on LT, EAST1 and STb log GCN/g faeces (P< 0·05). At 24 h post-challenge, LT and STb log GCN/g faeces were decreased compared with the faecal collection time point at 0 and 48 h post-challenge (P< 0·05). In contrast, EAST1 log GCN/g faeces was increased at 24 h compared with the 0 and 48 h time points (P< 0·05). The ETEC K88 challenge increased EAST1 and STb log GCN/g faeces (P< 0·05). Pigs weaned from the SWE-supplemented sows had reduced LT log GCN/g faeces compared with those weaned from the basal-fed sows (P< 0·05).
Table 4 Effect of sow dietary treatment on enterotoxigenic Escherichia coli (ETEC) K88 toxin log gene copy number/g faeces following an ETEC K88 challenge* (Least-squares mean values with their standard errors, n 10 pigs/treatment group)

SWE, seaweed extracts; LT, heat-labile enterotoxin; STb, heat-stabile enterotoxin b; EAST1, enteroaggregative E. coli heat-stable enterotoxin 1.
* ETEC K88 oral challenge: days 9 and 10 post-weaning.
† There was no effect detected for the SWE × ETEC interaction (P>0·10) or the SWE × ETEC × time interaction (P>0·10).
Small-intestinal morphology
The effect of sow dietary treatment and ETEC K88 challenge on VH, CD and the VH:CD ratio in the duodenum, jejunum and ileum of pigs on day 13 PW is shown in Table 5. Pigs challenged with ETEC K88 tended to have a reduced VH:CD ratio in the duodenum (P= 0·064) and deeper CD in the jejunum (P< 0·05) compared with unchallenged pigs. Pigs weaned from the SWE-supplemented sows had longer VH and higher VH:CD ratio in the ileum compared with those weaned from the basal-fed sows (P< 0·05).
Table 5 Effect of sow dietary treatment and enterotoxigenic Escherichia coli (ETEC) K88 challenge* on small-intestinal morphology of pigs on day 13 post-weaning (Least-squares mean values with their standard errors, n 10 pigs/treatment group)
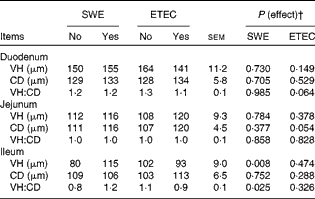
SWE, seaweed extracts; VH, villus height; CD, crypt depth.
* ETEC K88 oral challenge: days 9 and 10 post-weaning.
† There was no effect detected for the SWE × K88 interaction (P>0·10).
Cytokine gene expression
The effect of sow dietary treatment and ETEC K88 challenge on the expression of pro- and anti-inflammatory cytokines in the ileum and in the colon tissues is presented in Table 6. There was a SWE × ETEC interaction effect on the gene expression of the cytokine IL-6 (P< 0·05). The gene expression of IL-6 was down-regulated in the ileum and colon tissues of SC pigs compared with BC pigs (P< 0·05). However, there was no difference in the gene expression of IL-6 in the ileum and colon tissues between the SE and BE pigs (P>0·10). There was an ETEC K88 × GI region interaction effect on the gene expression of the cytokines IL-6, IL-10, IL-12A (p35) and TGF-β1 (P< 0·05). The gene expression of IL-10 and IL-12A (p35) was up-regulated in the ileum of ETEC K88-challenged pigs compared with their expression in the ileum of unchallenged pigs (P< 0·05). However, there was no difference in the gene expression of IL-10 and IL-12A (p35) in the colon between the ETEC K88-challenged and unchallenged pigs (P>0·10). The gene expression of IL-6 and TGF-β1 was down-regulated in the colon of ETEC K88-challenged pigs compared with their expression in the colon of unchallenged pigs (P< 0·05). However, there was no difference in the gene expression of IL-6 and TGF-β1 in the ileum between the ETEC K88-challenged and unchallenged pigs (P>0·10). There was a SWE × GI region interaction effect on the gene expression of the cytokines IL-8, TGF-β1 and TNF-α (P< 0·05). The gene expression of IL-8 was up-regulated in the ileum of pigs weaned from the SWE-supplemented sows compared with its expression in the ileum of pigs weaned from the basal-fed sows (P< 0·05). However, there was no difference in the gene expression of IL-8 in the colon between the pigs weaned from the SWE-supplemented sows and those weaned from the basal-fed sows (P>0·10). The gene expression of TGF-β1 and TNF-α was down-regulated in the colon of pigs weaned from the SWE-supplemented sows compared with their expression in the colon of pigs weaned from the basal-fed sows (P< 0·05). However, there was no difference in the gene expression of TGF-β1 and TNF-α in the ileum between the pigs weaned from the SWE-supplemented sows and those weaned from the basal-fed sows (P>0·10). The gene expression of IFN-γ tended to be up-regulated in the ileum and colon tissues of pigs weaned from the SWE-supplemented sows compared with those weaned from the basal-fed sows (P= 0·09). The gene expression of IL-10 was up-regulated in the ileum and colon tissues of pigs challenged with ETEC K88 compared with unchallenged pigs (P< 0·05). No effect was observed on the gene expression of the cytokines FOXP3, IL-1, IL-17 and IL-2 in the ileum and colon tissues (P>0·10).
Table 6 Effect of sow dietary treatment on the transcriptional response of pig genes related to immune response following an enterotoxigenic Escherichia coli (ETEC) K88 challenge on days 9 and 10 PW (Least-squares mean values with their standard errors, n 10 piglets per treatment group)

SWE, seaweed extracts; ETEC, enterotoxigenic Escherichia coli K88; GI, gastrointestinal; FOXP3, forkhead box P3; IFN-γ, interferon-γ; TGF-β1, transforming growth factor-β1.
* There was no effect detected for the SWE × ETEC × GI interaction (P>0·01).
† There was an effect detected for the SWE × ETEC interaction (P< 0·05).
‡ There was an effect detected for the ETEC × GI interaction (P< 0·05).
§ There was an effect detected for the SWE × GI interaction (P< 0·05).
Discussion
The objective of the present experiment was to evaluate the effects of maternal dietary supplementation with SWE from day 83 of gestation until weaning on weaned piglet growth performance, faecal score, faecal ETEC K88 toxin quantification, intestinal histology and gene expression profiles of pro- and anti-inflammatory cytokines following an ETEC K88 challenge on days 9 and 10 PW. It was hypothesised that maternal supplementation with SWE would improve the growth performance and indicators of GI health of weaned pigs after an ETEC K88 challenge PW. The positive response observed in pigs weaned from SWE-supplemented sows on growth performance, faecal score, intestinal morphology and immunological response PW compared with those weaned from basal-fed sows supports the hypothesis.
Lactation period
The SWE supplement (10·0 g/d) contained laminarin (1·0 g), fucoidan (0·8 g) and ash (8·2 g). It is speculated that this level of ash in the SWE supplement would have no impact on sow performance as it made up only a very small proportion of total ash intake. The present data indicate that SWE supplementation from day 83 of gestation increased the gestation length of the gilt. Their gestation length was prolonged by 1 d compared with the basal-fed gilts. In the swine species, the gestation length is an average of 114 d, with 85 % of farrowing concentrating between 114 and 116 d of gestation( Reference Meredith and Meredith 29 ). However, this increase in gestation length did not influence piglet BW at birth. There was also no effect of maternal dietary treatment on piglet BW on day 7 of age and at weaning. Chau et al. ( Reference Chau, Colllier and Welsh 30 ) observed no effect of sow dietary supplementation with yeast-derived β-glucans in the growth rate of piglets. Similarly Leonard et al. ( Reference Leonard, Sweeney and Bahar 24 ) also reported no effect of sow supplementation with a similar SWE from day 107 of gestation to weaning (26 d) on piglet growth during the lactation period.
Post-weaning
The SE pigs had higher BW, ADG, ADFI and G:F compared with the BE pigs. However, there was no difference in BW, ADG, ADFI and G:F between the BC and SC pigs. The SE pigs had a lower faecal score at 0, 12, 36, 48, 60 and 72 h post-challenge compared with the BE pigs. However, there was no difference in faecal score at 0, 12 and 36 h post-challenge between the BC and SC pigs. At 48, 60 and 72 h post-challenge, the SC pigs had a lower faecal score compared with the BC pigs. The lack of a negative effect of ETEC K88 challenge on the growth performance and faecal score of pigs weaned from the SWE-supplemented sows could be due to a number of reasons. First, it may be attributed to the immunomodulatory effects of SWE in enhancing cellular and humoral immune function, as well as suppressing the E. coli population( Reference Leonard, Sweeney and Bahar 24 ). Leonard et al. ( Reference Leonard, Sweeney and Bahar 24 ) demonstrated that sow supplementation with SWE reduced colonic E. coli numbers in piglets at weaning. A suppressed colonic E. coli population may ultimately alleviate the incidence and severity of PW diarrhoea( Reference Melin, Mattsson and Katouli 31 ). The present data demonstrated that maternal supplementation with SWE down-regulated the gene expression of a panel of pro-inflammatory cytokines (IL-6, TGF-β and TNF-α) in the ileum and colon tissues. It has been shown that less activation of the immune system during the weaning period could result in an improvement in growth performance( Reference Li, Li and Xing 32 ). Second, it may be attributable to an increase in E. coli-phagocytising effect (not analysed in the present experiment). According to Leonard et al. ( Reference Leonard, Sweeney and Bahar 33 ), piglets suckling SWE-supplemented sows had a greater percentage of E. coli phagocytising leucocytes and a reduced percentage of E. coli-phagocytising lymphocytes at weaning, indicating an enhancement of immune function against presenting pathogens. Third, it may be attributable to the positive changes in the intestinal architecture (increased VH and VH:CD ratio in the ileum). A reduction in VH and the VH:CD ratio has been associated with poor performance and increased incidences of scouring in pigs challenged with ETEC( Reference Owusu-Asiedu, Nyachoti and Marquardt 34 ). Villi are critical components of the digestive tract and their geometry provides an indicator of the absorptive capacity of the small intestine( Reference Pluske, Hampson and Williams 7 ). Turnover of the intestinal epithelium reflects a dynamic equilibrium between the production of enterocytes in the crypts and their subsequent desquamation from the villus( Reference Pluske, Hampson and Williams 7 ). The VH:CD ratio is a useful criterion for assessing intestinal health and function( Reference Pluske, Hampson and Williams 7 , Reference Montagne, Pluske and Hampson 35 ). The results obtained from the present study showed that pigs weaned from the SWE-supplemented sows had longer villi and a higher VH:CD ratio in the ileum. Furthermore, sow dietary supplementation with SWE clearly prevented the damage to intestinal morphology, otherwise caused by ETEC K88 infection alone, which is in accordance with the improved performance and lower incidence of diarrhoea in pigs weaned from those sows.
Diarrhoea caused by ETEC K88 is attributable to the action of one or more enterotoxins produced by the bacteria that have colonised the small intestine, such as EAST1, either as the only toxin gene or with the classic toxins associated with this pathotype, LT and STb, to impair the intestinal barrier function, which indirectly induces fluid losses( Reference Fairbrother, Nadeau and Gyles 4 , Reference Nataro and Kaper 36 , Reference Yang, Jiang and Zheng 37 ). In the present experiment, the ETEC K88 challenge increased the gene expression of EAST1 and STb. Interestingly, in the present experiment, pigs weaned from the SWE-supplemented sows had reduced LT log GCN/g faeces compared with those weaned from the basal-fed sows. According to Fairbrother et al. ( Reference Fairbrother, Nadeau and Gyles 4 ), ETEC K88 is a major cause of diarrhoea in pigs during days 3–10 PW. The pathogen colonises in the small intestine, releasing specific enterotoxins that induce fluid losses( Reference Fairbrother, Nadeau and Gyles 4 – Reference Lallès, Bosi and Smidt 6 ). An in vivo study on gnotobiotic piglets revealed that LT is the major virulence factor of K88 ETEC, because it enhances bacterial colonisation of the intestine( Reference Berberov, Zhou and Francis 38 ). It may also be postulated from the present study that the reduction of ETEC K88 toxin LT in pigs weaned from the SWE-supplemented sows may be associated with the reduced faecal score of pigs weaned from those sows. However, a potential effect of ETEC was already present before the ETEC challenge begun, as evidenced by the GCN of ETEC toxins. Despite the presence of high GCN of ETEC toxins before the challenge, faecal scores were low, except for the BE pigs. Therefore, caution should be exercised when using the presence of toxin genes in the faeces as an indicator of ill health and diarrhoea in weaned pigs.
Maternal supplementation with SWE from day 83 of gestation and throughout the lactation period enhanced the immune response of weaned pigs reflected by a down-regulation of the gene expression of the pro-inflammatory cytokines IL-6, TGF-β and TNF-α. If maternal supplementation with SWE suppresses the secretion of pro-inflammatory cytokines, then less activation of the immune system during the weaning period would be achieved, which could result in an improvement in growth performance( Reference Li, Li and Xing 32 ). The gene expression of IL-10 and IL-12A (p35) (pro-inflammatory cytokines) was up-regulated in the ileum of ETEC K88-challenged pigs compared with their expression in the ileum of unchallenged pigs. According to Girard et al. ( Reference Girard, Oswald and Taranu 39 ), the first step in the pathogenesis of ETEC is adhesion to the ileal epithelium with the subsequent production of enterotoxins inducing intestinal acute diarrhoea and inflammation. Then, E. coli can be probably engulfed by antigen-presenting cells, e.g. macrophages, and evokes different courses of inflammation( Reference Pavlova, Volf and Alexa 40 ). An interesting observation is that the gene expression of cytokines classically involved in ETEC challenge (i.e. IL-1, IL-8 and TNF-α) was low or null in the present study. Perhaps some early sign may have already been passed in the small intestine on day 4 post-challenge. During ETEC infection, it is imperative to generate an adequate inflammatory response against the pathogen, accompanied by efficient regulation, in order to achieve protection without damaging the host tissues( Reference Takanashi, Tomosada and Villena 41 ). In the present study, the gene expression of IL-8 was up-regulated in the ileum of pigs weaned from the SWE-supplemented sows compared with its expression in the ileum of pigs weaned from the basal-fed sows. The gene expression of IFN-γ tended to be up-regulated in the ileum and colon tissues of pigs weaned from the SWE-supplemented sows compared with those weaned from the basal-fed sows. The tissue collection was carried out on day 13 PW, the period during which PW diarrhoea due to ETEC K88 generally occurs in pigs( Reference Carstensen, Ersbøll and Jensen 5 , Reference Lallès, Bosi and Smidt 6 ). Leonard et al. ( Reference Leonard, Sweeney and Bahar 24 ) reported that maternal supplementation with SWE enhanced the expression of a pro-inflammatory cytokine (TNF-α) in the ileum after an ex vivo lipopolysaccharide challenge at weaning. Rice et al. ( Reference Rice, Adams and Ozment-Skelton 42 ) also reported an increase of a pro-inflammatory cytokine (IL-6), in the serum, after oral administration of β-glucan. According to Xiao et al. ( Reference Xiao, Trincado and Murtaugh 43 ), monocytes/macrophages are the major effectors of innate immunity, functioning as the first barrier of natural defence. β-Glucans can stimulate macrophages, neutrophils and natural killer cells, and could promote T-cell-specific responses by the induction of cytokines such as IFN-γ, IL-8 and IL-12 from macrophages, neutrophils and natural killer cells( Reference Xiao, Trincado and Murtaugh 43 – Reference Chaung, Huang and Yu 45 ). Rice et al. ( Reference Rice, Adams and Ozment-Skelton 42 ) demonstrated that laminarin delivered orally can be internalised by intestinal epithelial cells and gut-associated lymphoid cells in the murine model. However, the possibility of mammary uptake of laminarin or fucoidan or both and subsequent introduction to the GI tract of suckling piglets was not examined in the present experiment. Furthermore, in the present experiment, the up-regulation of pro-inflammatory cytokines (IL-8 and IFN-γ) by maternal supplementation with SWE did not affect growth performance.
Conclusion
The data reported in the present study indicate that maternal supplementation with SWE from day 83 of gestation to weaning prevented the negative effect on growth performance and diarrhoea induced by the ETEC K88 challenge. It does so by improving the function of intestinal health, offsetting or preventing impaired intestinal morphology, reducing faecal ETEC K88 toxin (LT) and down-regulating a panel of inflammatory cytokines. Collectively, these results indicated an important immunomodulatory role of maternal SWE supplementation on piglet immune function at weaning that may help alleviate the negative impact of a disease challenge at this period. These results provide new insights into the protective activity of maternal SWE supplementation, making it a good alternative for improving the health status of pigs with ETEC-associated diarrhoea.
Acknowledgements
The present study was supported financially by the Irish Research Council for Science, Engineering and Technology (IRCSET) and BioAtlantis Limited. The IRCSET and BioAtlantis Limited had no role in the design or analysis of the study or in the writing of this article.
The authors’ contributions are as follows: G. H. wrote the manuscript, collected the samples and carried out the laboratory analysis; J. V. O’. D. was the principal investigator responsible for the design of the experiment, supervision of the data collection and statistical analysis and correction of the manuscript; T. S. designed the study and corrected the manuscript; C. J. O’. S. and D. N. D. contributed to the data collection and laboratory collection. All authors approved the final version of the manuscript.
None of the authors had a financial or personal conflict of interest in relation to the present study.