Pigs are confronted with multiple stressors during the post-weaning period such as a change in diet, separation from the sow and exposure to unfamiliar pigs(Reference Spreeuwenberg, Verdonk and Gaskins1). This situation generally results in low voluntary feed intake and suboptimal growth rate, both of which are associated with the effects on intestinal mucosal integrity and with the occurrence of pathological disorders(Reference Pluske, Hampson and Williams2). Supplementation with in-feed antibiotics has been widely used to reduce pathogen infection in swine; however, the European Union ban of these in-feed antibiotics has prompted the search for alternatives.
Recently, seaweed extracts containing both laminarin (LAM) and fucoidan (FUC) have been explored as a novel source of bioactive compounds containing immunomodulatory and antimicrobial properties(Reference Reilly, O'Doherty and Pierce3–Reference Leonard, Sweeney and Bahar5). LAM and FUC are the main water-soluble polysaccharides of brown algae(Reference Michell, Lahaye and Bonnet6). LAM represents a group of low-molecular-weight polysaccharides composed of β-(1 → 3)-linked glucans with β-(1 → 6)-linked side chains of varying distribution and length(Reference Read, Currie and Bacic7, Reference Brown and Gordon8). LAM have shown promising antibacterial(Reference Lynch, Sweeney and Callan9) and immunomodulating activities(Reference Rice, Adams and Ozment-Skelton10). FUC is a sulphated polysaccharide, containing l-fucose as one of the major monosaccharides(Reference Berteau and Mulloy11). Research has indicated that FUC may function as immunomodulatory(Reference Cumashi, Ushakova and Preobrazhenskaya12) and antibacterial agents(Reference Shibata, Iimuro and Uchiya13). Recently, O'Doherty et al. (Reference O'Doherty, Dillon and Figat14) found that the inclusion of a seaweed extract rich in both LAM and FUC increases the daily gain and the gain:feed ratio of pigs post-weaning mainly through improved nutrient digestibility and decreasing the faecal Escherichia coli population. However, the mode of action of the individual components of the seaweed extract is still unknown in the weaned pig's diet.
LAM and FUC may offer a dietary means to alter gut morphology and/or modulate immunity, thereby reducing the risk of diarrhoea and promoting growth performance in the absence of in-feed antibiotics. However, application of any alternative feed additive requires a detailed knowledge of the physiological effects. Therefore, the objective of the present experiment was to evaluate the effects of the dietary supplementation of LAM and FUC, independently or in combination, on gut morphology, selected intestinal microbiota populations, volatile fatty acid (VFA) concentrations and inflammatory cytokine gene expression in the weaned pig. It is hypothesised that the inclusion of both LAM and FUC would improve the aspects of gastrointestinal health after weaning due to the biological properties of LAM and FUC, resulting in an altered microflora and immune response in the gastrointestinal tract of the weaned pig.
Experimental methods
All procedures described in the present experiment were conducted under experimental licence from the Irish Department of Health in accordance with the cruelty to Animals Act 1876 and the European Communities (Amendments of the Cruelty to Animals Act 1976) Regulations.
Experimental diets
The experiment was designed as a 2 × 2 factorial arrangement, comprising four dietary treatments. The dietary treatments were as follows: (1) basal diet; (2) basal diet+240 parts per million (ppm) FUC; (3) basal diet+300 ppm LAM; (4) basal diet+300 ppm LAM and 240 ppm FUC. The concentrations of LAM and FUC used in the present study were based on previous work by Lynch et al. (Reference Lynch, Sweeney and Callan9) and McDonnell et al. (Reference McDonnell, Figat and O’ Doherty15). LAM (990 g LAM/kg) and FUC (720 g FUC/kg, 180 g/kg crude protein and 100 g/kg ash) were derived from Laminaria spp. and sourced from BioAtlantis Limited (Kerry Technology Park). The diets were fed for 8 d ad libitum; after which time pigs were humanely killed. The diets were formulated to have similar digestible energy (14·5 MJ/kg) and standardised ileal digestible lysine (12·5 g/kg) contents. All amino acid requirements were met relative to lysine(16). The ingredient composition and chemical analysis of the dietary treatments are presented in Table 1.
Table 1 Composition and chemical analysis of the basal diet (g/kg, unless otherwise indicated)
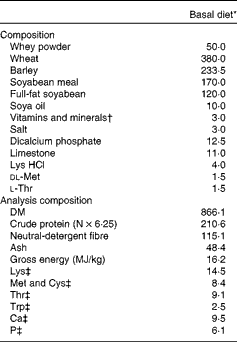
* Treatments: (1) basal diet; (2) basal diet+240 parts per million (ppm) fucoidan (FUC); (3) basal+300 ppm laminarin (LAM); (4) basal+300 ppm LAM and 240 ppm FUC.
† Vitamin and mineral inclusion (per kg diet): Cu, 25; Zn, 100; Se, 0·3; Mn, 25; I, 0·2; retinol, 0·3; cholecalciferol, 0·05; α-tocopherol, 40.
‡ Calculated for tabulated nutritional composition(Reference Sauvant, Perez and Tran51).
Animals and management
A total of twenty-eight pigs (progeny of large white × (large white × landrace sows)) were selected from a commercial pig unit at 24 d of age. The pigs had a weaning weight of 6·9 (sd 0·50) kg and were blocked on the basis of litter of origin and body weight (n 7). The pigs were individually housed in fully slated pens (1·7 m × 1·2 m). They were individually fed and had ad libitum access to feed and water. The house temperature was thermostatically controlled at 30°C throughout the experiment. The pigs were weighed at the beginning of the experiment (day 0) and at the end of the experiment (day 8).
Gut morphological analysis
The pigs were humanely killed on day 8 by lethal injection with Euthatal (pentobarbitone sodium BP; Merial Animal Limited) at a rate of 1 ml/1·4 kg body weight. On removal of the digestive tract, sections of the duodenum (10 cm from the stomach), the jejunum (60 cm from the stomach) and the ileum (15 cm from the caecum) were excised and fixed in 10 % phosphate-buffered formalin. The preserved segments were prepared using standard paraffin-embedding techniques. The samples were sectioned at a 5 μm thickness and stained with haematoxylin and eosin(Reference Pierce, Sweeney and Brophy17). Villous height and crypt depth were measured on the stained sections (4 × objective) using a light microscope fitted with an image analyser (Image-Pro Plus; Media Cybernetics). Measurements of fifteen well-oriented and intact villi and crypts were taken for each segment. Villous height was measured from the crypt–villous junction to the tip. Crypt depth was measured from the crypt–villous junction to the base. Results are expressed as mean villous height or crypt depth in μm.
Extraction and enumeration of microbial DNA from the gastrointestinal tract digesta
For microbial analysis, digesta samples (approximately 10 (sd 1) g) were aseptically recovered from the proximal colon of each pig immediately post-slaughter. The proximal colon is where we have previously observed much incidence of pathology and the region which has demonstrated the greatest response to dietary amendment with seaweed extracts. This reflects the dynamic microbial activity of the proximal colon before the recovery of moisture, as the digesta moves distally through the colon. Digesta samples were stored in sterile containers (Sarstedt), placed on ice and transported to the laboratory within 2 h. The Lactobacillus population was enumerated as an example of changes in the population structure of potentially beneficial bacteria(Reference Leser, Amenuvor and Jensen18), while the population of Enterobacteriaceae was enumerated as an example of potentially pathogenic bacteria(Reference Manzanilla, Perez and Martin19). The detection of the eae gene was taken as a marker for the presence of attaching and effacing E. coli (AEEC) strains that are characterised by their ability to cause lesions in the gut mucosa, leading to diarrhoeal disease as described previously by Krause et al. (Reference Krause, Zimmermann and Beutin20) and Ochoa et al. (Reference Ochoa, Barletta and Contreras21). Microbial genomic DNA was extracted from the digesta samples using a QIAamp DNA stool kit (Qiagen) in accordance with the manufacturer's instructions. The quantity and quality of DNA was assessed using a NanoDrop-ND1000 Spectrophotometer (Thermo Fisher Scientific, Inc.). Standard curves were prepared as described by O'Shea et al. (Reference O'Shea, Sweeney and Bahar22). Briefly, genomic DNA from all digesta samples was pooled and amplified through routine PCR using Lactobacillus, Enterobacteriaceae and eae-specific primers (Table 2). Serial dilutions of these amplicons served to generate standard curves using quantitative PCR (ABI 7500 Real-Time PCR System; Applied Biosystems Limited), permitting estimations of absolute quantification based on gene copies(Reference Lee, Kim and Shin23). Quantitative PCR was performed in a final reaction volume of 20 μl containing 1 μl of template DNA, 1 μl of forward and reverse primers (100 pm), 10 μl of SYBR Green PCR Master Mix (Applied Biosystems Limited) and 8 μl nuclease-free water. Thermal cycling conditions involved an initial denaturation step at 95°C for 10 min followed by forty cycles of denaturation at 95°C for 15 s and 65°C for 1 min. Dissociation analyses of the PCR product were performed to confirm the specificity of the resulting PCR products. Digesta samples were collected from the caecum and colon and were mixed with sodium benzoate and phenylmethylsulfonyl fluoride, in order to stop any bacterial activity and minimise the effects of post-thawing fermentation on resulting VFA concentrations. VFA analysis was performed using GLC according to the method described by Pierce et al. (Reference Pierce, Sweeney and Brophy17).
Table 2 Oligonucleotide primers used for quantitative PCR to profile the selected bacteria in the colon

T m, annealing temperature; AEEC, attaching and effacing Escherichia coli.
RNA extraction and complementary DNA synthesis
Tissue samples were collected from the mesenteric side of the colon, rinsed with ice-cold sterile PBS (Oxoid) and stripped of overlying smooth muscle. The samples were then cut into small pieces using a sterile scalpel blade and stored in 15 ml of RNAlater™ (Applied Biosystems) overnight followed by storage at − 20°C before RNA extraction. Total RNA was extracted from colonic tissue samples using a GenElute Mammalian Total RNA Miniprep Kit (Sigma-Aldrich Corporation) according to the manufacturer's instructions. To eliminate possible genomic DNA contamination, total RNA samples were subjected to DNase I (Sigma-Aldrich Corporation) treatment according to the manufacturer's instructions. RNA was further purified using phenol–chloroform(Reference Chomczynski and Sacchi24). Total RNA was quantified using a NanoDrop-ND1000 Spectrophotometer (Thermo Fisher Scientific, Inc.) and purity was assessed by determining the ratio of absorbance at 260 and 280 nm. All total RNA samples had 260:280 nm ratios above 1·8. In addition, RNA integrity was verified by the visualisation of 18S and 28S ribosomal RNA bands following gel electrophoresis stained with ethidium bromide (Egel; Invitrogen, Inc.). Complementary DNA synthesis was performed using 1 μg of total RNA and oligo (dT)20 primers in a final reaction volume of 20 μl using a Superscript™ III First-Strand Synthesis Kit (Invitrogen Life Technologies) following the manufacturer's instructions.
Quantitative PCR
All primers for the selected cytokines, IL-6, IL-8, TNF-α, IL-17A, IL-1β and IL-10, are presented in Table 3. The panels of pro- and anti-inflammatory cytokines chosen were selected based on previous work by Sweeney et al. (Reference Sweeney, Collins and Reilly25) and Leonard et al. (Reference Leonard, Sweeney and Bahar26). Overall, eight previously validated reference genes(Reference Ryan, Collins and O'Doherty27) were evaluated for stability in a subset of samples using geNorm(Reference Vandesompele, De Preter and Pattyn28), which included: β-actin (ACTB); β2-microglobin (B2M); glyceraldehyde-3-phosphate dehydrogenase (GAPDH); peptidylprolyl isomerase A (PPIA); hypoxanthine ribose transferase cyclophilin (HPRT); tyrosine 3-monooxygenase/tryptophan 5-monooxygenase activation protein ζ polypeptide (YWHAZ); hydroxymethyl-bilane synthase (HMBS); 18S ribosomal RNA (18S rRNA). HPRT and YWHAZ had the lowest stability M value of all the reference genes tested and were therefore chosen for subsequent normalisation. The stability ‘M’ value generated by the geNorm application was less than 1·5, which indicated their stability as endogenous controls (HPRT and YWHAZ) for these intestinal samples. Amplification was carried out in a reaction volume of 20 μl containing 10 μl of SYBR Green Fast PCR Master Mix (Applied Biosystems), forward and reverse primer mix (1 μl), 8 μl of diethylpyrocarbonate-treated water and 1 μl of template complementary DNA. Quantitative PCR was carried out using an ABI PRISM 7500 Fast Sequence Detection System for ninety-six well plates (Applied Biosystems). Thermal cycling conditions were as follows: an initial denaturation step at 95°C for 10 min, forty cycles of denaturation at 95°C for 15 s followed by 60°C for 1 min. Dissociation analysis of the PCR products was performed to confirm specificity. All samples were run in triplicate. Relative quantities for each target gene were generated by QBase software(Reference Hellemans, Mortier and De Paepe29) (Biogazelle).
Table 3 Porcine oligonucleotide primers used for quantitative PCR*
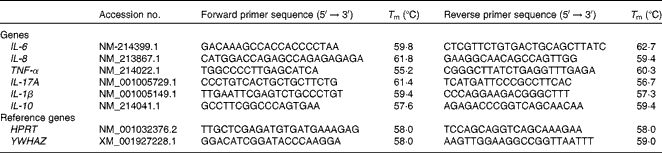
T m, melting temperature; HPRT, hypoxanthine ribose transferase cyclophilin; YWHAZ, tyrosine 3-monooxygenase/tryptophan 5-monooxygenase activation protein ζ polypeptide.
* Primers were designed using Primer Express™ software and were synthesised by MWG Biotech.
Laboratory analysis
The total LAM content in the diets was determined using a commercial assay kit (K-YBGL; Megazyme International Ireland Limited). The FUC level in the diets was determined according to the method described by Usov et al. (Reference Usov, Smirnova and Klochkova30). The feed samples were milled through a 1 mm screen (Christy and Norris Hammer Mill). The DM of the feed was determined after drying overnight at 103°C. Ash was determined after ignition of a known weight of concentrate in a muffle furnace (Nabertherm) at 500°C. The N content of the feed was determined using the LECO FP 528 instrument (Leco Instruments, UK Limited). The neutral-detergent fibre content was determined by the method of Van Soest et al. (Reference Van Soest, Robertson and Lewis31) using the Ankom 220 Fibre Analyzer (Ankom™ Technology). The gross energy of the feed was determined using a Parr 1201 oxygen bomb calorimeter (Parr).
Statistical analysis
Experimental data were analysed as a 2 × 2 factorial, randomised complete block design using the general linear model procedure of SAS(32). The statistical model used included the main effects of block, LAM and FUC supplementation, and the associated interaction between LAM and FUC. The individual pig served as the experimental unit. Food intake was included as a covariate in the model for villous height, crypt depth and the villous height:crypt depth ratio in the digestive tract, but was not significant for any of the variables. Gene copy estimates of selected bacteria were log-transformed before statistical analysis. Data were checked for normality using the Proc Univariate function of SAS. Means were separated using the Tukey–Kramer test. Probability values of less than 0·05 were used as the criterion for statistical significance. All results are presented as least-square means with their standard errors.
Results
Gut morphology
The effects of purified LAM and/or FUC on villous height, crypt depth and the villous height:crypt depth ratio in the gastrointestinal tract are shown in Table 4. No effect was observed in either the jejunum or the ileum (P>0·05). However, a significant interaction between LAM and FUC supplementation on villous height (P< 0·01) and the villous height:crypt depth ratio (P< 0·01) was observed in the duodenum. Pigs offered the LAM or FUC diet alone had an increased villous height and villous height:crypt depth ratio in the duodenum compared with pigs offered the basal diet. However, there was no effect of LAM in the diet on gut morphology when included with FUC.
Table 4 Effect of purified laminarin (LAM) and/or fucoidan (FUC) on villous height, crypt depth and the villous height:crypt depth ratio in the gastrointestinal tract of the weaned pig (Least-square mean values with their standard errors, n 7 pigs per treatment)
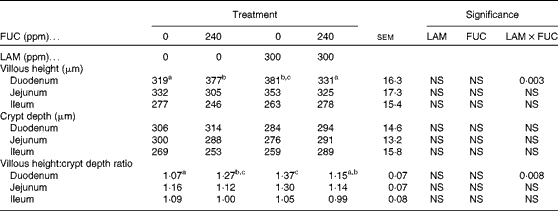
ppm, Parts per million.
a,b,cMean values with unlike superscript letters were significantly different (P< 0·05).
Microbiology
The effects of purified LAM and/or FUC on faecal scoring and selected microbial populations in the proximal colon of the pig are shown in Table 5. There was a significant interaction between LAM and FUC supplementation on the faecal score (P< 0·01). Pigs offered the LAM or FUC diet alone had a decreased faecal score compared with pigs offered the basal diet. However, there was no effect of the LAM and FUC combination diet on the faecal score. There was no effect of the dietary treatment on the Lactobacillus population (P>0·05) in the colon. There was a significant interaction between LAM and FUC supplementation on the Enterobacteriaceae population (P< 0·05) in the colon. Pigs offered the FUC diet alone had a reduced Enterobacteriaceae population compared with pigs offered the basal diet. However, there was no effect of FUC when combined with LAM on the Enterobacteriaceae population. There was a significant interaction between LAM and FUC supplementation on the abundance of AEEC strains in the colon (P< 0·05). Pigs offered the LAM diet alone had reduced abundance of AEEC strains compared with pigs offered the basal diet. However, there was no effect of LAM when combined with FUC on the abundance of AEEC strains.
Table 5 Effect of purified laminarin (LAM) and/or fucoidan (FUC) on faecal scoring and selected microbial populations in the proximal colon of the weaned pig (Least-square mean values with their standard errors, n 7 pigs per treatment)
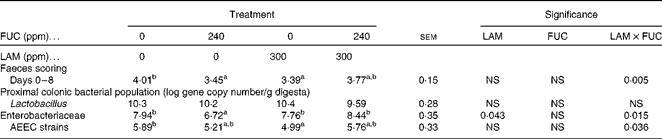
AEEC, attaching and effacing Escherichia coli.
a,bMean values with unlike superscript letters were significantly different (P< 0·05).
Cytokine gene expression analysis
The effects of purified LAM and/or FUC on cytokine gene expression in colonic tissues of the pig are shown in Table 6. There was no interaction (P>0·05) between LAM and FUC supplementation on IL-1β, IL-6 or IL-17A mRNA expression (P< 0·05) in the colon. Pigs offered the LAM-supplemented diets had a significantly lower IL-1β, IL-6 and IL-17A mRNA expression in the colon compared with pigs offered the diets without LAM supplementation. There was a significant interaction between LAM and FUC supplementation on IL-10 mRNA expression (P< 0·05) in the colon. Pigs offered the LAM diet alone had a reduced IL-10 mRNA expression compared with pigs offered the basal diet. However there was no effect of LAM when in combination with FUC on IL-10 mRNA expression. There was no effect of FUC alone on the expression of inflammatory cytokines (IL-6, IL-8, TNF-α, IL-17A, IL-1β and IL-10) in the colon (P>0·05).
Table 6 Effect of purified laminarin (LAM) and/or fucoidan (FUC) on the immune response in proximal colonic tissues (Least-square means of the relative abundance of each gene of interest with their standard errors, n 7 pigs per treatment)
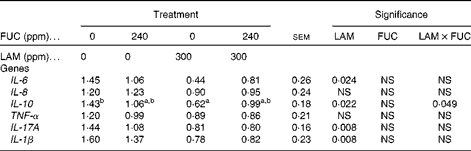
a,bMean values with unlike superscript letters were significantly different (P< 0·05).
Volatile fatty acids
The effects of purified LAM and/or FUC on the total VFA concentrations are shown in Table 7. There was no interaction (P>0·001) between LAM and FUC supplementation on total VFA and acetic acid concentrations in the caecum. Pigs offered the LAM-supplemented diets had increased concentrations of total VFA and acetic acid in the caecum compared with pigs offered the diets without LAM supplementation. There was an interaction between LAM and FUC supplementation on the concentrations of isobutyric acid (P< 0·01), isovaleric acid (P< 0·05), valeric acid (P< 0·05) and branched-chain fatty acids (P< 0·05) in the caecum. Pigs offered the FUC diet alone had decreased concentrations of isovaleric acid, valeric acid and branched-chain fatty acids compared with pigs offered the basal diet. However, there was no difference in the concentrations of these VFA when these pigs were offered the diets containing a combination of LAM and FUC compared with pigs offered the diets containing LAM alone. Pigs offered the FUC diet alone had increased concentrations of isobutyric acid compared with pigs offered the basal diet. However, pigs offered the diets containing a combination of LAM and FUC had similar concentrations of isobutyric acid compared with pigs offered the diets containing LAM alone.
Table 7 Effect of purified laminarin (LAM) and/or fucoidan (FUC) on the total volatile fatty acid (VFA) concentrations in the caecum and colon of the weaned pig (Least-square mean values with their standard errors, n 7 pigs per treatment)
a,b,cMean values with unlike superscript letters were significantly different (P< 0·05).
There was a significant interaction between LAM and FUC supplementation on the concentrations of valeric acid and branched-chain fatty acids (P< 0·05) in the colon. Pigs offered the FUC diet alone had decreased concentrations of branched-chain fatty acids compared with pigs offered the basal diet. However, there was no difference in the concentration of branched-chain fatty acids when these pigs were offered the diets containing a combination of LAM and FUC compared with pigs offered the diets containing LAM alone. Pigs offered the LAM diet alone had a decreased concentration of valeric acid compared with pigs offered the basal diet. However, there was no difference in the concentration of valeric acid when these pigs were offered the diets containing a combination of LAM and FUC compared with pigs offered the diets containing FUC alone.
Discussion
Recently, O'Doherty et al. (Reference O'Doherty, Dillon and Figat14) have shown the benefits of using a diet supplemented with seaweed extract containing a combination of LAM and FUC on post-weaning growth performance. The present study was designed to examine the effects of including purified LAM or FUC independently in weaned pig diets in order to understand the possible mode of action of each bioactive compound and their potential as alternatives to in-feed antibiotics. The results of the present study show that either purified LAM or FUC alone modified intestinal morphology and selected intestinal microbiota; however, these effects were not detected when the purified extracts were offered in combination. Furthermore, LAM inclusion alone down-regulated the gene expression of a selected panel of inflammatory cytokines in the colon.
Supplementation of either purified LAM or FUC exerted a positive influence on intestinal morphology in pigs 8 d post-weaning. This included an increase in villous height and the villous height:crypt depth ratio in the duodenum. This is an important observation as negative alterations normally occur in the intestinal morphology of pigs during the initial post-weaning period including reduced villous height and, to a lesser extent, reduced crypt depth(Reference Pluske, Thompson and Atwood33). Variations in villous height and the villous height:crypt ratio are a good indicator of variations in nutrient digestion and the absorption capacity of the small intestine(Reference Pluske, Thompson and Atwood33, Reference Montagne, Pluske and Hampson34).
In the present study, dietary consumption of FUC alone decreased the populations of Enterobacteriaceae in the colon. In agreement, Reilly et al. (Reference Reilly, O'Doherty and Pierce3) reported reductions in the Enterobacteriaceae population in the caecum and colon with the inclusion of seaweed extract containing a combination of LAM and FUC. FUC from different species of brown algae has been shown to inhibit the attachment of certain bacterial species in the porcine gastrointestinal tract as well as to prevent the binding of enterococci and streptococci species(Reference Shibata, Iimuro and Uchiya13) to the extracellular matrix proteins of animal cells. An inhibition of Enterobacteriaceae could prevent or decrease the severity of diarrhoea that appears after weaning(Reference Melin, Mattsson and Katouli35). This was reflected in the present study where pigs offered the FUC diet alone had a reduction in Enterobacteriaceae counts and displayed improved faecal consistency.
In the present study, the expression of a panel of pro- and anti-inflammatory cytokines (IL-6, IL-17A, IL-1β and IL-10) was down-regulated in the colon of pigs 8 d post-weaning following exposure to LAM. This is in agreement with a previous study in which the supplementation of β-glucans derived from Laminaria digitata, Laminaria hyperborea and Saccharomyces cerevisiae reduced the expression of a number of pro-inflammatory cytokine genes in the colon(Reference Sweeney, Collins and Reilly25). Anti-inflammatory cytokines such as IL-10 play an important role in maintaining the balance between pro- and anti-inflammatory mediators(Reference Hogaboam, Steinhauser and Schock36). In the present study, the anti-inflammatory cytokine IL-10 was down-regulated, which may reflect the decrease in the pro-inflammatory cytokines observed, as a spike in pro-inflammation would probably increase anti-inflammatory cytokines such as IL-10. Both in vitro and in vivo investigations have shown that overproduction of pro-inflammatory cytokines can reduce barrier integrity and alter epithelial permeability(Reference Mckay and Baird37). The anti-inflammatory effects of LAM may be attributed to a reduction of pathogen numbers, as was observed in the colon in the present study. The immunomodulatory effects of dietary LAM may be due to an indirect effect of LAM on the modulation of intestinal microbiota. If the supplementation of LAM suppresses the secretion of pro-inflammatory cytokines, then less activation of the immune system during the weaning period would be achieved, which could result in an improvement in growth performance(Reference Li, Li and Xing38). In a previous study, McDonnell et al. (Reference McDonnell, Figat and O’ Doherty15) reported the enhanced growth performance of pigs post-weaning with the dietary inclusion of dietary LAM.
Attaching and AEEC can be divided into two main pathogroups that are enteropathogenic E. coli and enterohaemorrhagic E. coli (Reference Nataro and Kaper39). Enteropathogenic E. coli and enterohaemorrhagic E. coli are implicated in post-weaning diarrhoea in pigs due to their ability to cause ‘attaching and effacing’ lesions(Reference Janke, Francis and Collins40–Reference Fairbrother, Nadeau and Gyles42). This supports the observations in the present study, where LAM supplementation alone reduced the abundance of AEEC strains in the colon while also improving the faecal score of weaned pigs. The lower abundance of AEEC strains could be attributed to a number of reasons. First, the reduction in the abundance of AEEC strains may be attributed to the agglutination properties of LAM, which is a novel source of β-(1 → 3)/(1 → 6) glucans. Yeast-derived β-glucans have the capacity to agglutinate certain bacterial species, thus inhibiting subsequent attachment and colonisation of epithelial mucosal surfaces(Reference Kogan and Kocher43). Second, it could be due to a host immune response following the consumption of LAM. Innate immune signalling has recently been shown to alter the composition of the microbiota, which consequently influences the development of disease(Reference Wen, Ley and Volchkov44, Reference Kinnebrew and Pamer45). Recent studies have suggested that certain pathogens exploit this relationship, and as a mechanism of pathogenesis, these pathogens promote intestinal inflammation to create a more favourable environment for growth(Reference Kinnebrew and Pamer45). In the present study, decreased inflammatory cytokine gene expression of the animals receiving the LAM diet alone may have promoted a more stable ecosystem that may have prevented the proliferation of some particular bacterial groups. Previous studies have suggested that inflammation induced in response to Salmonella enterica serovar Typhimurium alters luminal conditions and shifts growth competition in favour of the pathogen(Reference Stecher, Robbiani and Walker46). This may support the view that strains of E. coli have an advantage under conditions of inflammation, thus the inclusion of LAM alone decreasing the inflammatory response in the present study may partly account for the lower abundance of AEEC strains observed.
Previous research has focused on the dietary provision of seaweed extract containing both LAM and FUC, and found beneficial effects on post-weaning growth performance and selected faecal microbiota(Reference Gahan, Lynch and Callan4, Reference O'Doherty, Dillon and Figat14). It was observed from the results of the present experiment that either LAM or FUC alone could modulate intestinal morphology and selected intestinal microbiota; however, these effects were absent when offered in combination. The difference observed between studies may be due to a number of reasons. First, the interaction between LAM and FUC on branched-chain fatty acids in the caecum and proximal colon may help explain the findings with the combination treatment in the present study. The increase in Enterobacteriaceae numbers and branched-chain fatty acids would indicate the promotion of protein fermentation when the two algal polysaccharides are offered in combination compared with the FUC diet alone. O'Shea et al. (Reference O'Shea, Sweeney and Lynch47) showed an association between an increase in Enterobacteriaceae numbers and protein fermentation in the distal gastrointestinal tract. Branched-chain fatty acids mainly originate from protein fermentation(Reference Mortensen and Clausen48, Reference Macfarlane and Macfarlane49). Such fermentation can lead to the formation of toxic metabolites such as NH3 and amines, which have been implicated in the clinical expression of diarrhoea(Reference Macfarlane, Gibson and Beatty50). Similar results have been reported previously where grower–finisher pigs offered a combination of LAM and FUC increased Enterobacterium spp. and protein fermentation in the colon compared with pigs offered FUC alone(Reference Lynch, Sweeney and Callan9). Other reasons for the differences in responses observed between studies may be due to the variation in the purity of LAM and FUC between studies. In the present study, LAM and FUC were prepared as individual purified bioactive compounds, where individual purified components were extracted from the cell wall. Previous studies have utilised a more crude single extract of seaweed, where intact combinations of LAM and FUC are still in an intact complex and are probably less available. Third, another possible reason for the inconsistent results observed between studies may be due to the variations in experimental design and diet composition. The experimental period in previous studies(Reference Gahan, Lynch and Callan4, Reference O'Doherty, Dillon and Figat14) using seaweed extracts was longer (21–28 d) compared with the 8 d experimental period used in the present study. The interaction between LAM and FUC observed in the present study suggests distinct modes of action and the non-additive effects of these bioactive compounds.
The results of the present study show that supplementation with either purified LAM or FUC alone modified intestinal morphology and selected intestinal microbiota; however, these effects were not observed when offered in combination. Furthermore, the dietary inclusion of LAM alone had the added benefit of reducing inflammatory cytokine gene expression in the colon. The data suggest that LAM may have a different mode of action from FUC. Collectively, these results provide support that the inclusion of LAM alone was more beneficial than the FUC diet only or the combination treatment for weaned pigs. Future further studies will explore the effects of these bioactive compounds on growth performance and their potential to prevent the negative impact of a pathogen challenge.
Acknowledgements
Funding for the present study was provided by the Innovation Partnership Programme (Enterprise Ireland) and BioAtlantis Limited. A. M. W. wrote the manuscript, collected the samples and carried out the laboratory analysis. J. V. O’. D. designed the study, analysed the data and participated in the writing and correcting of the manuscript. T. S. designed the study and participated in the writing and correcting of the manuscript. C. J. O’. S. contributed towards the sample collection and laboratory analysis. D. N. D. contributed towards the sample collection. None of the authors had any conflict of interest.