Researchers have observed that at least 13·7 million infants are born with a low birth weight each year, accounting for about 11 % of newborns in developing countries( Reference De, Blossner and Villar 1 ). The probability of intra-uterine growth retardation (IUGR) in developing countries is approximately six times higher than that in developed countries. IUGR is defined as growth and development of a fetus and/or its organ that is less than that of a normal fetus during gestation. The most notable and direct feature of IUGR neonates is the lower birth weight, mainly owing to placental insufficiency( Reference Hay, Thureen and Anderson 2 ). During the critical stage of fetal growth and development, nutritional deficiencies may cause alterations in tissue and organ function, which has long-term effects( Reference Varvarigou 3 ). Barker et al.(4) originally stated the ‘early’ or ‘fetal’ origins of adult disease hypothesis. The hypothesis described that environmental factors, particularly the nutritional factor, acted during early life to programme the risks for the early onset of metabolic disease into adult life and premature death. It is obvious that IUGR has become a big problem for humans, especially in developing countries, and has adverse effects on children during their childhood and adulthood( Reference Mcmillen and Robinson 5 ). Numerous epidemiological studies of people from all over the world, including those in the USA, China, India and Australasia, have also strongly supported the hypothesis that IUGR could lead to a low birth weight initially, thus increasing the risk of developing metabolic diseases in adulthood( Reference Hales and Barker 6 ). Current data suggest that IUGR down-regulated the levels of proteins that regulated immune function and oxidative defence, and impaired liver function was found in newborn piglets with IUGR( Reference Junjun, Lixiang and Defa 7 ). Results also suggested that a stronger pro-inflammatory bias existed in peripheral blood lymphocytes of women with a diagnosis of IUGR( Reference Raghupathy, Al-Azemi and Azizieh 8 ). Reports also showed that the hepatocytes of IUGR fetuses might suffer from oxidative stress, thereby decreasing their liver detoxifying abilities( Reference Junjun, Lixiang and Defa 7 ). Although IUGR has emerged as a leading cause of neonatal morbidity and mortality, research on fetuses is extremely hard and complex during pregnancy. Thus, studies on IUGR are mainly focused on its postnatal treatment.
Curcumin (C21H20O6), a yellow pigment from turmeric (Curcuma longa), is a naturally occurring phenolic compound, which is widely used in food, beverages, medicines and so forth( Reference Aggarwal, Sundaram and Malani 9 ). Curcumin, first described in 1910 by Lampe and Milobedeska, is the most active ingredient in turmeric and accounts for 2–5 % of this spice( Reference Miłobȩdzka, Kostanecki and Lampe 10 ). Previous studies have found that curcumin plays an important role in the inhibition of platelet aggregation, tumorigenesis, oxidation, inflammatory cytokines and myocardial infarction( Reference Ghorbani, Hekmatdoost and Mirmiran 11 ). Curcumin is also known to possess the capacity for anti-inflammatory( Reference Wang, Chen and Chen 12 ), antioxidant( Reference Somparn, Phisalaphong and Nakornchai 13 ), anti-cancer, anti-tumour activities and has other potential protective effects against various diseases( Reference Ruby, Kuttan and Babu 14 ). In human applications, it has been demonstrated to be safe at dosages of up to 8 g/d( Reference Mazidi, Karimi and Meydani 15 ). In recent years, researchers have revealed that curcumin had protective effects against oxidative stress through multiple mechanisms, including the elevation of antioxidant enzyme activity, attenuation of mitochondrial dysfunction and reduction in liver injury( Reference Zhang, Xu and Zhang 16 , Reference Molina-Jijon, Tapia and Zazueta 17 ). Studies also revealed that diets supplemented with 0·05 % curcumin could attenuate oxidative stress and inflammation in rats( Reference Kowluru and Kanwar 18 ).
The maternal rat used as an animal model to create IUGR newborns has been widely recognised( Reference Desai, Gayle and Babu 19 ). During the selected period of pregnancy, maternal nutrition deficiencies or malnutrition have been illustrated to lead to growth retardation of rats( Reference Widdowson and McCance 20 ). It is generally known that IUGR can have harmful effects. However, more effective nutritional treatments are yet to be studied. To the best of our knowledge, the studies on the effects of curcumin on IUGR are limited. To test this hypothesis, we chose newborn rats with a low birth weight as the IUGR models to investigate whether IUGR could induce inflammation, oxidative damage and injury in the liver. We also observed the effects of dietary 400 mg curcumin/kg diet supplementation on IUGR.
Methods
Curcumin preparation
The curcumin used in this study was kindly provided by the Kehu Bio-technology Research Center.
Animal experiment design
The experimental design and procedures were approved by the Institutional Animal Care and Use Committee of Nanjing Agricultural University following the requirements of the Regulations for the Administration of Affairs Concerning Experimental Animals of China. The feed restriction method was used for maternal rats during pregnancy to create an IUGR mode based on a previous study( Reference Desai, Gayle and Babu 19 ). This method was easily controlled by us and based on our previous experiences with bilateral artery ligation and protein restriction models. First-time-pregnant Sprague–Dawley rats (Nanjing Qinglongshan Experiment Animal Center) were housed in a facility at a constant temperature and humidity and a controlled 12 h light–12 h dark cycle. At day 10 of gestation, rats were provided either a diet of standard laboratory chow (LabDiet 5001) (n 12) ad libitum or a 50 % feed-restricted (n 12) diet determined by the quantification of normal feed intake in ad libitum-fed rats.
In Experiment 1, maternal rats gave birth normally, and birth weights of newborns were recorded on day 1 of postnatal life and divided into normal or IUGR groups. Mice were limited to 10/litter to normalise rearing (average 11·83 and 12·1 mice/litter in normal and IUGR groups, respectively). During the 21-day lactation period, each litter of mice from the normal or IUGR group was nursed by their own maternal rats. At 3 weeks of age, offspring in each litter were weaned and housed individually until they were 6 weeks old. Each litter from the normal and IUGR groups was weighed, and the weight of an individual pup was calculated from the group (i.e. litter weight/number of pups). The weights of pups were recorded at 1, 21, 28, 35 and 42 d of age.
In Experiment 2, at 6 weeks of age, twenty-four female rats with nearly equal body weights (within each group) were allocated to the normal birth weight (NBW), NBW with curcumin supplementation (NC), IUGR and IUGR with curcumin supplementation (IC) groups (one rat per litter, n 6/group), respectively. The rats were allowed water and a standard granulated diet (AIN-93 diet)( Reference Reeves, Nielsen and Fahey 21 ) ad libitum. During the entire experimental period, rats in the NC and IC groups were fed a standard diet supplemented with 400 mg curcumin/kg. Curcumin was added to the feed before it was made into pellets. The light regimen was a 12 h light–12 hdark cycle and the temperature was maintained at 22±2°C. Body weights of each group were recorded at 7, 8, 9, 10, 11 and 12 weeks of age. At 12 weeks of age, all rats were fasted overnight, and blood was collected by cardiac puncture after anaesthesia. Serum was obtained from the blood by centrifugation for 15 min at 3000 g at 4°C. Liver tissue was removed after death, weighed and snap-frozen in liquid N2 and then stored at −80°C for further analysis. A portion of liver tissue (the same area for each sample) was removed and fixed in formalin for histologic assessment.
Analysis of serum TNF-α, IL-1β and IL-6
Concentrations of TNF-α, IL-1β and IL-6 in the serum were determined by ELISA kits from Shanghai YILI Biological Technology Co., Ltd.
Analysis of serum aminotransferase activities
Activities of serum aspartate aminotransferase (AST) and alanine aminotransferase (ALT) were measured via the enzymatic kinetic method using an automatic biochemistry analyser (SELECTA XL; Vital Scientific) according to the manufacturer’s protocol( Reference Zhang, Xu and Zhang 16 ).
Liver histologic evaluation
Liver tissue specimens from the right lobe were fixed immediately in 10 % formalin, embedded in paraffin and 6-μm sections were cut and stained with haematoxylin–eosin (H&E)( Reference Toydemir, Kanter and Erboga 22 ). After H&E staining, a morphologic evaluation was carried out using a light microscope (Nikon ECLIPSE 80i; Nikon Corporation). The section of each animal was counted at least three times.
Analysis of lipid peroxidation, protein and DNA oxidation levels
Lipid peroxidation levels were expressed by malondialdehyde (MDA) concentration, which is a byproduct of lipid peroxidation. Concentrations of MDA in the liver were determined by an assay kit (Nanjing Jiancheng Bioengineering Institute). Protein oxidation in the liver was estimated via the concentration of protein carbonyl (PC) according to a previous study( Reference Caprau, O’Grady and Callaway 23 ). For the measurement of endogenous oxidative DNA damage, 8-hydroxy-2'-deoxyguanosine (8-OHDG) was used as a biomarker. Concentrations of 8-OHDG in the liver were determined by ELISA kits from Shanghai YILI Biological Technology Co., Ltd.
Assay of antioxidant enzyme activities in the serum and liver
The liver samples from −80°C were homogenised with 0·86 % (w/v) ice-cold physiological saline or tissue homogenate provided by the corresponding diagnostic kit (Nanjing Jiancheng Bioengineering Institute) according to the instructions of the manufacturer. Liver samples were homogenised using an Ultra-Turrax homogenizer (Tekmar) at 13 500 g for 1 min in ice-cold water. Then, the homogenate was centrifuged at 3000 g for 15 min at 4°C, and the supernatant was analysed quickly. All results were normalised to total protein concentrations in each sample for inter-sample comparisons. The levels of GSH and glutathione peroxidase (GPX) in the serum, and activities of superoxide dismutase (SOD), total antioxidant capacity (T-AOC), glutathione reductase (GR), GPX and concentrations of GSH and GSSG in the liver were determined using assay kits (Nanjing Jiancheng Bioengineering Institute) according to the manufacturer’s instructions.
Assay of gene expression
Total RNA from the liver samples stored at −80°C was isolated using the TRIzol reagent (Invitrogen). The determination of RNA content, mRNA quantification and real-time PCR (Applied Biosystems) were performed according to previously described methods(
Reference He, Dong and Xu
24
). The primer sequences for the target and housekeeping genes (Tnfa, Il1b, Il6, nuclear factor, erythroid 2-like 2 (Nfe2l2), NAD(P)H dehydrogenase, quinone 1 (Nqo1), haeme oxygenase 1 (Hmox1), glutathione S transferase (Gst), glutathione peroxidase 1 (Gpx1), superoxide dismutase 1 (Sod1) and β-actin (Actb)) used for real-time PCR are listed in Table 1. Gapdh was also used as a control gene to normalise the expression of target genes. Briefly, a reaction system of 20 μl was composed of 0·4 μl of forward primers, 0·4 μl of reverse primers, 0·4 μl of ROX Reference Dye, 10 μl of SYBR Premix Ex Taq (TaKaRa Biotechnology Co. Ltd), 6·8 μl of double-distilled water and 2 μl of complementary DNA. The
$$2^{{{\minus}\Delta \Delta C_{t} }} $$
method was used to calculate relative levels of mRNA expression after normalisation with housekeeping genes(
Reference Schmittgen and Livak
25
). The values for the NBW group were used for calibration.
Table 1 Primer sequences used for quantitative real-time PCR assays

F, forward; R, reverse; Nfe2l2, nuclear factor, erythroid 2-like 2; Nqo1, NAD(P)H dehydrogenase, quinone 1; Hmox1, haeme oxygenase 1; Gst, glutathione S transferase; Gpx1, glutathione peroxidase 1; Sod1, superoxide dismutase 1; Actb, β-actin.
Western blotting
Antibodies against total NF-κB (dilution 1:500) and phosphorylated NF-κB (dilution 1:500) were purchased from Bioworld. Antibodies against total Janus kinase 2 (JAK2, dilution 1:1000), signal transducer and activator of transcription 3 (STAT3, dilution 1:1 000), IκBα (dilution 1:500) and phosphorylated JAK2 (dilution 1:1 000), STAT3 (dilution 1:500) and IκBα (dilution 1:1 000) were purchased from Abcam. The protein (total, nuclear or cytoplasmic) of the liver was extracted using assay kits according to the manufacturer’s instructions (Beyotime). The protein content of the sample was assayed using the BCA Protein Assay Kit (Beyotime). For Western blotting analyses, 60 μg of protein from each sample was subjected to sodium dodecylsulphate–PAGE. After electrophoresis, proteins were separated and transferred to polyvinylidene difluoride membranes. The membranes were blocked with blocking buffer (5 % non-fat dry milk) for 12 h at 4°C. The membranes were probed with appropriate primary and secondary antibodies (horseradish-peroxidase-conjugated goat anti-rabbit Ig G; Bioworld; 1:10 000 dilution in 1×TBS with 0·1 % Tween 20). The blots were detected using enhanced chemiluminescence reagents (ECL-Kit; Beyotime) followed by autoradiography. Photographs of the membranes were taken using the Luminescent Image Analyzer LAS-4000 system (Fujifilm Co.) and quantified by the Gel-Pro Analyzer 4.0 software (Media Cybernetics).
Statistical analysis
Body weights of NBW and IUGR mice at 1 d and from 1 to 6 weeks of age were analysed using unpaired, independent t tests. Body weights from 6 to 12 weeks of age and measurements at 12 weeks of age were analysed using a two-way ANOVA. The classification variables were birth weight (NBW+NC×IUGR+IC), diet (NBW+IUGR×NC+IC) and the interaction between birth weight and diet (NBW×NC×IUGR×IC). A Tukey’s post hoc analysis was used to determine the differences between the four groups when a statistically significant birth weight×diet interaction was observed. SPSS 17.0 (SPSS Inc.) was used for these analyses. A probability level of P<0·05 was considered statistically significant, and P<0·01 was considered very significant. Data are presented as means and standard deviations.
Results
Growth analysis
Offspring of IUGR rats were born smaller (P=0·005) than those of NBW rats (5·546 (sd 0·264) v. 6·314 (sd 0·358) g; Fig. 1(a)) and their body weights remained lower than those of the NBW group at 3, 4, 5 and 6 weeks of age (P=0·127, P=0·062, P=0·481 and P<0·001, respectively). Body weights of rats in NBW and IUGR groups increased at 10 (242·967 (sd 19·242) v. 237·333 (sd 12·138) g, P>0·05; 225·533 (sd 8·454) v. 221·100 (sd 9·291) g, P>0·05) and 11 weeks (252·867 (sd 19·307) v. 247·267 (sd 14·418) g, P>0·05; 236·933 (sd 9·915) v. 230·283 (sd 12·666) g, P>0·05) owing to dietary curcumin supplementation (Fig. 1(b)).

Fig. 1 Growth analysis of offspring with normal body weights (NBW) or intra-uterine growth retardation (IUGR) during the early period of life (a) and diets supplemented with curcumin (Cur) from 6 to 12 weeks of age (b). Values are means and standard deviations. a: and
, NBW;
and
, IUGR; b:
, NBW;
, NBW+Cur;
, IUGR;
, IUGR+Cur. Body weights of NBW and IUGR mice at 1 d of age and from 1 to 6 weeks of age were analysed using unpaired independent t tests. Body weights from 6 to 12 weeks of age were analysed by using two-way ANOVA, n 6. * Significant difference was observed (P<0·05).
Relative weight of liver
At 12 weeks of age, liver weights of IUGR rats were obviously lower (P<0·05) than those of NBW rats (Table 2). Curcumin treatment did not improve (P<0·05) liver weight or relative liver weight.
Table 2 Effect of curcumin on the liver weight and liver relative weight of rats with intra-uterine growth retardation (IUGR) (12 weeks of age)Footnote * (Mean values and standard deviations; n 6 per group)

NBW, normal birth weight rats; NC, normal birth weight rats fed diets supplemented with 400 mg/kg curcumin; IC, IUGR rats fed diets supplemented with 400 mg/kg curcumin;
B, birth weight; D, dietary curcumin supplementation; B×D, interaction between the corresponding parameters; BW, body weight; LRW, relative weight of liver.
a,b,c,d Mean values within a row with unlike superscript letters were significantly different when a significant interaction was observed (P<0·05).
* Data were analysed by using two-factor ANOVA and Duncan’s post hoc testing, where appropriate.
† The relative weight of liver is equal to the liver weight to per 100 g BW.
Levels of serum inflammation cytokines
IUGR rats exhibited significantly higher (P<0·05) concentrations of serum TNF-α, IL-1β and IL-6 compared with NBW rats (Fig. 2(A)–(C)). Curcumin supplementation reduced the concentrations of serum TNF-α (P=0·077), IL-1β (P=0·007) and IL-6 (P=0·002) in NBW or IUGR rats. In addition, a birth weight×dietary interaction effect (P<0·05) was noted for concentrations of TNF-α and IL-6 in the liver. There were no significant differences in the concentrations of serum inflammation cytokines among NBW, NC and IC groups (P>0·05).

Fig. 2 Concentrations of TNF-α (A), IL-1β (B) and IL-6 (C) in the serum of normal birth weight rats (N), normal birth weight rats supplemented with curcumin (NC), intra-uterine growth retardation (IUGR) rats (I) and IUGR rats supplemented with curcumin (IC) (12 weeks of age). Values are means (n 6) and standard deviations. , Normal birth weight;
, IUGR; B, birth weight; D, diet; B×D, interaction between the corresponding parameters. Data were analysed by using two-way ANOVA.
a,b Mean values with unlike letters were significantly different when a significant interaction was observed (P<0·05).
Activities of serum aspartate aminotransferase and alanine aminotransferase
The activity of serum AST was higher in the IUGR group (P>0·05) than in the NBW group (Fig. 3(A)). Dietary curcumin supplementation significantly decreased the activity of serum AST in the IUGR group (P<0·05). The activity of serum ALT was higher in the IUGR group than in the NBW group (P<0·05) (Fig. 3(B)). Because of dietary curcumin supplementation, the activity of ALT was significantly lower in the NC and IC groups than in the NBW and IUGR groups (P<0·01).

Fig. 3 Activities of aspartate aminotransferase (AST) (A) and alanine aminotransferase (ALT) (B) in the serum of normal birth weight rats (N), normal birth weight rats supplemented with curcumin (NC), intra-uterine growth retardation (IUGR) rats (I) and IUGR rats supplemented with curcumin (IC) (12 weeks of age). Values are means (n 6) and standard deviations. , Normal birth weight;
, IUGR; B, birth weight; D, diet; B×D, interaction between the corresponding parameters. Data were analysed by using two-way ANOVA. a,b Mean values with unlike letters were significantly different when a significant interaction was observed (P<0·05).
Liver histological changes
Normal histologic structures were observed in the liver of NBW and NC rats (Fig. 4(a) and (b)). In the liver sections of IUGR rats, vacuolisation and cell oedema were prevalent in the hepatocytes, and fat cells showed signs of mild denaturation (Fig. 4(c)). In the IC group, vacuolisation was significantly reduced and no fat cells were observed (Fig. 4(d)).
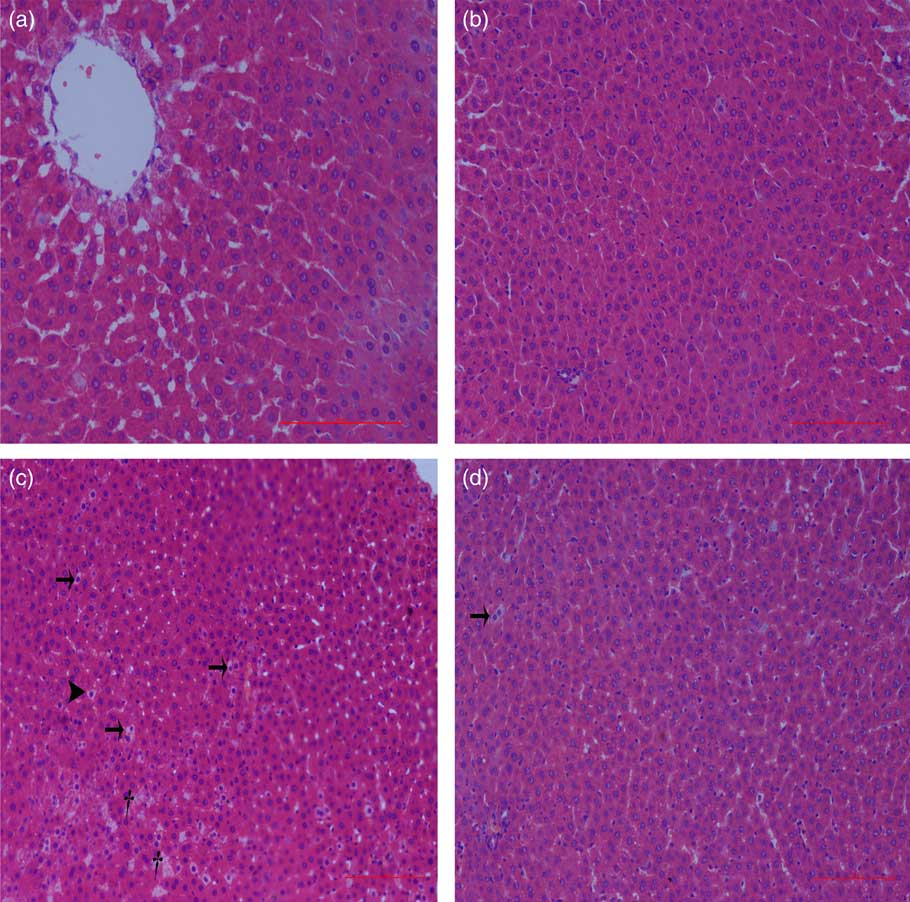
Fig. 4 Light microscopy of liver tissue in different groups (200× magnification): (a) normal birth weight rats, (b) normal birth weight rats supplemented with curcumin, (c) intra-uterine growth retardation rats and (d) intra-uterine growth retardation rats supplemented with curcumin. Haematoxylin–eosin, scale bar=100 μm. † Vacuole; , fat cell;
, cellular oedema.
Hepatic lipid peroxidation, protein and DNA oxidation levels
The concentrations of MDA, PC and 8-OHDG in the liver of IUGR rats were significantly higher (P<0·05) than those of NBW rats (Fig. 5(A)–(C)). In addition, a birth weight×dietary interaction effect was noted for liver concentrations of MDA and 8-OHDG in rats. Dietary curcumin supplementation significantly decreased the concentrations of MDA, PC and 8-OHDG in the liver of IUGR rats (P<0·05).

Fig. 5 Concentrations of malondialdehyde (MDA) (A), protein carbonyl (PC) (B) and 8-hydroxy-2'-deoxyguanosine (8-OHDG) (C) in the liver of normal birth weight rats (N), normal birth weight rats supplemented with curcumin (NC), intra-uterine growth retardation (IUGR) rats (I) and IUGR rats supplemented with curcumin (IC) (12 weeks of age). Values are means (n 6) and standard deviations. , Normal birth weight;
, IUGR; B, birth weight; D, diet; B×D, interaction between the corresponding parameters. Data were analysed by using two-way ANOVA. a,b,c Mean values with unlike letters were significantly different when a significant interaction was observed (P<0·05).
Hepatic antioxidant defence capacity
The IUGR group showed lower concentrations (P<0·01) of GSH and activity of GPX (P<0·1) in the serum of rats compared with the NBW group (Table 3). In the IC group, the concentration of GSH and activity of GPX in the serum of rats were higher (P<0·01) than those in the IUGR group. However, those of the NBW group were higher (P<0·01) than those of the NC group. A birth weight×dietary interaction effect was noted for activities of SOD, GR and GPX; concentrations of GSH and GSSG; and the value of GSSG:GSH in the liver of rats. The IUGR group showed lower (P<0·05) SOD, GR and GPX activities; lower GSH concentrations; and higher (P<0·05) GSSG concentration and GSSG:GSH value in the liver. In the IC group, the activity of GR and concentration of GSH in the liver were higher (P<0·05), and the concentration of GSSG and value of GSSG:GSH in the liver were lower (P<0·05) compared with those in the IUGR group. The NC group had lower (P<0·05) activities of SOD, GR and GPX; decreased concentrations of GSH (P<0·05); and a higher (P<0·05) GSSG:GSH value in the liver than in the NBW group.
Table 3 Effect of curcumin on the hepatic antioxidant defence capacity of rats with intra-uterine growth retardation (IUGR) (12 weeks of age)Footnote * (Mean values and standard deviations; n 6 per group)

NBW, normal birth weight rats; NC, normal birth weight rats fed diets supplemented with 400 mg/kg curcumin; IC, IUGR fed diets supplemented with 400 mg/kg curcumin; B, birth weight; D, dietary curcumin supplementation; B×D, interaction between the corresponding parameters; GPX, glutathione peroxidase; T-AOC, total antioxidant capacity; SOD, superoxide dismutase; GR, glutathione reductase; I, IUGR.
a,b,c Mean values within a row with unlike superscript letters were significantly different when a significant interaction was observed (P<0·05).
* Data were analysed by using two-factor ANOVA and Duncan’s post hoc testing, where appropriate.
Gene expression
In the liver of the IUGR group, the mRNA expression level for Il6 was higher (P<0·05) and the mRNA expression levels for Gst (P<0·05) and Nfe2l2, Hmox1, Gpx1 and Sod1 (P>0·05) were lower than those of the NBW group (Table 4). Dietary curcumin supplementation significantly decreased (P<0·05) the mRNA expression for Il1b, increased (P<0·05) the mRNA expressions for Nfe2l2, Nqo1, Gst and Gpx1, and had a tendency to decrease (P>0·05) the mRNA expressions for Tnfa and Il6 and to increase (P>0·05) the mRNA expressions for Hmox1 and Sod1 in the IC group. A birth weight×dietary interaction effect was noted for the mRNA expressions for Gst (P=0·001) in the liver of rats.
Table 4 Effect of curcumin on the hepatic gene expressions of rats with intra-uterine growth retardation (IUGR) (12 weeks of age)Footnote * (Mean values and standard deviations; n 6 per group)

NBW, normal birth weight rats; NC, normal birth weight rats fed diets supplemented with 400 mg/kg curcumin; IC, IUGR rats fed diets supplemented with 400 mg/kg curcumin;
B, birth weight; D, dietary curcumin supplementation; B×D, interaction between the corresponding parameters; Nfe2l2, nuclear factor, erythroid 2-like 2; Nqo1, NAD(P)H dehydrogenase, quinone 1; Hmox1, haeme oxygenase 1; Gst, glutathione S transferase; Gpx1, glutathione peroxidase 1; Sod1, superoxide dismutase 1.
a,b,c Mean values within a row with unlike superscript letters were significantly different when a significant interaction was observed (P<0·05).
* Data were analysed by using two-factor ANOVA and Duncan’s post hoc testing, where appropriate.
Phosphorylation levels of IκBα, NF-κB, Janus kinase 2 and signal transducer and activator of transcription 3
The IUGR rats exhibited higher (P<0·05) phosphorylated IκBα (Fig. 6) and nuclear phosphorylated NF-κB (Fig. 7) levels and lower (P<0·05) cytoplasmic phosphorylated NF-κB (Fig. 8) levels in the liver than in the NBW rats. In addition, the phosphorylated levels of JAK2 (Fig. 9) and STAT3 (Fig. 10) were higher (P=0·24, P=0·01) in the IUGR rats than in the NBW and NC groups. Dietary curcumin supplementation decreased the phosphorylated levels of IκBα (P<0·05) and JAK2 (P>0·05), and NF-κB (P>0·05) in the nucleus and cytoplasm of liver in the IC group.

Fig. 6 Abundance of phosphorylated IκBα in liver of normal birth weight rats (N), normal birth weight () rats supplemented with curcumin (NC), intra-uterine growth retardation (IUGR,
) rats (I) and IUGR rats supplemented with curcumin (IC) (12 weeks of age). B, birth weight; D, diet; B×D, interaction between the corresponding parameters. Results were corrected for cytoplasmic protein, n 6/group. a,b Mean values with unlike letters were significantly different when a significant interaction was observed (P<0·05).

Fig. 7 Abundance of phosphorylated NF-κB in the nucleus of normal birth weight () rats (N), normal birth weight rats supplemented with curcumin (NC), intra-uterine growth retardation (IUGR,
) rats (I) and IUGR rats supplemented with curcumin (IC) (12 weeks of age). B, birth weight; D, diet; B×D, interaction between the corresponding parameters. Results were corrected for nuclear protein, n 6/group. a,b Mean values with unlike letters were significantly different when a significant interaction was observed (P<0·05).

Fig. 8 Abundance of phosphorylated NF-κB in the cytoplasm of normal birth weight () rats (N), normal birth weight rats supplemented with curcumin (NC), intra-uterine growth retardation (IUGR,
) rats (I) and IUGR rats supplemented with curcumin (IC) (12 weeks of age). B, birth weight; D, diet; B×D, interaction between the corresponding parameters. Results were corrected for nuclear protein, n 6/group. a,b Mean values with unlike letters were significantly different when a significant interaction was observed (P<0·05).

Fig. 9 Abundance of phosphorylated Janus kinase 2 (JAK2) in liver of normal birth weight rats (N), normal birth weight () rats supplemented with curcumin (NC), intra-uterine growth retardation (IUGR,
) rats (I) and IUGR rats supplemented with curcumin (IC) (12 weeks of age). B, birth weight; D, diet; B×D, interaction between the corresponding parameters. Results were corrected for total protein, n 6/group.

Fig. 10 Abundance of phosphorylated signal transducer and activator of transcription 3 (STAT3) in liver of normal birth weight rats (N), normal birth weight () rats supplemented with curcumin (NC), intra-uterine growth retardation (IUGR,
) rats (I) and IUGR rats supplemented with curcumin (IC) (12 weeks of age). B, birth weight; D, diet; B×D, interaction between the corresponding parameters. Results were corrected for total protein, n 6/group. a,b Mean values with unlike letters were significantly different when a significant interaction was observed (P<0·05).
Discussion
Globally, the number of underweight children was 113·4 million in 2015( Reference Onis, Blössner and Borghi 26 ). It has been widely accepted that early fetal life in the maternal uterus has long-term influences on offspring after birth in a variety of ways( Reference Aiken and Ozanne 27 ). Finding an effective treatment of IUGR has become one of the more important challenges for human health.
Numerous studies revealed that poor maternal nutrition was one of the main reasons for smaller offspring in animals( Reference Tarryadkins, Fernandeztwinn and Hargreaves 28 ) and humans( Reference Onis, Blössner and Borghi 26 ). In this study, rats were subjected to a 50 % food restriction from day 10 of pregnancy. A low birth weight was regarded as an important feature of IUGR( Reference Hay, Thureen and Anderson 2 ). Body weight of IUGR rats was lower than those of NBW rats from 1 d to 12 weeks of age. The results implied that the restriction of fetal nutrient intake during pregnancy delayed postnatal catch-up growth in early life( Reference Desai, Gayle and Babu 19 ). At 12 weeks of age, liver weight and its relative weight in IUGR rats were still lower than those in the NBW group, and were not improved by dietary curcumin supplementation. However, the body weights of IUGR and NBW rats slightly increased after treatment with curcumin. Early studies found that dietary administration with curcumin could alleviate the negative effects on growth caused by environmental factors( Reference Sahin, Orhan and Tuzcu 29 , Reference Zhang, Hu and Lu 30 ). In recent years, Jun et al.(31) found that dietary curcumin supplementation had no influence on the growth of rats. Further studies are necessary to investigate whether dietary curcumin supplementation has a beneficial effect on the growth of IUGR rats, especially liver weight.
IUGR neonates are associated with a high risk of inflammation and oxidative stress( Reference Tarryadkins, Fernandeztwinn and Hargreaves 28 ). TNF-α is a key immune modulator, which can activate chemotactic cytokines and produce free radicals, leading to oxidative stress( Reference Starke, Daniel and Dumont 32 ). Furthermore, TNF-α, IL-1β and IL-6 have been implicated as prototype pro-inflammatory cytokines in the pathogenesis of sepsis( Reference Deventer 33 ). Higher TNF-α, IL-1β and IL-6 levels were observed in the serum of IUGR rats, which implied that pro-inflammation occurred in the body. Reports had demonstrated that a strong pro-inflammatory bias existed in IUGR with placental insufficiency( Reference Raghupathy, Al-Azemi and Azizieh 8 ). Significantly high concentrations of TNF-α, IL-1β and IL-6 in IUGR rats were significantly decreased after dietary curcumin supplementation, suggesting that curcumin could reduce inflammatory responses in the serum. These results are similar to those of previous studies in cells( Reference Liu, Shang and Li 34 ) and rats( Reference Soetikno, Sari and Veeraveedu 35 ). The liver is an important organ, and liver dysfunction has been observed in IUGR newborn pigs( Reference Junjun, Lixiang and Defa 7 ). The activities of AST and ALT are positively correlated with portal flow( Reference Kuo, Li and Alfrey 36 ). Hepatic ALT is usually located in the cytoplasm, whereas AST is distributed in the cytoplasm and mitochondrial fractions. However, when the structure of the liver is seriously damaged, ALT and AST are released into the circulatory system, resulting in the improvement of ALT and AST activity in the serum( Reference Nyblom, Berggren and Balldin 37 ). In this study, the activities of serum AST and ALT in the IUGR group were higher than those in the NBW group. The increased activities of serum ALT and AST are generally accepted biomarkers for hepatic dysfunction( Reference Tang, Chao and Xing 38 ). The vacuolisation and cell oedema from the histological examination of liver sections were prevalent in hepatocytes of IUGR rats, which revealed histological damage in the liver of IUGR rats. Meanwhile, fat cells also had mild denaturation. Similar to our findings, IUGR fetuses had a higher activity of glutamate oxaloacetate transaminase and impaired liver function than the normal ones( Reference Liu, Lin and Wang 39 ). After curcumin supplementation, the abnormal levels of serum AST and ALT and liver histological damage improved, and these results were not different from those observed in the normal condition. Previous studies had shown that curcumin could significantly reduce the levels of serum AST and ALT( Reference Zhao, Song and Chai 40 ) and liver injury in mice( Reference Zhang, Xu and Zhang 16 ) and rats( Reference Toydemir, Kanter and Erboga 22 ). Our findings indicated that diets supplemented with curcumin attenuated liver injury and inflammation in IUGR rats. Thus, we concluded that the protective effects of curcumin on the liver may be very important in IUGR rats. To investigate the immunomodulatory mechanisms of curcumin, we detected the expressions of the NF-κB and JAK/STAT pathways in the liver. NF-κB is found in cytoplasm bound to IκB. In response to various stimuli, including cytokines, stress and bacterial pathogens, the latent cytoplasmic NF-κB/IκB complex is activated by phosphorylation( Reference Hiscott, Kwon and Genin 41 ). Phosphorylation of IκB induces their ubiquitination, proteosome degradation and, subsequently, NF-κB release and nucleus translocation( Reference Echeverri, Nancy and Mockus 42 ). NF-κB is one of the most ubiquitous eukaryotic transcription factors that regulate the expression of genes associated with control of inflammatory responses, cellular proliferation/growth, cell adhesion and other processes( Reference Chen, Castranova and Shi 43 ). Our results showed that IUGR induced increased levels of phosphorylated IκBα and nuclear NF-κB and decreased levels of cytoplasmic phosphorylated NF-κB in the liver. Similar to our study, Zhong et al.( Reference Zhong, Li and Huang 44 ) also reported that the IκB was activated and the expression of NF-κB was increased by IUGR. These results suggested that the activation of the NF-κB/IκB complex might be related to increased levels of cytokines in IUGR, including TNF-α and IL-1β. The JAK2/STAT3 pathway is known to be involved in the immune response of numerous cytokines, including IL-6, and activated in response to injury( Reference Yang, He and Hao 45 ). Our results demonstrated that IUGR induced high levels of phosphorylated JAK2 and STAT3 in the liver. The activation of the JAK/STAT3 pathway might be the result of increased IL-6 in IUGR rats. Our results also showed that expression levels of Tnfa, Il1b and Il6 were up-regulated in the liver of IUGR rats. Importantly, dietary curcumin supplementation obviously inhibited the phosphorylation levels of the NF-κB pathway and JAK2 in IUGR rats and down-regulated the expression of those downstream genes. These observations supported that curcumin attenuated inflammation through the NF-κB( Reference Soetikno, Sari and Veeraveedu 35 , Reference Surh, Chun and Cha 46 ) and JAK/STAT pathways( Reference Kim, Park and Joe 47 ) in the liver of IUGR rats, which resulted in the negative regulation of cytokines (TNF-α) and pro-inflammatory interleukins (IL-1β and IL-6)( Reference Reyes-Gordillo, Segovia and Shibayama 48 ).
Oxidative stress results from the imbalance of oxidation and antioxidation and appeared in patients with IUGR( Reference Centini, Kenanidis and Rosignoli 49 ). Overproduction of free oxygen radicals leads to lipid peroxidation and becomes one of the important reasons for cell damage. MDA is the primary product of lipid peroxidation. PC are used as markers of protein oxidation in the liver( Reference Chaudhuri, de Waal and Pierce 50 ). For the measurement of endogenous oxidative DNA damage, 8-OHDG has been used widely. In this study, we observed that the concentrations of MDA, PC and 8-OHDG in the liver of IUGR rats were significantly higher than those of NBW rats. The activities of T-AOC and SOD can validly reflect the antioxidant status of body and tissue. The IUGR group significantly decreased the activities of T-AOC and SOD in our study. GSH is one of the most important intracellular antioxidants that could eliminate lipid peroxides and repair oxidative proteins through a reaction catalysed by GPX( Reference Fang, Yang and Wu 51 ). During these reactions, GSH is converted to its disulphide form, GSSG. Under normal conditions, GSSG is reduced back to GSH by GR. GR is also an important intracellular antioxidant enzyme and can protect the cell against oxidative stress( Reference Mahmoud and Edens 52 ). Our results showed that the IUGR group significantly decreased the concentration of GSH and activities of GR and GPX and increased the concentration of GSSG and value of GSSG/GSH in the liver. Furthermore, the concentration of GSH and activity of GPX were decreased in the serum of IUGR rats. These data strongly implied that IUGR rats caused lipid, protein and DNA damage and were susceptible to oxidative stress. During severe oxidative stress, the decreased GSH concentration and increased GSSG concentration induced an increase in the GSSG/GSH cycle( Reference Jones 53 ). Lower activities of GR and GPX might be another reason for GSSG failing to reduce GSH in the liver of IUGR rats. The findings were consistent with the results reported by Zhang et al.( Reference Zhang, Chen and Li 54 ), who noted that the metabolic efficiency of the hepatic GSH redox cycle was decreased in IUGR piglets. Previous studies had proved that IUGR neonates could only weakly protect against oxidative stress( Reference Hracsko, Orvos and Novak 55 ). Curcumin is an antioxidant used to protect against oxidative stress in mice experiments( Reference Wen, Xue and Yu 56 ). However, investigations on the effect of curcumin on oxidative stress and antioxidant defence in IUGR rats are very limited. In this study, our results revealed that dietary curcumin supplementation significantly decreased the concentrations of hepatic MDA, PC and 8-OHDG and slightly increased the activities of hepatic T-AOC and SOD in IUGR rats, which were in agreement with the results of previous studies( Reference Suryanarayana, Satyanarayana and Balakrishna 57 ). Curcumin supplementation also efficiently improved the hepatic GSH redox cycle in IUGR rats. A study similar to this one was performed by Altintoprak( Reference Altıntoprak, Kar and Acar 58 ), who demonstrated that dietary curcumin supplementation had beneficial effects on the hepatic antioxidant defence capacity of IUGR rats. A report showed that curcumin could attenuate As-induced hepatic oxidative stress by activating the Nfe2l2 pathway( Reference Gao, Duan and Wang 59 ). The Nfe2l2 is a key transcription factor that regulates the transcription of some antioxidant-related genes through the Nfe2l2/antioxidant response element (ARE) pathway( Reference Kensler, Wakabayashi and Biswal 60 ). In this study, Nfe2l2 mRNA expression was down-regulated in the liver of IUGR rats. Diets supplemented with curcumin increased the hepatic Nfe2l2 mRNA expression by 27·26 %. The expression of antioxidant genes involved in the Nfe2l2/ARE pathway (Nqo1, Hmox1, Gst, Gpx1 and Sod1) were increased by curcumin supplementation in IUGR rats. The findings of this study were in agreement with previous observations that the hepatic Nfe2l2 protein and two typically recognised Nfe2l2 downstream genes, Nqo1 and Hmox1, were consistently up-regulated in curcumin-treated mice( Reference Gao, Duan and Wang 59 ). These results may indicate that curcumin protected IUGR rats from antioxidant stress by activating the expression of Nfe2l2 and downstream genes( Reference Li and Kong 61 ).
In conclusion, maternal nutrition deficiency reduced the growth of rats in utero, leading to a low birth weight and delayed catch-up growth in early life. IUGR rats exhibited a high risk of inflammation and oxidative damage in the liver. Diets supplemented with curcumin could efficiently attenuate the inflammation and hepatic injury of IUGR rats by regulating the NF-κB and JAK/STAT pathways, and enhance hepatic antioxidation capacity by activating expressions of Nfe2l2 and downstream genes. Our findings may be helpful in finding a new strategy for the early treatment of IUGR in humans in future.
Acknowledgements
The authors thank Kehu Bio-technology Research Center (Guangzhou, China) for kindly supplying the curcumin.
This study was supported by the grants from the National Natural Science Foundation of China (grant nos 31572418, 31472129 and 31601948).
The authors’ responsibilities were as follows: J. H. designed the research, conducted research, analysed data and wrote the manuscript; Y. N. conducted the research, and wrote and revised the manuscript; C. W., L. Z., X. Z. and J. Z. designed the research; F. W., T. C. and K. B. conducted the research; and T. W. had primary responsibility for final content. All authors read and approved the final manuscript.
The authors declare that there are no conflicts of interest.