The consumption of citrus fruits might be involved in the prevention of non-communicable diseases such as cancers and cardiovascular diseases (CVD) (Kurowska et al. Reference Kurowska, Spence, Jordan, Wetmore, Freeman, Piche and Serratore2000; Yano, Reference Yano2002; Aviram et al. Reference Aviram, Rosenblat, Gaitini, Nitecki, Hoffman, Dornfeld, Volkova, Presser, Attias, Liker and Hayek2004). Citrus juices, such as orange and mandarin, are consumed all over the world and are one of the main dietary sources of β-cryptoxanthin (β-CX) (Lin & Chen, Reference Lin and Chen1995; Mouly et al. Reference Mouly, Gaydou, Lapierre and Corsetti1999; Kiong-Cheol et al. Reference Kiong-Cheol, Chan-Shick, Nam Ho, Sam-Pin and Doo-Khil2000; Goodner et al. Reference Goodner, Rouseff and Hofsommer2001; Lee & Castle, Reference Lee and Castle2001). β-CX is the main precursor of vitamin A in citrus juices (Dhuique-Mayer et al. Reference Dhuique-Mayer, Caris-Veyrat, Ollitrault, Curk and Amiot2005). This carotenoid is not fully cleaved in vitamin A in the gut and it is therefore recovered in human plasma (Yeum et al. Reference Yeum, Booth, Sadowski, Liu, Tang, Krinsky and Russell1996). Recent clinical studies indicated that the frequent intake of citrus fruits increases plasma β-CX concentrations and reported β-CX as a biomarker of mandarin consumption (Sugiura et al. Reference Sugiura, Kato, Matsumoto, Nagao and Yano2002; Irwig et al. Reference Irwig, El-Sohemy, Baylin, Rifai and Campos2002). In vivo and in vitro experiments suggest that β-CX might prevent certain cancers (Narisawa et al. Reference Narisawa, Fukaura, Oshima, Inakuma, Yano and Nishino1999; Tanaka et al. Reference Tanaka, Kohno, Murakami, Shimada, Kagami, Sumida, Azuma and Ogawa2000; Yano, Reference Yano2002). However, the biological activities of β-CX greatly depend on its bioavailability.
Carotenoid bioavailability is usually assessed by following changes in plasma carotenoid concentrations after the ingestion of a carotenoid-rich meal (Edwards et al. Reference Edwards, Vinyard, Wiley, Brown, Collins, Perkin-Veazie, Baker and Clevidence2002; Tyssandier et al. Reference Tyssandier, Cardinault, Caris Veyrat, Amiot, Grolier, Bouteloup, Azais Braesco and Borel2002, Reference Tyssandier, Feillet-Coudray, Caris-Veyrat, Guilland, Coudray, Bureau, Reich, Amiot-Carlin, Bouteloup-Demange, Boirie and Borel2004). However, the wide variations in plasma carotenoid response between individuals and the costs of these studies limit the number possible (Castenmiller & West, Reference Castenmiller and West1998). Some animal models are available, but no model appears ideal and again costs limit their use (Lee et al. Reference Lee, Boileau, Boileau, Williams, Swanson, Heintz and Erdman1999). Recently an in vitro model was developped giving promising results (Garrett et al. Reference Garrett, Failla and Sarama1999a; Hedren et al. Reference Hedren, Diaz and Svanberg2002; Chang-shu et al. Reference Chang-shu, Glahn and Hai Liu2004). This model first simulates gastric and duodenal processes and then evaluates the micellarisation of carotenoids from a test meal. The quantity of micellarised carotenoids is used as an estimate of carotenoid bioaccessibility (the bioaccessibility of a fat-soluble nutrient is the proportion of this micronutrient extracted from the food matrix and solubilised in mixed micelles). Then, Caco-2 cells are used as a model of the intestine in order to measure carotenoid uptake (During et al. Reference During, Hussain, Morel and Harrison2002).
The objective of this study was to assess β-CX bioaccessibilty by using an in vitro digestion model coupled to Caco-2 (TC-7 clone) intestinal cells. In this study, special attention was given to the behaviour of esterified β-CX forms compared with its free form.
Materials and methods
Chemicals
Extraction solvents were RPE-grade hexane, ethanol and dichloromethane from Carlo-Erba (Val de Reuil, France). Analytic solvents were HPLC-grade methanol, acetonitrile and tetrahydrofuran (THF) also from Carlo-Erba (Val de Reuil, France), and methyl-tert-butyl-ether (MTBE) from Sigma-Aldrich (Steinheim, Germany). Carotenoid standards (98 % pure) used for HPLC analysis were purchased from Extrasynthèse (Genay, France): β-carotene, β-CX, zeaxanthin, lutein, lycopene and β-apo-8′-carotenal. Dulbecco's modified Eagle's medium (DMEM) containing 4·5 g/l glucose and trypsin-EDTA (500 and 200 mg/l, respectively) was purchased from Bio Whittaker (Fontenay-sous-Bois, France). Fetal bovine serum (FBS) was purchased from Biomedia (Issy-les-Moulineaux, France), and NEAA and penicillin/streptomycin were from Gibco BRL (Cergy-Pontoise, France). Pepsin, porcine pancreatin, porcine bile extract and pyrogallol were purchased from Sigma-Aldrich (St Quentin Fallavier, France).
Citrus fruits and juices
Different varieties and species of Citrus were chosen from Agronomic Research Station (SRA) selections in Corsica: orange cv. Shamouti (Sh) (Citrus sinensis (L.) Osbeck), mandarin cv. Wilowleaf (W) (Citrus deliciosa Ten), mandarin cv. Hansen (H) (Citrus reticulata blanco) and lemon cv. Meyer (Citrus meyeri Yu. Tanaka) following the Tanaka classification. SRA numbers were: cv. Shamouti SRA 538; cv. mandarin M SRA 133; cv. mandarin Hansen SRA 356; cv. Meyer lemon SRA 549. The representative sample size (15 fruits/cultivar) was determined in a previous study (Dhuique-Mayer et al. Reference Dhuique-Mayer, Caris-Veyrat, Ollitrault, Curk and Amiot2005). Citrus fruits received at maturity (maturity index: soluble solids/titrable acidity = 7) were immediately hand-squeezed, then filtered through a stainless steel sieve (1 mm). The juices were placed in sealed amber vials (15 ml) under nitrogen and kept frozen at − 20°C until analysed; storage time did not exceed 1 month.
Extraction and HPLC analysis of carotenoids from native juices
Extraction, saponification and analysis were carried out according to our previous study (Dhuique-Mayer et al. Reference Dhuique-Mayer, Caris-Veyrat, Ollitrault, Curk and Amiot2005). Citrus juice were extracted with ethanol–hexane (4:3, v/v). Saponification with 10 % methanolic KOH was carried out overnight in the dark at room temperature. HPLC analyses were performed with an Agilent 1100 System. Carotenoids were separated on a C30 column (250 × 4·6 mm id, 5 μm YMC (EUROP GmbH, Germany). The mobile phases were H2O as eluent A, methanol as eluent B, and MTBE as eluent C. The flow rate was fixed at 1 ml/min, the column temperature was set at 25°C, and the injection volume was 20 μl. A gradient programme was performed: the initial condition was 40 % A/60 % B, 0–5 min; 20 % A/80 % B, 5–10 min; 4 % A/81 % B/15 % C, 10–60 min; 4 % A/11 % B/85 % C, 60–71 min; 100 % B 71–72 min; and back to the initial conditions for re-equilibration. Absorbance was followed using an Agilent 1100 photodiode array detector. Quantification of carotenoids was achieved using calibration curves with β-carotene and β-CX at 450 nm.
Carotenoid extraction from digested juices
A 10 ml aliquot of filtered digested juices (and 10 ml of non-filtered digested juices) was extracted three times with 10 ml of hexane (with 0·1 % butylated hydroxytoluene) and 5 ml of ethanol containing 100 μl of recovery standard (100 μl of lycopene solution, equivalent to 12 μg). Without saponification, the hexanic extracts were evaporated and redissolved in 500 μl of mobile phase (250 μl of dichloromethane and 250 μl of an 80:20 (v/v) mixture of MTBE and methanol. Samples were injected under the analytical conditions described above. With saponification, the hexanic extracts were evaporated and redissolved in 4 ml of hexane and placed in a 15 ml amber vial to which were added 4 ml of 10 % methanolic KOH. Saponification was carried out overnight in the dark at room temperature. The sample was shaken under nitrogen in the sealed vial. The hexanic layer was separated from the aqueous phase with 8 ml of water. The methanolic KOH layer was extracted three times with 10 ml of hexane and 5 ml of ethanol. The extracts were pooled and washed to remove alkali. The pooled extracts were evaporated, dried and redissolved as described above for extraction without saponification.
Isolation of β-cryptoxanthin esters (β-CXE)
β-CXE from mandarin juice extracts were purified by HPLC; the retention times of the respective β-CXE were laurate, 44 min and myristate, 46 min. The identification of β-CXE was previously carried out by liquid chromatography–mass spectrometry (LC-MS) in positive electrospray mode according to the conditions established by Caris-Veyrat et al. (Reference Caris-Veyrat, Schmid, Carail and Böhm2003). The collected fractions of β-CXE were combined and evaporated to dryness, and the residue redissolved in 1 ml of mobile phase (500 μl of dichloromethane and 500 μl of an 80:20 (v/v) mixture of MTBE and methanol). Quantification of β-CXE was achieved using the calibration curves of β-CX and expressed as β-CX equivalent (mg/l).
HPLC analysis of carotenoids from Caco-2 cells
A 500 μl aliquot of scraped cells in PBS was extracted twice with 2 ml of hexane and 500 μl of ethanol containing internal standard (cantaxanthin 2 mg/l). The hexanic phases obtained after centrifugation (2000 rpm, 5 min, room temperature) were evaporated to dryness under nitrogen; the residue was redissolved in 100 μl of dichloromethane–methanol 50:50 (v/v). Carotenoids were separated on a C30 column (250 × 4·6 mm id, 5 μm YMC (EUROP GmbH, Germany) by HPLC as described above.
In vitro digestion
The in vitro digestion system was similar to that previously described by Garett et al. (1999a) with modifications. A volume of 30 ml of juice (or 20 g of concentrate juice at 60° brix) was placed in a 100 ml amber bottle and mixed with 32 ml of 0·9 % NaCl containing 12·6 mg/ml pyrogallol as amphiphilic antioxidant. This mixture was homogenized for 10 min at 37°C in a shaking water bath. To mimic the gastric step, the pH was adjusted to 4·00 (sd 0·02) with about 2 ml of 1 m-NaOH, then 2 ml of porcine pepsin (40 mg/ml in 0·1 m-HCl) were added. The homogenate was incubated at 37°C in a shaking water bath for 30 min. To mimic the intestinal step, the pH of the partially digested mixture was raised to 6·00 (sd 0·02) by adding around 20 ml of 0·45 m-sodium bicarbonate pH 6·0. Then a mixture of porcine bile extract and pancreatin (9 ml containing 2 mg/ml pancreatin and 12 mg/ml bile extract in 100 mmol/l trisodium citrate, pH 6·0) and 4 ml of bile extract at 0·1 g/ml were added. Samples were incubated in a shaking water bath at 37°C for 30 min to complete the digestion process. (According to Reboul et al. (Reference Reboul, Richelle, Perrot, Desmoulins-Malezet, Pirisi and Borel2006b), the duration of incubation in duodenal conditions was set at 30 min instead of 2 h in order to mimic the digestive transit time of a food particle in the human duodenum.) Micelles were separated by ultracentrifugation (20 000 rpm for 18 h at 10°C using a Beckman L755 TST 41-14 SW rotor). The aqueous fraction was collected from the centrifuge tube using a needle attached to a 10 ml syringe. It was then filtered through a 0·22 μm filter (Millipore). Aliquots were stored at − 20°C under nitrogen until analysis.
Synthetic micelles of carotenoid from citrus juice extract
β-CX from citrus juice extract was delivered to Caco-2 cells incorporated in mixed micelles that were prepared as reported by Reboul et al. (Reference Reboul, Abou, Mikail, Ghiringhelli, Andre, Portugal, Jourdheuil-Rahmani, Amiot, Lairon and Borel2005). Stock solutions of phosphatidylcholine, lysophosphatidylcholine, monoolein, free cholesterol and oleic acid were prepared in chloroform–methanol (2:1, v/v). Appropriate volumes of the following compounds were transferred into glass bottles to obtain the final concentrations: 0·04 mm-phosphatidylcholine, 0·16 mm-lysophosphatidylcholine, 0·3 mm-monoolein, 0·1 mm-free cholesterol, 0·5 mm-oleic acid and 30 μm-citrus juice extract. Stock solution solvents were carefully evaporated under nitrogen. Dried residue was solubilized in DMEM containing 5 mm-taurocholate and vigorously mixed by sonication (Branson 250 W sonifier, Osi) for 3 min. The mixture obtained was sterilised by filtering it through a pre-sterilised 0·22 μm filter (Millipore), and the resulting solutions were optically clear.
Measurement of carotenoid absorption by intestinal cells
Caco-2, clone TC-7 cells were a gift from Dr Monique Roussey (U178 INSERM, Villejuif, France). Cells, passage 70–80, were grown in 25 cm2 flasks (TTP, Switzerland) in the presence of DMEM supplemented with 20 % heat-inactivated FBS, 1 % NEAA and 1 % antibiotics (complete medium). Cells were incubated at 37°C in a humidified atmosphere of air–carbon dioxide (95:5, v/v) and the medium changed every 48 h. Monolayers were subcultured with a 7 d passage frequency when they reached a confluence of about 80 % by treatment with 0·25 % trypsin-EDTA. For each experiment, cells were seeded at a density of 250 000 cells/well, and grown on transwells (6-well plate, 24 mm diameter, 1 μm pore size polycarbonate membrane; Becton Dickinson, le Pont-de-Chaix, France). The medium used in apical and basolateral chambers was the complete medium. Media were changed every day for 21 d to obtain confluent, differentiated cell monolayers (Salvini et al. Reference Salvini, Charbonnier, Defoort, Alquier and Lairon2002). One day before each experiment, the medium used in apical and basolateral chambers was DMEM supplemented with 20 % heat-inactivated lipid-free FBS, 1 % NEAA and 1 % antibiotics. Before each experiment and after 150 min of incubation, cellular integrity was checked by measuring trans-epithelial electrical resistance with a voltohmmeter equipped with a chopstick electrode (Millicell ERS, Millipore, Saint-Quentin-en-Yvelines, France). In order to measure carotenoid absorption by Caco-2 cells, carotenoid-rich micelles coming from the in vitro digestion were used at 1:3 dilution. At the beginning of each experiment, cell monolayers were washed twice with 1 ml of PBS in the apical side and 2 ml in the basolateral side. The apical side of the cell monolayers received 1 ml of micelles, while the basolateral side received 2 ml of FBS-free medium. Cell monolayers were incubated at 37°C for 30, 60 and 150 min. The short times used in our experiments represent approximately, the time during which the bowel content is in contact with duodenal cells during digestion. After the incubation period, media from each side of the membrane were harvested. Cell monolayers were washed twice with 1 ml of PBS containing 5 mmol/l taurocholate to eliminate adsorbed carotenoids, scraped and collected in 1 ml of PBS. Absorbed carotenoids were estimated as carotenoids both in scraped cells and in the basolateral chambers of the transwells. All the samples were stored at − 80°C under nitrogen with 0·5 % pyrogallol as a protective antioxidant before carotenoid extraction and HPLC analysis.
Statistical analysis of data
All statistical analyses were performed using XLSTAT software version 7·0. All data were reported as mean (and sd) from three replicates of each experiment. For the in vitro digestion experiment, samples were duplicated (n 6) Differences between means were tested using non-parametric Kruskal–Wallis and Mann–Whitney tests. Means were considered to be significantly different if P values were < 0·05.
Results
β-Cryptoxanthin changes in digested citrus juices
Both free β-CX and β-CXE were present in citrus fruits (mainly β-CX laurate and β-CX myristate) (Table 1). The percentage of the free form for orange and mandarins varied from 5 to 18 %. In contrast, Meyer lemon was characterized by its high content of free β-CX, which accounted for 75 % of the total. In orange and mandarin juices, the main ester was myristate, which accounted for >50 % of total β-CX. Submitting juices to in vitro digestion significantly increased the percentage of the free form of β-CX 3·5- to 5-fold in orange and mandarins, but only 1·1-fold in Meyer lemon.
Table 1 Free and esterified β-cryptoxanthin (mg/200 ml) in native and digested citrus juices (200 ml is the volume of a serving portion of fruit juice) (Mean values of three determinations and standard deviation)

The mean of three determinations is shown.
Values in parentheses indicate the ratio of each β-cryptoxanthin species to the total β-cryptoxanthin.
β-CX, β-cryptoxanthin; β-CXL, β-cryptoxanthin laurate; β-CXM, β-cryptoxanthin myristate.
* Extract made from centrifugated digested juice.
† A significant difference (P < 0·05) between the percentage of free β-cryptoxanthin in fresh juice and the corresponding percentage after in vitro digestion.
Bioaccessibility of carotenoids from citrus juices
Centrifugation and filtration of digested juices yields the micellar fraction used to estimate bioaccessibility. The in vitro digestion model estimates the proportion of carotenoids micellarised. Bioaccessibility of total β-CX (free plus esterified forms) and β-carotene from citrus juices is shown in Fig. 1. Mandarin H, mandarin W and orange Sh displayed similar β-CX bioaccessibilities (16, 18 and 22 % respectively, P>0·05), whereas for Meyer lemon it was significantly higher (40 %, P < 0·05). Among the four species of citrus, mandarin H had the highest concentration of total β-CX (2·18 mg/l) in the micelle fraction, followed by mandarin W (1·26 mg/l), Meyer lemon (0·63 mg/l) and orange Sh (0·44 mg/l). Regarding the bioaccessibility of β-carotene (Fig. 1B), the percentage transferred into the micellar fraction did not differ significantly (P>0·05) between the different citrus juices. The percentages of accessible β-carotene were 26, 31, 33 and 30, respectively, for mandarin M, mandarin H, orange Sh and Meyer lemon. The percentages of bioaccessible β-carotene were higher than those of β-CX for all citrus juices except Meyer lemon. Thus, the bioaccessibility of β-CX in Meyer lemon (40 %) significantly exceeded (P < 0·05) that of β-carotene (30 %). Concerning the concentration of β-carotene determined in the micelle fraction, mandarin H and mandarin W displayed the highest values with 0·74 and 0·41 mg/l, respectively, whereas Meyer lemon and orange Sh displayed the lowest values (0·07 mg/l for both). Similar results for carotenoid micellarisation were obtained with synthetic micelles enriched in carotenoids purified from citrus juices (Table 2). The percentage micellarisation of free β-CX was about three times higher (27 %) than that of β-carotene (10 %) and its esters (9 %). The micellarisation of the β-CXE, β-CX laurate, was 2·5 times higher (34 %) than that of the other form, β-CX myristate (13·7 %).
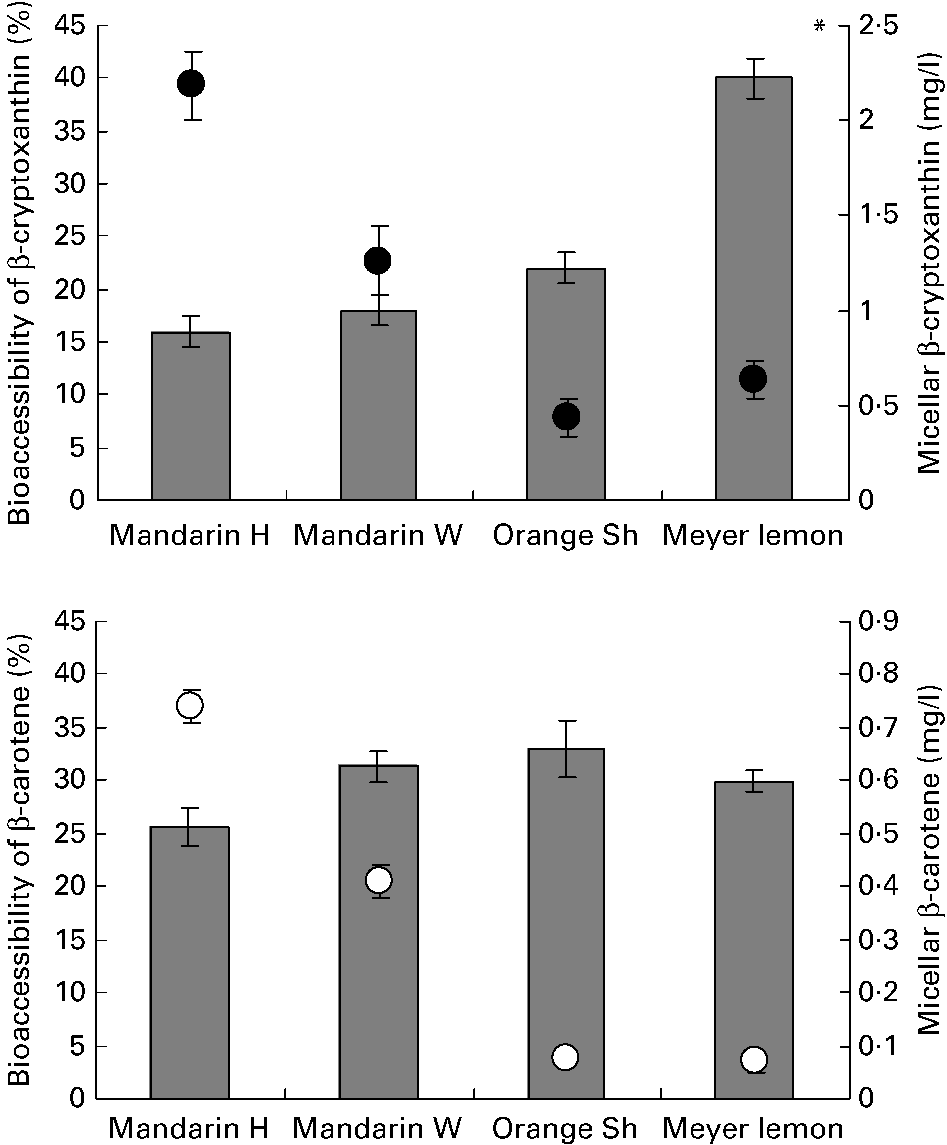
Fig. 1 (A) Bioaccessibility of total β-cryptoxanthin (free plus ester form) in different citrus juices. Bioaccessibility is expressed as the percentage of micellar total β-cryptoxanthin (left axis). ●, concentration of micellar total β-cryptoxanthin in the micelle fraction of different citrus juices (right axis). (B) β-Carotene data. ○, concentration of micellar total β-carotene in the micelle fraction of different citrus juices (right axis). Data are the mean and sd of three experiments with duplicate samples for each experiment (n 6). An asterisk indicates a significant difference (P < 0·05) between bars of the same figure.
Table 2 Carotenoid micellarisation in synthetic micelles from carotenoid citrus extracts (Mean values and standard deviation)

β-CX, β-cryptoxanthin; β-CXL, β-cryptoxanthin laurate; β-CXM, β-cryptoxanthin myristate.
* Mean of three determinations (%).
Uptake of carotenoids by Caco-2 cells exposed to either synthetic micelles or physiological micelles
Synthetic micelles prepared from a mixture of lemon and mandarin carotenoid extract were delivered to Caco-2 cell monolayers (the juices were mixed in a ratio to obtain final percentages of β-CX and β-carotene). Carotenoid uptake by Caco-2 monolayers at 150 min was estimated (Table 3). Cellular uptake was expressed as a percentage of absorbed carotenoids recovered in the scraped cells. The uptake by cells was similar for β-CX and β-carotene (19·0 (sd 0·6) % and 20·6 (sd) 1·2 %). The two esters, β-CX laurate and β-CX myristate, appeared to be less efficiently absorbed (14·4 (sd 0·7) %) in spite of their high contents in micelles. Nevertheless althought percentage uptakes were lower than for β-carotene and β-CX, the two esters represented >60 % of the total carotenoids in cells. To complete this study, purified β-CXE from carotenoid mandarin extracts were micellarised. In these synthetic micelles containing only β-CX laurate and β-CX myristate, the uptake by cells was similar for the two esters (16 (sd 0·3) %). No trace of free β-CX was found in cells or the basolateral chamber after uptake of the two esters. Note that in this experiment, no carotenoids were found in the basolateral chamber although the preparation of synthetic micelles requires some compounds, such as oleic acid, which are capable of stimulating the synthesis and secretion of chylomicrons in Caco-2 cells (Luchoomum & Hussain, Reference Luchoomum and Hussain1999).
Table 3 Carotenoid distribution in micelles and Caco-2 cells and percentage of carotenoid uptake by cells exposed to synthetic or physiological micelles

No carotenoids were found in the basolateral chamber.
β-CX, β-cryptoxanthin; β-CXL, β-cryptoxanthin laurate; β-CXM, β-cryptoxanthin myristate.
* Carotenoid composition in micelles and in cells.
† Percentage of carotenoids in cell+basolateral chamber/carotenoids in apical chamber.
The intestinal uptake of citrus juice carotenoids was also assessed by using physiological micelles produced during the in vitro digestion of citrus juices. As reported in Table 3, after 150 min of incubation, the four carotenoids present in citrus juice after digestion were absorbed by Caco-2 cells. There were large differences in uptake among the carotenoids, the uptake by cells being inversely proportional to the initial carotenoid concentration present in the micelle digestate. Carotenoid distributions in cells were 64·6 % (β-carotene), 19·5 % (β-CX), 8·3 % (β-CX laurate) and 7·6 % β-CX myristate). β-Carotene was the carotenoid that accumulated most (14·3 (sd 1·8) %) in the cells, followed by free β-CX (3·9 (sd 0·9) %). Uptake by cells of the two esters was very low: 0·7 (sd 0·1) % for myristate and 0·6 (sd 0·1) % for laurate.
Discussion
Because esters of xanthophylls such as β-CXE are widely distributed in nature in several fruits and vegetables and particularly in citrus (Breithaupt & Bamedi, Reference Breithaupt and Bamedi2001), our study focused on the bioaccessibility of these esters present in citrus juices at different concentrations. The Caco-2 human intestinal cell line was chosen as a model for assessing the intestinal uptake of carotenoids (Garrett et al. Reference Garrett, Failla and Sarama1999Reference Garrett, Failla, Sarama and Craftb; Ferruzzi et al. Reference Ferruzzi, Failla and Schwartz2001; During et al. Reference During, Hussain, Morel and Harrison2002). In our study, we conducted experiments to assess the bioaccessibility of carotenoids in citrus juices using a Caco-2 cell culture model coupled with an in vitro digestion process.
The first step was to evaluate the bioaccessibility of carotenoids using an in vitro digestion model. Due to its high ratio of free to ester form of β-CX, Meyer lemon was used to evaluate the bioaccessibility of free β-CX, whereas mandarin and orange were used to study the bioaccessibility of β-CXE. We showed that after submitting citrus juices to in vitro digestion conditions, the bioaccessibility of free β-CX exceeded that of β-carotene and β-CXE. These data suggested that the main factor involved in bioaccessibility was the micellarisation capacity of carotenoids. Previous studies (Garrett et al. Reference Garrett, Failla and Sarama1999a; Chitchumroonchokchai et al. Reference Chitchumroonchokchai, Schwartz and Failla2004) have reported a preferential micellarisation of xanthophylls such as lutein compared with β-carotene submitted to in vitro digestion conditions. In the present study, the behaviours of free β-CX and ester forms were in agreement with previous data. When preparing synthetic micelles with carotenoids purified from citrus juice extracts, we showed that free β-CX was 3-fold more micellarised than β-carotene and the ester forms of β-CX. In a previous study, Borel et al. (Reference Borel, Grolier, Armand, Partier, Lafont, Lairon and Azais Braesco1996) reported that more apolar carotenoids are preferentially located in the triacylglycerol core of lipid droplets, while more polar carotenoids such as lutein reside near the surface. According to some authors (Cooper, Reference Cooper, Webb and Peters1997; Tyssandier et al. Reference Tyssandier, Borel, Choubert, Grolier, Alexandre-Gouabau and Azais-Braesco1998), carotenoids are highly lipophilic compounds characterized by a high octanol partition coefficient (log10P>8); they reported that the bioavailability of carotenoids is related to their polarity.
The increase in the proportion of free β-CX in micelles after submitting citrus juices to in vitro digestion conditions suggests that β-CXE were partially hydrolysed under these conditions. The percentage of hydrolysis was shown to depend on the type of citrus juice. The percentages of free β-CX for mandarin and orange juices were around 3–4·5 times higher than in native juices. Several data from human studies suggest that β-CXE are hydrolysed in the intestinal lumen prior to their absorption (Wingerath et al. Reference Wingerath, Sies and Stahl1998; Breithaupt & Bamedi, Reference Breithaupt and Bamedi2001; Breithaupt & Bamedi, Reference Breithaupt and Bamedi2002). More recently, Breithaupt et al. (Reference Breithaupt, Weller, Wolters and Hahn2003) have confirmed that only free β-CX was detected in plasma after ingestion of β-CXE from a vegetable source. The enzyme involved in hydrolysis is probably a carboxyl ester hydrolase able to hydrolyse xanthophyll esters (Breithaupt & Bamedi, Reference Breithaupt and Bamedi2002). Our study suggests that the esters could be cleaved in the small intestine by pancreatic lipase before absorption by intestinal cells. β-CXE are hydrolysed during in vitro digestion probably by an enzyme present in pancreatin in the intestinal digestion step. We suppose that this enzyme involved in the hydrolysis of esters could be the pancreatic lipase. Lindstrom et al. (Reference Lindstrom, Sternby and Borgstrom1988) demonstrates that this enzyme can hydrolyse the same type of substrate as carboxyl ester hydrolase, i.e. cholesteryl oleate or retinyl palmitate in the presence of bile salt in physiological conditions. It would be interesting to study the role of lipases in the hydrolysis of xanthophylls esters. In fact, it was recently shown that classical pancreatic lipase and pancreatic lipase-related proteins 2 were able to hydrolyse retinyl palmitate in the duodenum, which is the site of pancreatic and biliary secretions (Reboul et al. Reference Reboul, Berton, Moussa, Kreuzer, Crenon and Borel2006a).
The second step was to evaluate the intestinal uptake of carotenoids by Caco-2 cells. Carotenoid accumulation by Caco-2 cells was in inverse proportion to carotenoid content in the micelles, such that the percentages of β-CXE taken up by cells were dramatically reduced. In our study, the preferential cellular uptake of β-carotene compared with β-CXE was remarkable as it is known that the β-carotene concentration in the micelles was lower than that of β-CXE. When we conducted experiments with synthetic micelles produced from citrus juice extracts, we observed large differences in the uptake of carotenoids by cells exposed to the synthetic micelles compared with micelles generated during in vitro digestion. In synthetic micelles produced from lemon+mandarin extract, the cellular accumulation of carotenoids was proportional to the content of micellarised carotenoids in the medium. Although the results obtained with synthetic micelles confirmed a similar preferential uptake of β-carotene and free β-CX, the two ester forms appeared more absorbed by cells from the synthetic micelles than those from from the physiological micelles. Chitchumroonchokchai et al. (Reference Chitchumroonchokchai, Schwartz and Failla2004) also observed a difference between physiological and synthetic micelles, suggesting that the phospholipid composition of synthetic micelles was a factor in enhancing the carotenoid uptake by cells (Sugawara et al. Reference Sugawara, Kushiro, Zhang, Nara, Ono and Nagao2001). Differences in the molecular composition of the micelles may contribute to this discrepancy, while particle size and surface charge may affect the ability to interact with the brush border surface (Borel et al. Reference Borel, Grolier, Armand, Partier, Lafont, Lairon and Azais Braesco1996).
Our study underlined that β-carotene seems to be absorbed to a similar extent from all types of micelles. Previous studies reported the preferential uptake of β-carotene compared with lutein (Garrett et al. Reference Garrett, Failla and Sarama1999Reference Garrett, Failla, Sarama and Craftb, Reference Garrett, Failla and Sarama2000; During et al. Reference During, Hussain, Morel and Harrison2002; Chang-Shu et al. Reference Chang-shu, Glahn and Hai Liu2004). In these previous studies, carotenoids were in emulsified forms. Borel et al. (Reference Borel, Grolier, Armand, Partier, Lafont, Lairon and Azais Braesco1996) suggested that the emulsified form of β-carotene was more efficiently taken up than a more polar carotenoid due to the stability of the emulsified compound. Our results supported previous findings suggesting that the transfer of β-carotene across the brush border was more efficient than that of other carotenoids (Garrett et al. Reference Garrett, Failla and Sarama2000).
Finally, in the present investigation, it should be stressed that esters were taken up by intestinal epithelial cells. Chitchuroonchokchai & Failla (Reference Chitchuroonchokchai and Failla2006) also reported the presence of zeaxanthin monoester in cells, but they indicated that free zeaxanthin was taken up by Caco-2 cells more efficiently than zeaxanthin esters. On the other hand, the fact that after incubation of purified esters on Caco-2 cells no trace of free β-CX was observed in cells or the basolateral chamber suggests that β-CXE were not cleaved by intestinal cells. These in vitro data are consistent with the in vivo data reporting a higher plasma response to the free form of β-CX. According to Breithaupt et al. (Reference Breithaupt, Weller, Wolters and Hahn2003), β-CXE were not recovered in plasma but the bioavailabilities of free and β-CXE were comparable.
However, the fact that Caco-2 cells seemed to absorb β-CXE to a lesser extent than the free form corroborated previous studies where the presence of xanthophyll esters was reported in the plasma of humans administered high doses (Bowen et al. Reference Bowen, Herbst-Espinosa, Hussain and Stacewicz-Sapuntzakis2002) of lutein for extended periods (Granado et al. Reference Granado, Olmedilla, Gil-Martinez and Blanco1998) and in human skin (Wingerath et al. Reference Wingerath, Sies and Stahl1998).
In conclusion, the present study provides the first assessment of β-CX (free and esters) uptake by differentiated Caco-2 cells. The simulated digestion coupled with the Caco-2 cell model appeared to be an efficient tool for studying the uptake of dietary carotenoids. Using this model, although the bioaccessibility of β-CXE was relatively high, the esters were poorly absorbed. When citrus carotenoids were incorporated into synthetic micelles, we showed that the absorption of esters could be enhanced by their vectorisation with phospolipids. Nevertheless, β-CXE appeared to be hydrolysed during the digestion phase, resulting in an apparent enhancement of the bioavailability of the free form of β-CX. Although our results suggest that the Caco-2 cells (TC7 clone) were not able to cleave β-CXE, further investigations are needed to specify the metabolism of esters within the enterocytes as well as the possible cleavage of β-CX into vitamin A.
Acknowledgements
The authors thank the Agronomic Research Station (SRA) Inra of San Guiliano (Corsica) for the kind gift of citrus fruits. We also thank Catherine Caris-Veyrat for her help in identification of β-CXE by LC-MS, and Guy Self for assistance in English text corrections.