l-Arginine (Arg) is an essential amino acid for chickens and is a conditionally essential amino acid for mammals under surgery, sepsis and many other pathological conditions( Reference Popovic, Zeh and Ochoa 1 – Reference Luiking, Poeze and Ramsay 3 ). It is used for the synthesis of NO and polyamines, which are involved in the modulation of inflammation and wound healing( Reference Bronte and Zanovello 2 , Reference Calkins, Bensard and Heimbach 4 , Reference Shi, Efron and Most 5 ). Although Arg has been used in chickens to enhance the immune function during immunological stress( Reference Tayade, Jaiswal and Mishra 6 ), there is still no consensus on the benefits of supplementing Arg beyond the NRC( 7 )-recommended concentration( Reference Jahanian 8 ). In normal animal-rearing conditions, the animal is inevitably confronted with various microbial challenges that often will not result in clinical disease. However, these microbial challenges lead to a series of immune responses, including mild inflammatory responses, which changes the partitioning of nutrients away from body protein accretion to support the immune responses, thereby reducing the growth potential of the animal( Reference Roura, Homedes and Klasing 9 ). Studies in piglets and chickens have demonstrated that dietary Arg supplementation could enhance the immune functions of animals under immunological stress( Reference Munir, Muneer and Masaoud 10 , Reference Han, Liu and Fan 11 ). Furthermore, a study in mice has shown that Arg administration decreases the expression of pro-inflammatory cytokines in a dextran sulphate sodium-induced inflammatory bowel disease model( Reference Coburn, Gong and Singh 12 ). However, few studies have been conducted to determine the effect of dietary Arg supplementation on the inflammatory response in chickens.
Lipopolysaccharide (LPS), or endotoxin, is a Gram-negative bacteria cell-wall component that has been extensively used in chickens to induce inflammatory response( Reference Zhang, Nii and Isobe 13 – Reference Munyaka, Tactacan and Jing 15 ). Toll-like receptor 4 (TLR4) is a germ line-encoded receptor that can be stimulated by structural motifs characteristically expressed by bacteria, such as LPS, which triggers the release of pro-inflammatory cytokines as well as the functional maturation of antigen-presenting cells of the innate immune system( Reference Lu, Yeh and Ohashi 16 ). In mammals, the sensing of LPS involves the LPS-binding protein (LBP), which mediates the transfer of LPS to CD14 and/or CD11/18 cells, which deliver the LPS to myeloid differentiation protein-2, which finally transfers the LPS to a TLR4 molecule( Reference Beutler 17 ). No clear orthologues to human LBP have been identified in the chicken genome; however, chickens still sense LPS via the TLR4 pathway and further trigger NF-κB-mediated inflammatory response( Reference Keestra and Putten 14 ).
TLR4 is a transmembrane signal transporter that activates intracellular NF-κB and mitogen-activated protein kinases, resulting in the production of pro-inflammatory cytokines such as IL-1β and IL-6( Reference Keestra and Putten 14 , Reference Zhang, Zhong and Zhou 18 , Reference Baker, Hayden and Ghosh 19 ). Calkins et al. ( Reference Calkins, Bensard and Heimbach 4 ) reported that oral Arg administration attenuates the LPS-induced overexpression of lung chemokines in mice by the inhibition of NF-κB DNA binding and that Arg supplementation in cell-culture media reduces the expression of TLR4 mRNA in swine intestinal cells( Reference Tan, Yin and Kong 20 ). Thus, the hypothesis of the present study was that dietary Arg supplementation could attenuate LPS-induced inflammation in broiler chickens through the suppression of the LPS/TLR4 pathway. The present study was designed to test this hypothesis using a LPS-induced inflammatory model and investigate the potential modulatory effect of dietary Arg supplementation on inflammatory response in broiler chickens.
Materials and methods
Birds and experimental design
In Expt 1, the effect of dietary Arg supplementation on the inflammatory response of chickens following LPS injection was determined. A total of 288 male Ross broilers (708; Aviagen, Inc.) were obtained from a local commercial hatchery. The chickens were weighed and allocated to groups so that their initial weights were similar across all the groups. This experiment was designed with six treatments, each involving eight replicate cages (six birds per cage) with a 2 × 3 factorial arrangement including two different treatments (an unchallenged positive control (control treatment) and a LPS-challenged negative control (LPS treatment)) and three concentrations of Arg (1·05, 1·42 or 1·90 %). The experimental design has been described in a previous study, in which chickens fed a diet containing 1·00 % of Arg had lower immunity( Reference Jahanian 8 ); Arg concentration of 1·40 % has been used in our previous study (J Tan, Y Guo, TJ Applegate and E Du, unpublished results) and it led to a good immune function in the chickens, and 1·90 % served as the highest concentration value of Arg used in the supplementation. In this experiment, all diets (except Arg) were formulated to meet or exceed the NRC( 7 ) requirements and Ross 708 Nutrient Specifications for broilers. An initial basal diet was mixed to contain a formulated Arg of 1·05 %. The final diets were obtained by mixing 98·4 % of the basal ration with a premix containing different concentrations of Arg and Solka-Floc® (purified cellulose; Table 1). At 14, 16, 18 and 20 d of age, the broilers were injected intra-abdominally with Escherichia coli LPS (serotype 0111:B4, LPS treatment; Sigma-Aldrich, Inc.) at a dose of 1 mg/kg of body weight (BW) or the same amount of 0·9 % (w/v) sterile saline (control treatment). At 21 d of age, one bird was randomly selected from each replicate and was killed by CO2 overdosing for sampling. Spleens were collected for splenocyte isolation and mRNA extraction and caecal tonsils were collected for RNA extraction. Tissue samples collected for RNA extraction were held overnight at 4°C in RNAlater (Ambion) and stored at − 80°C until RNA isolation.
Table 1 Composition of the experimental diets used in Expt 1 (g/kg dry diet)
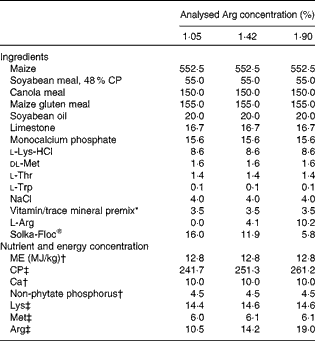
CP, crude protein; ME, metabolisable energy.
* Supplied the following per kg complete diet: Cu, 8 mg; Zn, 75 mg; Fe, 80 mg; Mn, 100 mg; Se, 0·15 mg; I, 0·35 mg; trans-retinyl acetate, 24 mg; cholecalciferol, 6 mg; dl-α-tocopheryl acetate, 7·2 mg; menadione, 1·3 mg; thiamin, 2 mg; riboflavin, 6 mg; cyanocobalamin, 0·025 mg; biotin, 0·0325 mg; folic acid, 1·25 mg; pantothenic acid, 12 mg; niacin, 50 mg.
† Calculated value.
‡ Analysed concentrations.
In Expt 2, the effect of graded supplementation of Arg (0·99, 1·39, 1·76, 2·13 or 2·53 %) on the percentage of circulating B cells (percentage of circulating lymphocytes) was investigated using a completely randomised design. A total of 250 female Ross broilers (308; Aviagen, Inc.) were used. The chickens were weighed and allocated to five treatments (ten replicates per treatment; five birds per replicate) so that the initial weight of each group was similar. The chickens were fed one of the five experimental diets for 21 d ad libitum. In this experiment, all diets (except Arg) were formulated to meet or exceed the NRC( 7 ) requirements for broilers. An initial basal diet was mixed to contain a formulated Arg of 0·99 %. The final diets were obtained by mixing 97·96 % of the basal ration with a premix (2·04 %) containing different concentrations of Arg and l-alanine. Ingredient formulation, nutrients and analysed dietary Arg concentration in Expt 2 are given in Table 2. At 21 d of age, a blood sample from one randomly selected bird per replicate was collected from the brachial vein with a heparinised syringe for peripheral blood mononuclear cell (PBMC) isolation.
Table 2 Composition of the experimental diets used in Expt 2 (g/kg dry diet)
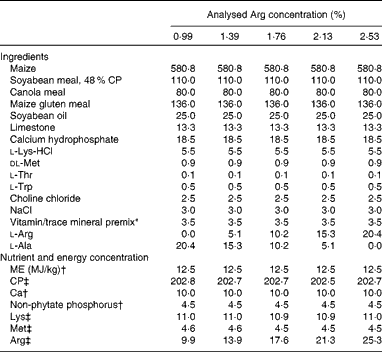
CP, crude protein; ME, metabolisable energy.
* Supplied the following per kg complete diet: Cu, 8 mg; Zn, 75 mg; Fe, 80 mg; Mn, 100 mg; Se, 0·15 mg; I, 0·35 mg; trans-retinyl acetate, 24 mg; cholecalciferol, 6 mg; dl-α-tocopheryl acetate, 7·2 mg; menadione, 1·3 mg; thiamin, 2 mg; riboflavin, 6 mg; cyanocobalamin, 0·025 mg; biotin, 0·0325 mg; folic acid, 1·25 mg; pantothenic acid, 12 mg; niacin, 50 mg.
† Calculated value.
‡ Analysed concentrations.
Animal use and care procedures followed in Expt 1 were approved by the Purdue Animal Care and Use Committee and those followed in Expt 2 were approved by the China Agricultural University Animal Care and Use Committee. Expt 1 and Expt 2 were carried out following the same management procedure. All the chickens were housed in stainless-steel brooders equipped with nipple-type waterers and thermostatically controlled heaters. The temperature was maintained at 37 ± 1°C during the first week and was reduced by 3°C each consecutive week until reaching 24°C. The lighting schedule was 22 h light–2 h dark throughout the experiments. Drinking-water and feed were provided ad libitum. Diet analyses for amino acid concentration were conducted using HPLC (University of Missouri Agriculture Experiment Station Chemistry Laboratory; AOAC International, 2000; method 982.30.E (a, b, c))( 21 ).
Splenocyte and peripheral blood mononuclear cell isolation
Splenocyte isolation was carried out as described previously( Reference Park, Cha and Kang 22 ) with the following modifications. The spleens were collected immediately after the chicks were killed and placed in sterile Roswell Park Memorial Institute (RPMI) medium in 10 ml tubes kept on ice. After removal of the adherent fat and tissues, a cell suspension was prepared by grinding the spleens through a 60 mesh screen; the cells were centrifuged at 80 g for 10 min at 4°C and the supernatant was collected into a new 15 ml tube and the pellet discarded. The supernatant was centrifuged at 200 g for 10 min at 4°C. The supernatant was discarded and the pellets were resuspended in 2·5 ml RPMI, after which splenocytes were washed two times. Splenocyte counts and viability were evaluated using Trypan Blue exclusion.
PBMC were isolated from peripheral blood samples using Ficoll density centrifugation according to the method of Long et al. ( Reference Long, Guo and Wang 23 ). Briefly, blood samples were carefully layered on the top of the Ficoll layer (Histopaque 1077; Sigma Chemical Company) in a 10 ml centrifuge tube (1:1). The tube was centrifuged at 200 g for 30 min (25°C). After centrifugation, the PBMC at the plasma–ficoll interface were collected. Cold RPMI-1640 medium (containing 5·0 % inactivated fetal bovine serum, 100 U (0·0599 mg) penicillin/ml, 100 μg streptomycin/ml and 10 mm-HEPES) was added to the tube containing the PBMC. Then, the cells were washed three times with cold RPMI-1640 medium by centrifugation at 100 g for 10 min (4°C). PBMC counts and viability were evaluated using Trypan Blue exclusion.
Determination of the phagocytic activity of splenocytes
The phagocytic activity of splenocytes was performed using pHrodo™ E. coli BioParticles® Phagocytosis Kits (Invitrogen Canada, Inc.). Briefly, 100 μl of splenocytes were incubated for 15 min at 37°C with 20 μl of pHrodo™ BioParticles® conjugate (Molecular Probes). Control samples were incubated for 15 min on ice. Then, the samples were washed two times according to the kit instructions, and the cell pellets were resuspended in 0·5 ml of wash buffer for flow cytometric analysis. The samples were analysed using an Accuri® C6 flow cytometer (Accuri Cytometers). Data were collected from 10 000 events. The threshold was adjusted to eliminate background fluorescence after the application of a negative control sample. The linear forward-scatter characteristic (FSC) and side-scatter characteristic (SSC) voltages were set to locate the heterophil and macrophage population following the manufacturer's instructions (Invitrogen Canada, Inc.) and as described previously( Reference Watson 24 ). Phagocytic activity is expressed as a percentage of cells that were phagocytic, and phagocytic intensity is expressed as mean fluorescence intensity.
Flow cytometric analysis of immune cell phenotypes
Splenocytes were stained with chicken CD3 (fluorescein isothiocyanate), chicken Bu-1 (phycoerythrin; PE), human CD14 (PE) and human CD11 (Alexa Fluor® 488) for 30 min in a water-bath (37°C). The PBMC were stained with chicken Bu-1 (PE) for 30 min on ice. The control samples were processed without antibody staining. All the antibodies used in the present study were purchased from Southern Biotech. The CD14 antibodies have been used to label chicken lymphocytes in a previous study( Reference Dil and Qureshi 25 ). The cells were then washed two times with 1 × Hanks balanced salt solution (HBSS), fixed with paraformaldehyde solution (3 % in HBSS) and stored at 4°C until further analysis. The samples were analysed using an Accuri®C6 flow cytometer (Accuri Cytometers). Lymphocytes were gated by FSC and SSC and 10 000 gated events were analysed. Briefly, subcellular dead cell debris can be distinguished from single cells by size as they have lower FSC and higher SSC than living cells. Compared with monocytes, lymphocytes have lower FSC and SSC. The threshold was adjusted to eliminate background fluorescence after the application of a negative control sample( Reference Watson 24 ). Cell phenotype data are expressed as a percentage of gated lymphocytes. The percentage of total splenic lymphocytes was based on total isolated splenocytes. The percentage of total peripheral blood lymphocytes was based on total isolated PBMC.
Total RNA extraction and RT
Total RNA of spleen and caecal tonsils was extracted using TRIzol Reagent (Invitrogen Canada, Inc.) following the manufacturer's protocol. The concentration, quality and integrity of total RNA were evaluated using a spectrophotometer as described previously( Reference Simms, Cizdziel and Chomczynski 26 ). The total RNA was incubated with DNase I (Sigma Chemical Company) at room temperature for 15 min, and the DNase I was subsequently inactivated by heating the reaction mixture at 70°C for 10 min. An RNA amount of 2 μg was used for RT using Moloney murine leukaemia virus RT (Promega) with Oligo-dt (Qiagen, Inc.) according to the manufacturer's protocol, and the complementary DNA was stored at − 20°C.
Real-time quantitative PCR
Real-time quantitative PCR for determining the expression of inflammation-related genes in the spleen and caecal tonsils was carried out using iQ SYBR Green Supermix (Bio-Rad) in a Bio-Rad iQ5 detection system (Bio-Rad Laboratories). The expression of glyceraldehyde-3-phosphate dehydrogenase was considered as an internal control to normalise the amount of starting RNA used for real-time quantitative PCR for each sample. A reaction mixture of 20 μl contained 10 μl of iQ SYBR Green Supermix (Bio-Rad), 0·3 μm of each forward primer and reverse primer (Table 3), and 6 μl of complementary DNA. Each analysis was carried out in triplicate. The following protocol was used for all the genes: 95°C for 5 min; forty cycles of 95°C for 10 s and 55°C for 20 s; 72°C for 20 s. The standard curve was determined using pooled samples, and the real-time quantitative PCR efficiency for each gene was determined by amplifying a dilution series of complementary DNA (10( − 1/slope)), and the efficiency values were consistent between the target genes and housekeeping gene. The specificity of the amplified products was evaluated with the melting curve analysis. The abundance of glyceraldehyde-3-phosphate dehydrogenase mRNA as the housekeeping gene was not influenced by the dietary Arg concentration and LPS injection in both the spleen and caecal tonsils (data not shown). The average gene expression relative to the expression of glyceraldehyde-3-phosphate dehydrogenase for each sample was calculated using the
$$2^{ - \Delta \Delta C _{t}} $$
method(
Reference Livak and Schmittgen
27
), and the calibrator for each gene was the average ΔC
t value of the pooled sample.
Table 3 Primers used in real-time quantitative PCR*
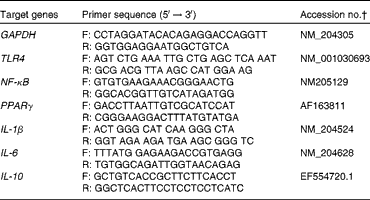
GAPDH, glyceraldehyde-3-phosphate dehydrogenase; F, forward; R, reverse; TLR, Toll-like receptor.
* Primers designed using the Primer Express software (Applied Biosystems).
† National Center for Biotechnology Information accession numbers.
Statistical analysis
The results are reported as means with their standard errors, and all the data were analysed using the GLM procedure of SAS software (SAS Institute, Inc.). Data were subjected to two-way ANOVA in a 2 × 3 factorial arrangement with immunological challenge and dietary Arg concentration as the main effects and their interactions. The analysis of interactions was based on a hypothesis that a non-linear response to Arg concentration could potentially interact with LPS; therefore, a polynomial contrast for the dose responsiveness of dietary Arg concentrations was conducted. When interactions were significant, polynomial contrasts were used to determine the linear and quadratic responses of simple means to dietary Arg concentrations within the LPS-injected and saline-injected groups, respectively. When interactions were not significant, the polynomial contrasts were conducted over main effect means for Arg concentrations (averaged over LPS treatments). At 14 d of age, the BW were different between the treatment groups; therefore, 14 d BW was used as a covariate in the model to analyse the growth performance from 14 to 21 d. Polynomial contrasts were used to determine significant linear, quadratic and cubic responses of circulating B cells (peripheral blood B cells) to dietary Arg concentrations (analysed dietary Arg concentrations). A P≤ 0·05 was considered to be statistically significant, and 0·05 < P <0·10 was considered to be a trend towards significance.
Results
Expt 1
With the exception of the feed conversion ratio (FCR) and IL-1β mRNA expression in the caecal tonsils, no significant Arg × LPS interactions were observed for any of the other measured characteristics.
Growth performance
The growth performance of chickens between 14 and 21 d of age is summarised in Table 4. Dietary Arg supplementation significantly increased (P< 0·05) the BW of chickens at 14 d of age. Compared with the saline injection, LPS injection significantly decreased (P< 0·05) the BW gain by 9·36 % and feed intake (FI) by 14·87 % in the LPS-challenged chickens, while dietary Arg supplementation significantly increased (P< 0·05) the BW gain and FI of the chickens. An interaction between LPS challenge and dietary Arg concentration was significant (P< 0·05) for the FCR, wherein chickens in the control group (1·05 % Arg) had the highest FCR and those in the challenged group (1·90 % Arg) had the lowest FCR compared with all the other groups. The FCR was linearly and quadratically (P< 0·05) reduced by increasing dietary Arg concentrations in the saline-injected groups. Increasing dietary Arg concentrations had a more pronounced effect in the non-challenged chickens than in the LPS-challenged chickens.
Table 4 Growth performance of broilers fed diets containing 1·05, 1·42 or 1·90 % arginine (Arg) with or without lipopolysaccharide (LPS) challenge from 14 to 21 d of age (Expt 1)* (Mean values with their standard errors)

L, linear; Q, quadratic; BW, body weight; BWG, body-weight gain; FI, feed intake; FCR, feed conversion ratio.
a,b Mean values within a row with unlike superscript letters have a L and Q dose response to Arg concentrations (P< 0.05).
* LPS injection: chickens were injected with LPS (1 mg/kg of BW) four times at 48 h intervals from 14 to 20 d; saline injection (control): chickens were injected with the same amount of sterile saline (0·9 %).
† L and Q contrasts on the main effect means for Arg concentrations (averaged over LPS treatments).
‡ Analysed by ANOVA.
§ Analysed by covariance analysis using 14 d BW as the covariant.
Determination of the phagocytic activity of splenocytes
LPS injection significantly suppressed (P< 0·05) the percentage of phagocytic heterophils and macrophages (Table 5). However, no significant effect of dietary Arg concentration on phagocytic capacity was observed (P>0·05).
Table 5 Effect of graded supplementation of arginine (Arg) (1·05, 1·42 or 1·90 %) on the phagocytic activity of splenic heterophils and macrophages of broiler chickens on day 7 after continuous lipopolysaccharide (LPS) injection (Expt 1)* (Mean values with their standard errors)
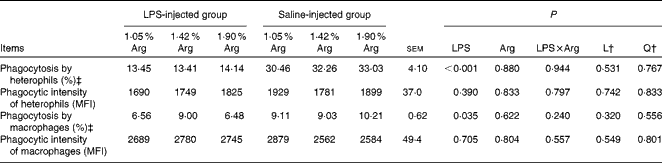
L, linear; Q, quadratic; MFI, mean fluorescence intensity.
* LPS injection: chickens were injected with LPS (1 mg/kg of BW) four times at 48 h intervals from 14 to 20 d; saline injection (control): chickens were injected with the same amount of sterile saline (0·9 %).
† L and Q contrasts on the main effect means for Arg concentrations (averaged over LPS treatments).
‡ Percentage of phagocytic-active cells.
Immune cell phenotypes
The effect of dietary Arg concentration on splenocyte phenotypes is summarised in Table 6. Increasing dietary Arg concentrations linearly (P< 0·05) reduced the percentages of splenic Bu1+, CD11+ and CD14+ cells (percentages of splenic lymphocytes), whereas no significant difference was found in the percentages of splenic CD3+ cells (P>0·05). Compared with the control treatment, LPS injection significantly reduced (P< 0·05) the percentages of Bu1+ and CD11+ cells in the spleen and tended to reduce the percentage of CD14+ cells (P< 0·10). No significant (P>0·05) difference was observed in the percentages of CD3+ cells. The percentage of total lymphocytes in the spleen (percentage of total splenocytes) was not influenced by either LPS injection or Arg supplementation (P>0·05).
Table 6 Effect of graded supplementation of arginine (Arg) (1·05, 1·42 or 1·90 %) on the percentages of splenic leucocytes expressing cell-surface antigens for CD3, B cells (Bu-1), CD11 and CD14 of broiler chickens on day 7 after continuous lipopolysaccharide (LPS) injection* (Expt 1) (Mean values with their standard errors)

L, linear; Q, quadratic.
* LPS injection: chickens were injected with LPS (1 mg/kg of BW) four times at 48 h intervals from 14 to 20 d; saline injection (control): chickens were injected with the same amount of sterile saline (0·9 %).
† L and Q contrasts on the main effect means for Arg concentrations (averaged over LPS treatments).
‡ Based on gated lymphocytes.
§ Based on total splenocytes.
Gene expression in the spleen and caecal tonsils
LPS injection significantly (P< 0·05) increased the expression of the pro-inflammatory cytokines IL-1β in the spleen and IL-6 in the caecal tonsils. Dietary Arg supplementation linearly decreased (P< 0·05) the expression of IL-1β in the spleen; however, no significant (P>0·05) effect was found on the expression of IL-6. LPS injection significantly (P< 0·05) reduced the expression of the anti-inflammatory cytokine IL-10 in the spleen, while increasing Arg concentrations linearly and quadratically (P< 0·05) decreased the expression of IL-10 in the caecal tonsils. LPS injection significantly increased the expression of TLR4 (P< 0·05) in the spleen and tended to up-regulate the expression of TLR4 in the caecal tonsils (P= 0·061), while dietary Arg supplementation linearly (P< 0·05) down-regulated the expression of TLR4 in the spleen and caecal tonsils. In the caecal tonsils, dietary Arg supplementation linearly and quadratically (P< 0·05) decreased the expression of NF-κB; however, no significant (P>0·05) effect was observed in the spleen. The expression of PPAR-γ tended (P= 0·052) to be reduced by LPS injection in the caecal tonsils. Dietary Arg supplementation quadratically (P< 0·05) reduced the expression of PPAR-γ in the spleen. There was a LPS × Arg interaction (P< 0·05) for the expression of IL-1β in the caecal tonsils, wherein dietary Arg supplementation had no significant (P>0·05) effect on the non-challenged chickens, whereas it linearly and quadratically (P< 0·05) reduced the expression of IL-1β in the caecal tonsils of the LPS-challenged chickens (Table 7).
Table 7 Effect of graded supplementation of arginine (Arg) (1·05, 1·42 or 1·90 %) on the relative mRNA expression of pro/anti-inflammatory cytokine and inflammatory regulation genes in the spleen and caecal tonsils of broiler chickens on day 7 after continuous lipopolysaccharide (LPS) injection* (Expt 1) (Mean values with their standard errors)
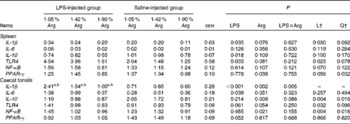
L, linear; Q, quadratic; TLR4, Toll-like receptor 4.
a,b Mean values within the LPS- or saline-injected groups have a linear and quadratic dose response to Arg concentrations (P< 0·05).
* LPS injection: chickens were injected with LPS (1 mg/kg of BW) four times at 48 h intervals from 14 to 20 d; saline injection (control): chickens were injected with the same amount of sterile saline (0·9 %).
† L and Q contrasts on the main effect means for Arg concentrations (averaged over LPS treatments).
Expt 2
With the increase in dietary Arg concentrations, the percentage of circulating B cells (percentage of circulating lymphocytes) decreased linearly (P< 0·01), quadratically (P< 0·01) and cubically (P< 0·05). The percentage of total circulating lymphocytes (percentage of isolated PBMC) was not influenced by the dietary Arg concentration (P>0·05; Fig. 1).
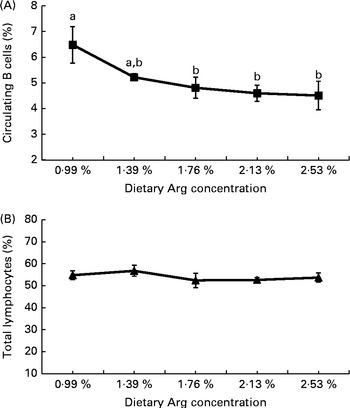
Fig. 1 Effect of dietary l-arginine (Arg) concentration on (A) the percentage of circulating B cells (based on gated total lymphocytes) and (B) the percentage of gated total lymphocytes (based on isolated peripheral blood mononuclear cells) (21 d, Expt 2). Values are means of ten individual chickens, with their standard errors represented by vertical bars. a,bMean values (■) with unlike letters were significantly different (P≤ 0·05). The percentage of circulating B cells decreased linearly (P= 0·002), quadratically (P= 0·002) and cubically (P= 0·022) with increasing dietary Arg concentrations (P< 0·01). No effect was observed on the percentage of gated total lymphocytes (P>0·05). (B) Linear (P= 0·171), quadratic (P= 0·315) and cubic (P= 0·253).
Discussion
Animals that are raised in an environment in which they are exposed to frequent microbial challenges grow at a slower growth rate and have lower nutrient utilisation, which cause a tremendous loss to poultry production( Reference Klasing and Barnes 28 ). Studies in mammals and birds have demonstrated that Arg is an important immune-modulatory nutrient, wherein dietary Arg supplementation could enhance both innate and adaptive immune functions of animals( Reference Bronte and Zanovello 2 , Reference Tan, Li and Kong 29 ). Arg supplementation has been used to alleviate inflammatory response in mammalian models( Reference Coburn, Gong and Singh 12 ). Although the immune system of birds, which is different from that of mammals, is well characterised, the regulatory function of Arg in the inflammatory response of chickens is still unknown. To evaluate whether Arg supplementation alleviates the inflammatory response in broiler chickens, a model for inducing inflammation in broilers by injecting E. coli LPS was used.
The suppression of the growth performance of animals reared in unsanitary environments is thought to be a result of excessive immune defence response, such as inflammation, which is marked by reduced FI and muscle protein accretion and wasted energy and protein for defence protein synthesis (e.g. acute-phase proteins)( Reference Klasing and Barnes 28 , Reference Cook 30 ). We employed a previously described inflammatory model wherein repeated injections of LPS resulted in a significant reduction in weight gain and FI( Reference Klasing and Barnes 28 , Reference Webel, Johnson and Baker 31 ), which is in agreement with the results of the present study. One may question whether LPS-induced reductions in performance are primarily a result of reductions in FI or excessive inflammatory response. A previous report from our laboratory( Reference Jiang, Schatzmayr and Mohnl 32 ) has demonstrated that compared with pair-feeding (the same amount of feed is fed), LPS injection significantly reduces BWG and increases the FCR of chickens (14–21 d), which provides evidence that activation of the inflammatory system of chickens is costly in terms of growth beyond that of only reducing FI. In the present study, LPS injection increased the expression of inflammatory cytokines IL-1β, IL-6 and TLR4, which suppressed growth processes by directing nutrients away from body protein accretion to support the immune response. Thus, if Arg supplementation could alleviate the inflammatory response of chickens, it could be used as an important component for designing immune-enhancing diets to diminish the economic loss caused by infection, which has been considered as an effective nutritional strategy to reduce infection rates and post-infection recovery( Reference Bansal, Syres and Makarenkova 33 , Reference Ochoa, Makarenkova and Bansal 34 ).
Phagocytosis is an innate immune reaction for the clearance of bacterial infections of animals, which plays an indispensable role in the defence against various infections( Reference Seifert, Fritz and Carlini 35 ). In chickens, heterophils are key components of the innate immune system that play a role similar to that of neutrophils in mammals( Reference Chuammitri, Redmond and Kimura 36 ). Heterophils are highly phagocytic and have a broad spectrum of antimicrobial activities( Reference Harmon 37 ). A previous study has reported that dietary Arg supplementation increases the proportion of circulating heterophils in chickens( Reference Lee, Austic and Naqi 38 ), but it failed to enhance the phagocytic activity of heterophils in the present study. Macrophages are a key arm of the innate immune defence system in intracellular bacterial killing( Reference Ibuki, Kovacs-Nolan and Fukui 39 ). The antimicrobial activities of macrophages are due to the generation of reactive oxygen species and reactive N species, such as H2O2 and NO, which are important metabolites of Arg( Reference Bronte and Zanovello 2 ). An in vitro study in channel catfish has suggested that supplementation of Arg to cell-culture media enhances the phagocytic activity of head kidney macrophages( Reference Pohlenz, Buentello and Mwangi 40 ), but no significant influence was observed in the present study. Central to inflammation is the recruitment of leucocytes to the site of infection, which is characterised by the migration of heterophils and macrophages from blood and spleen to the site of infection( Reference Gong, Hart and Shchurin 41 ). Therefore, LPS injection induced the migration of leucocytes from the spleen to the site of injection, which suppressed the phagocytic activity of heterophils and macrophages in the spleen.
It is well known that Arg plays an important role in the development of T cells( Reference Zea, Rodriguez and Culotta 42 ), as Arg deprivation arrests T cells in the G0–G1 phase of the cell cycle( Reference Rodriguez, Hernandez and Morrow 43 ), and Arg deficiency can have an unambiguous effect on early B-cell development( Reference Jonge, Kwikkers and Velde 44 ). In the present study, no significant effect of Arg deficiency was observed on the percentage of CD3+T cells, while increasing dietary Arg concentrations significantly reduced the percentages of both splenic and circulating B cells (P< 0·05). Previous studies have reported that H2O2 can cause the apoptosis of macrophages by mitochondrial membrane depolarisation( Reference Chaturvedi, Cheng and Asim 45 ). In our previous study (J Tan, Y Guo, TJ Applegate and E Du, unpublished results), dietary Arg supplementation quadratically (P< 0·05) increased in vitro macrophage H2O2 production. Thus, the decrease in the proportion of B cells may be associated with the increasing production of H2O2, which could be synthesised by Arg through a NO synthase-2-depedent metabolic pathway( Reference Bronte and Zanovello 2 ). It is well known that immune-modulating nutrients can be helpful as well as harmful if not utilised in moderation( Reference Klasing 46 ), such as n-3 PUFA, which could be used to reduce the adverse effects of infectious bursal disease( Reference Maroufyan, Kasim and Ebrahimi 47 ) and improve cellular immunity( Reference Wang, Ding and Wang 48 ) of chickens, while it increases the incidence of Marek's disease( Reference Klasing and Korver 49 ). Thus, further research should be conducted to gain a better understanding of the optimal immune response for dietary Arg supplementation to direct the immune system in an optimal direction.
Cytokines are small, non-structural proteins with molecular weights ranging from 8 to 40 000 Da. Pro-inflammatory cytokines such as IL-1β, IL-6 and TNF-α promote inflammation, whereas anti-inflammatory cytokines such as IL-4, IL-10 and IL-13 suppress inflammation( Reference Opal and DePalo 50 ). LPS injection has been used to trigger pro-inflammatory cytokine response in chickens( Reference Munyaka, Tactacan and Jing 15 ), which was considered to be a result of TLR4 activation( Reference Keestra and Putten 14 ). When expressed in excess, pro-inflammatory cytokines may cause fever, inflammation and tissue destruction( Reference Dinarello 51 ). In the present study, the overexpression of IL-1β in the spleen and caecal tonsils was alleviated by dietary Arg supplementation, indicating that Arg supplementation may have a beneficial effect on the attenuation of the inflammatory response induced by LPS injection.
IL-10 is a critical anti-inflammatory cytokine secreted by activated macrophages that serves as a feedback response of inflammation to prevent the excessive expression of pro-inflammatory cytokines. T helper (Th) 2 cytokines, such as IL-10 and IL-4, could activate arginase-1 in macrophages( Reference Popovic, Zeh and Ochoa 1 ), which converts Arg into ornithine, and ornithine can be decarboxylated by ornithine decarboxylase to produce putrescine and further converted into polyamines (spermidine and spermine). Polyamines have been reported to be important inhibitory substances for the LPS-induced expression of pro-inflammatory cytokines( Reference Bossche, Lamers and Koehler 52 ). In the present study, LPS injection decreased the expression of IL-10, which is in agreement with the findings of a previous study( Reference Shanmugasundaram and Selvaraj 53 ). However, dietary Arg supplementation failed to enhance the expression of IL-10. PPAR are members of the nuclear hormone receptor family. PPAR ligands, in particular, PPAR-γ ligands, could inhibit inflammatory response and negatively regulate pro-inflammatory transcription factor signalling pathways in inflammatory cells( Reference Moraes, Piqueras and Bishop-Bailey 54 ). Nutritional dietary strategies have been described to enhance the expression of PPAR-γ, which exerts a beneficial effect on the alleviation of the inflammatory response induced by LPS injection( Reference Zhang, Guo and Yang 55 ). However, dietary Arg supplementation failed to enhance the expression of PPAR-γ. Thus, Arg does not modulate the inflammatory response through anti-inflammatory cytokines and the PPAR-γ pathway.
Chicken CD14 is 34 % homologous to human orthologues at the protein level, and they share several structural features such as conserved leucine-rich repeats and conserved cysteines( Reference Wu, Rothwell and Hu 56 ). Mammalian CD14 is expressed as a glycosylphosphatidylinositol (GPI) anchored cell-surface protein that can be released as a soluble serum protein (sCD14)( Reference Schutt 57 ). In the absence of chicken serum, LPS induced the up-regulation of pro-inflammatory cytokine and chemokine gene expression in chicken heterophils, indicating that LPS can be directly recognised by CD14 or TLR4, while the presence of chicken serum or mouse LBP dramatically enhanced the activation of heterophils, suggesting that a functional protein similar to mammalian LBP may exists in chicken serum( Reference Kogut, He and Kaiser 58 ). Chicken CD14 plays an unclear role in LPS sensing, while chicken CD14 is essential for the activation of LPS-induced pro-inflammatory cytokine response( Reference Brownlie and Allan 59 ). Panaro et al. ( Reference Panaro, Cianciulli and Gagliardi 60 ) reported that the activation of chicken embryo cardiomyocyte inflammation by LPS requires the engagement of both TLR4 and CD14, since anti-CD14 pretreatment of cardiomyocytes prevents the production of pro-inflammatory cytokines. A similar finding has been reported for chicken macrophages, with the administration of either anti-CD14 or anti-TLR4 antibodies completely blocking LPS-mediated inducible NO synthase (iNOS) activation, suggesting that both CD14 and TLR4 are required for LPS-mediated iNOS activation( Reference Dil and Qureshi 61 ). The inhibition of CD14, such as with an anti-CD14 antibody, has been used as a therapeutic approach to protect primates from LPS-induced shock due to overexpression of inflammatory cytokines( Reference Leturcq, Moriarty and Talbott 62 ). In the present study, increasing dietary Arg concentrations linearly reduced the percentage of CD14+ cells in the spleen. Therefore, Arg supplementation could be suggested as a potential nutritional approach for the mediation of a hyper-inflammatory response. Since the exact role that chicken CD14 plays in inflammatory response is still unclear, further studies are needed to get the whole picture.
Chicken TLR4 is about 43 % homologous to human orthologues at the protein level( Reference Brownlie and Allan 59 ). Chicken sense LPS via the TLR4 and myeloid differentiation protein-2 complex and further activate the NF-κB inflammatory cascade( Reference Keestra and Putten 14 , Reference Zoete, Keestra and Roszczenko 63 ). Compared with mammalian species, chicken lack the myeloid differentiation factor 88 (MyD88)-independent signalling pathway in which the downstream production of interferon-β occurs in mammals( Reference Keestra and Putten 14 ). Mammalian TLR4/myeloid differentiation protein-2 complex depends on two different intracellular signalling routes for NF-κB activation: the MyD88/Toll-IL 1 receptor domain-containing adaptor protein (TIRAP) and TRAM/TRIF (TRIF-related adaptor molecule/Toll-IL 1 receptor domain-containing adaptor inducing interferon) pathways( Reference O'Neill and Bowie 64 ). As chickens lack an orthologue of TRAM that bridges TLR4 and TRIF, chicken TLR4 signals only by the MyD88/TIRAP-dependent pathway( Reference Keestra, Zoete and Bouwman 65 ). The result reported herein showed that LPS injection increased the expression of TLR4 mRNA in the spleen and caecal tonsils and activated the pro-inflammatory cytokine response. Interestingly, dietary Arg supplementation suppressed the expression of TLR4 mRNA linearly. Thus, the suppression of the TLR4 pathway could be one of the mechanisms by which Arg alleviates inflammatory response. In subclinical conditions, the local inflammatory response recruits other phagocytic or non-phagocytic lymphocytes to eliminate foreign pathogens, which is very important for host defence. Therefore, under these conditions, the suppression of the TLR4 pathway and CD14+ cell percentage could be a potential negative effect.
In cells (e.g. macrophages and myeloid cells), Arg is mainly metabolised by iNOS or by arginase-1 to synthesise NO and polyamines, respectively( Reference Popovic, Zeh and Ochoa 1 ). iNOS and arginase-1 are stimulated by Th1 and Th2 cytokines, respectively( Reference Bronte and Zanovello 2 ). Dietary Arg supplementation failed to enhance the expression of Th2 cytokines (IL-2 and IL-10) in the present study; thus, Arg may not exert its inflammation-modulatory effects through the arginase-1 pathway. Previous work has shown that oral administration of Arg attenuates LPS-induced lung chemokine production in mice( Reference Calkins, Bensard and Heimbach 4 ). This effect is dependent on NO and is mediated by the stabilisation of inhibitory κB-α concentrations. Studies in mice have also shown that Arg supplementation could alleviate the inflammatory response in dextran sulphate sodium colitis, while this benefit has been found to be eliminated when tested in the iNOS –/– mice( Reference Coburn, Gong and Singh 12 ). Furthermore, a study in chickens has shown that dietary Arg supplementation could increase circulating NO production( Reference Khajali, Tahmasebi and Hassanpour 66 ); thus, we conclude that the beneficial effect of Arg on the alleviation of inflammatory response depends on NO through the iNOS pathway. Regretfully, in the present study, no direct evidence was obtained to prove whether Arg has a direct effect or acts through NO on the percentages of CD11+ and CD14+ cells and expression of TLR4, thus, requiring further studies.
In summary, the present study demonstrates that dietary Arg supplementation alleviated systemic inflammation of LPS-challenged chickens. It is possible that the ability of Arg to alleviate systemic inflammation is associated with the reduction in the expression of pro-inflammatory cytokines via the suppression of the TLR4 pathway and percentage of CD14+ cells (percentage of splenic lymphocytes). The results obtained in the present study clarified the molecular events by which Arg alleviated the systemic inflammatory response caused by LPS injection. Furthermore, excessive dietary Arg supplementation suppressed the percentages of both splenic (1·90 %) and peripheral blood B cells (1·76 %) (percentage of splenic and circulating lymphocytes).
Acknowledgements
The authors thank Bertrand Grenier, Sunday A. Adedokun, Qian Zhang, Xi Chen and Kolapo Ajuwon for their help with the experiments.
The present study was supported by a grant from the China Agriculture Research System (CARS-42-G13) and China Scholarship Council. The funders (China Agricultural University and China Scholarship Council) had no role in the design or analysis of the study and in the writing of this article.
Mention of trade names or commercial products in this article is solely for the purpose of providing specific information and does not imply recommendation or endorsement by the US Department of Agriculture.
USDA is an equal-opportunity employer.
Data reported herein were partially presented at the 2013 PSA Annual Meeting, San Diego, CA (July 2013).
The authors' contributions are as follows: J. T. conducted the animal trial, carried out the sample analyses and wrote the manuscript; S. L. assisted with all the data analyses; T. J. A. contributed to the study design, conduct of the study and preparation of the manuscript; Y. G. and S. D. E. contributed to the experimental design and manuscript preparation.
There is no conflict of interest to be disclosed.