With its enriched nutritional value and balanced protein content, pork has become a prevalent type of meat worldwide, and it serves as a vital source of essential protein in human diets(Reference Buła, Przybylski and Jaworska1,Reference Xu, Chen and Huang2) . The key indicators of pork quality are flavour, juiciness, tenderness and nutritional value. Muscle fibre characteristics are closely associated with tenderness, meat colour, water-holding capacity and postmortem redox characteristics(Reference Bai, Yin and Ru3,Reference Kim, Jeong and Jung4) . Besides the composition of amino acids and fatty acids, lipo-nutritional quality (determined by lipid profiles) is a crucial determinant of pork nutritional value and is intricately linked to human health(Reference Wang, Zhang and Huang5,Reference Yi, Huang and Wang6) . For example, increased dietary cholesterol intake during pregnancy was associated with greater risk of gestational diabetes mellitus(Reference Wu, Sun and Zhou7). A recent study has demonstrated that DHA-enriched phosphatidylcholine (PC) alleviates high-fat diet-induced non-alcoholic fatty liver disease by regulating lipid metabolism and dysbiosis via the gut–liver axis(Reference Qian, Tian and Jiang8). With the development of lipidomics, lipids can be classified into fatty acids, glycerolipids, glycerophospholipids, sphingolipids, sterol lipids and prenol lipids, saccharolipids and polyketides(Reference Fahy, Subramaniam and Brown9). In addition to storing energy, lipids are also important components of cell membranes and play a key role in signal transduction during cell development(Reference Yoon, Shaw and Haigis10). Additionally, lipid oxidation is a major contributor to the deterioration of meat flavour and nutritional value(Reference Rossi, Pastorelli and Cannata11). Muscle antioxidant capacity is closely related to pork preservation and flavour(Reference Shakoor, Zhang and Xie12). The excessive pursuit of feeding efficiency and lean percentage in the pig industry has led to a decline in pork quality(Reference Xu, Chen and Huang2). Therefore, it is imperative to improve pork quality without compromising on production efficiency.
Numerous studies have confirmed that supplementing some feed additives such as vitamin E(Reference Jin, Gao and Wang13), conjugated linoleic acid(Reference Wang, Zhang and Huang5) and proanthocyanidin(Reference Xu, Chen and Huang2) can improve intramuscular fat, meat colour, flavour and tenderness while altering the composition of fatty acid and amino acids. Fermented feed (FF) refers to a product obtained by fermenting a combination of plant materials with bacteria or fungi. Compared with adding feed additives, microbial fermentation can improve the nutritional value of feed ingredients, such as increasing the content of amino acids and fatty acids, while decreasing the content of β-conglycinin and glycinin(Reference Hao, Su and Zhang14,Reference Liu, Du and Tu15) . Previous research has shown that FF can improve growth performance and reduce the diarrhoea rate in animal production(Reference Ding, Li and Wen16,Reference Wang, Lin and Su17) . Additionally, FF has demonstrated promising results for improving pork quality and antioxidant capacity in finishing pigs(Reference Hao, Su and Zhang14). However, the effects of FF on muscle fibre type and lipo-nutritional quality remain unclear.
Therefore, the purpose of this study was to investigate the impact of FF on muscle fibre characteristics, lipo-nutritional quality and antioxidant capacity in finishing pigs. We hypothesise that FF could regulate muscle fibre characteristics, alter muscle lipid profiles and enhance antioxidant capacity in finishing pigs. This investigation would provide crucial theoretical evidence for utilising FF to enhance pork quality.
Materials and methods
Animals used in this study was approved by the Institutional Animal Care and Use Committee at Zhejiang University (Hangzhou, China, ZJU20170466) and animal trial carried out based on the guidelines for the Care and Use of Laboratory Animals.
Experimental design and diets
A total of 144 Duroc × Berkshire × Jiaxing Black finishing pigs (weighing approximately 80 kg) were randomly assigned into three groups with four replicates (twelve pigs per replication). Each group consisted of half males and half females. The three treatments were a basal diet (0 % FF), basal diet + 5 % FF and basal diet + 10 % FF, respectively. We used Bacillus subtilis and Enterococcus faecalis for co-fermentation. The B. subtilis ZJU12 used in this study was isolated from pickled vegetables. E. faecium was purchased from Baolai-leelai Biotech Co., Ltd. The basal substrate contained 40 % maize, 40 % soyabean meal and 20 % wheat bran. The contents of glycinin, β-conglycinin, trypsin inhibitor and globulin in feed were analysed using the ELISA kits (Longkefangzhou Bio Co.) according to the manufacturers’ introductions. The diets were formulated to ensure similar levels of digestible energy and crude protein in the three experimental diets to meet the nutritional requirements of finishing pigs according to the Chinese National Feeding Standard. The ingredients and nutrient levels of the experimental diets were the same as those used in our previous research(Reference Liu, Du and Tu15). All pigs were fed three times (at 07.30, 13.30 and 17.30) per day and given free access to water and food throughout a 38-d period, including 4 d of pre-feeding.
Sample collection
At the end of the study, eighteen pigs (six barrows per group) were randomly selected and fasted for 12 h with access to water before slaughter. The procedures of slaughter and sample collection were performed in a commercial slaughterhouse (Qinglian Food Co., Ltd.). After electrical stunning, the pigs were slaughtered humanely. Blood was collected through the anterior vena cava, centrifuged at 3000 r/min for 10 min and, subsequently, serum was extracted from the supernatant. The serum samples were stored at –80°C for enzyme activity analysis. Within 20 min of slaughter, the longissimus thoracis samples were collected, frozen in liquid N2 and then stored at –80°C for enzyme activity, gene and protein expression analysis. At the same time, the muscles used for morphological observation were stored in a closed centrifuge tube containing a fixed solution (Servicebio, G1111-100ML).
Measurement of muscle chemical composition
Analysis of muscle biochemical indicators included collagen, mycotoxins, total cholesterol, glucose, lactate and lactate dehydrogenase (LDH). The levels of glucose and total cholesterol were determined by following the instructions provided with the commercial kits purchased from Nanjing Jiancheng Bioengineering Institute. The levels of lactate and LDH activity were detected using the commercial kits purchased from Lunchangshuo Biotechnology Co., Ltd. The determination of total collagen in muscle samples followed the Association of Official Analytical Chemists (AOAC) method 990·26(Reference Kolar18). A conversion constant 7·25 was used to calculate the content of collagen from hydroxyproline content.
Haematoxylin–eosin staining
Haematoxylin–eosin staining of muscle samples was performed according to our previous study(Reference Liu, Du and Tu15). The diameter and cross-sectional area of myofibres are measured using Image J software (National Institutes of Health). The images were collected using an Aperio Scan Scope slide scanner (Aperio).
Assay of antioxidant activities in muscle and serum
Muscle and serum samples that were used to analyse antioxidant activity were stored at −80°C. The activities of superoxide dismutase (SOD), glutathione peroxidase, catalase (CAT), total antioxidant capacity and malondialdehyde (MDA) content were determined using the commercial kits according to the instructions (Nanjing Jiancheng Bioengineering Institute). The total protein concentrations were determined using a Pierce BCA protein assay reagent (Thermo Fisher Scientific). MDA content was shown as nmol/mg protein or nmol/mL. The enzyme activities were presented as U/mg protein or U/mL.
Lipidomic assay
Lipid sample preparation and lipidomic assays were referenced from our previous research(Reference Xu, Chen and Wang19). The LipidSearch software version 4.1 (Thermo Scientific™) was used for peak identification, peak extraction, lipid identification (secondary identification) and other treatments. The key parameters used were precursor tolerance of 5 ppm, product tolerance of 5 ppm and product ion threshold of 5 %. First, the quality of the data extracted by LipidSearch was evaluated before analysis was conducted. Data analysis encompassed identification quantity statistics, lipid composition analysis and lipid difference analysis. Lipid composition analysis comprised subclass composition and lipid content distribution analysis, while lipid difference analysis was based on lipid content, chain length and chain saturation.
Real-time quantitative PCR
RNA extraction and quantitative real-time PCR analysis were performed following the methodology outlined in our previous study(Reference Liu, Du and Tu15). The quality and concentration of the extracted RNA were determined using a NanoDrop 2000 instrument (Gene Company Limited). Reverse transcription was performed using a ReverAid First Strand cDNA Synthesis Kit (Thermo Fisher) with random primers to generate cDNA. Primer sequences used in the quantitative real-time PCR are listed in Table 1. Relative gene expression was analysed using the 2 −ΔΔCT method.
Table 1. Primer sequences used in this study
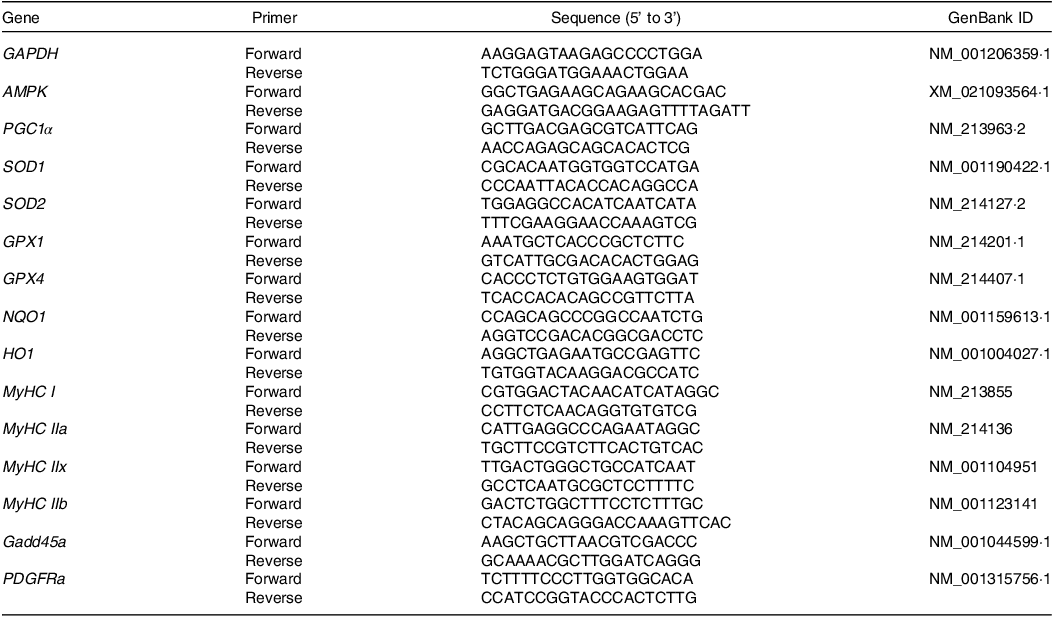
Western blotting
Western blotting analysis was conducted as previously described(Reference Xu, You and Zhou20).The anti-slow MyHC (Cat #: ab11083, mouse) and anti-fast MyHC (Cat #: ab91506, rabbit) antibody was purchased from Abcam, and the GAPDH (Cat #: EM1101) antibody was purchased from HuaAn Biotechnology.
Immunofluorescence staining
Immunofluorescence staining was performed as previously described(Reference You, Xu and Sun21). In brief, muscle sections were deparaffinised, and antigen retrieval was conducted. The sections were then blocked with blocking buffer containing 5 % goat serum, 2 % bovine serum albumin (BSA), 0·2 % triton X-100 and 0·1 % sodium azide in PBS for 1 h. Then the sections were incubated with antibody mouse anti-slow MyHC (ab11083, 1:200) and anti-fast MyHC (ab91506, 1:200) primary antibody overnight at 4°C. Then the sections were incubated with secondary antibodies (Alexa Fluor 488 or 594) for 1 h at room temperature. After washing with PBS for 5 min, DAPI (Roche) was used to label nuclei for 10 min. Fluorescent images were captured using a Leica DM 6000B fluorescent microscope.
Statistical analysis
The muscle biochemical indicators, gene and protein expression data were analysed using one-way ANOVA with SPSS 26·0 (IBM Corp). Duncan’s multiple range analysis was used for post-hoc tests. The results are shown as mean values with their standard error of the mean. GraphPad Prism 9 (GraphPad Software Inc.) was used for data visualisation. Significant differences were identified at P < 0·05. The correlation analysis in this study was performed using Pearson correlation analysis.
Results
Determinations of anti-nutritional factors
As shown in Table 2, we compared the contents of anti-nutritional factors in mixed feed and FF and observed that co-fermentation by Bacillus subtilis and Enterococcus faecalis decreased the levels of β-conglycinin, trypsin inhibitor and globulin in the feed ingredients.
Table 2. Contents of anti-nutritional factors in mixed feed and co-fermented feed (%, air-dry basis)
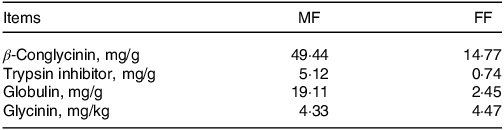
MF, mixed feed; FF, fermented feed (40 % maize, 40 % soyabean meal, 20 % wheat bran).
Effects of fermented feed on longissimus thoracis chemical composition
As displayed in Table 3, FF supplementation had no effect (P > 0·05) on muscle collagen and total cholesterol content. We further analysed the effects of FF on muscle glycolytic capacity, which is closely related to meat colour and water-holding capacity. FF showed no effects on muscle glucose content and LDH activity but reduced lactate content (P < 0·05) (Table 3).
Table 3. Effects of FF on longissimus thoracis biochemical indicators (Standard error of the means)
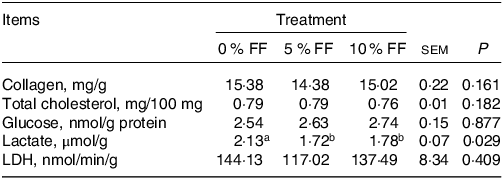
FF, fermented feed; LDH, lactate dehydrogenase.
a, b Within a row, values with different superscripts differ significantly at P < 0·05. Data are expressed as means ± sem, n 6.
Effects of fermented feed on muscle fibre characteristics
To explore the effects of FF on muscle fibre characteristics, we observed the morphology of myofibres by haematoxylin–eosin staining. The cross-sections of the myofibres are presented in Fig. 1(a). No differences were observed in the section area of muscle fibres among the three groups (Fig. 1(b)). As displayed in Fig. 1(c) and (d), the immunofluorescence staining results showed that the percentage of slow-twitch fibres in muscle was significantly up-regulated by FF supplementation. Further, we detected the expressions of muscle fibre type-related genes. 10 % FF significantly up-regulated MyHC I, MyHC IIa and MyHC IIx mRNA expression but decreased MyHC IIb mRNA expression (Fig. 1(e)). The slow MyHC protein expression in 10 % FF group was increased in comparison with 0 % FF group (Fig. 1(f)). The expressions of protein kinase AMP-activated catalytic subunit (AMPK) were significantly up-regulated in 5 % FF and 10 % FF group, only 10 % FF significantly increased PPAR-γ coactivator 1-α(PGC-1α) expression (Fig. 1(g)). Additionally, the expression of growth arrest and DNA-damage-inducible 45α(Gadd45a) was also significantly up-regulated in 5 % FF and 10 % FF group (Fig. 1(g)). There was no significant difference in the expression level of platelet derived growth factor receptorα (PDGFRa) mRNA among the three groups (Fig. 1(g)).
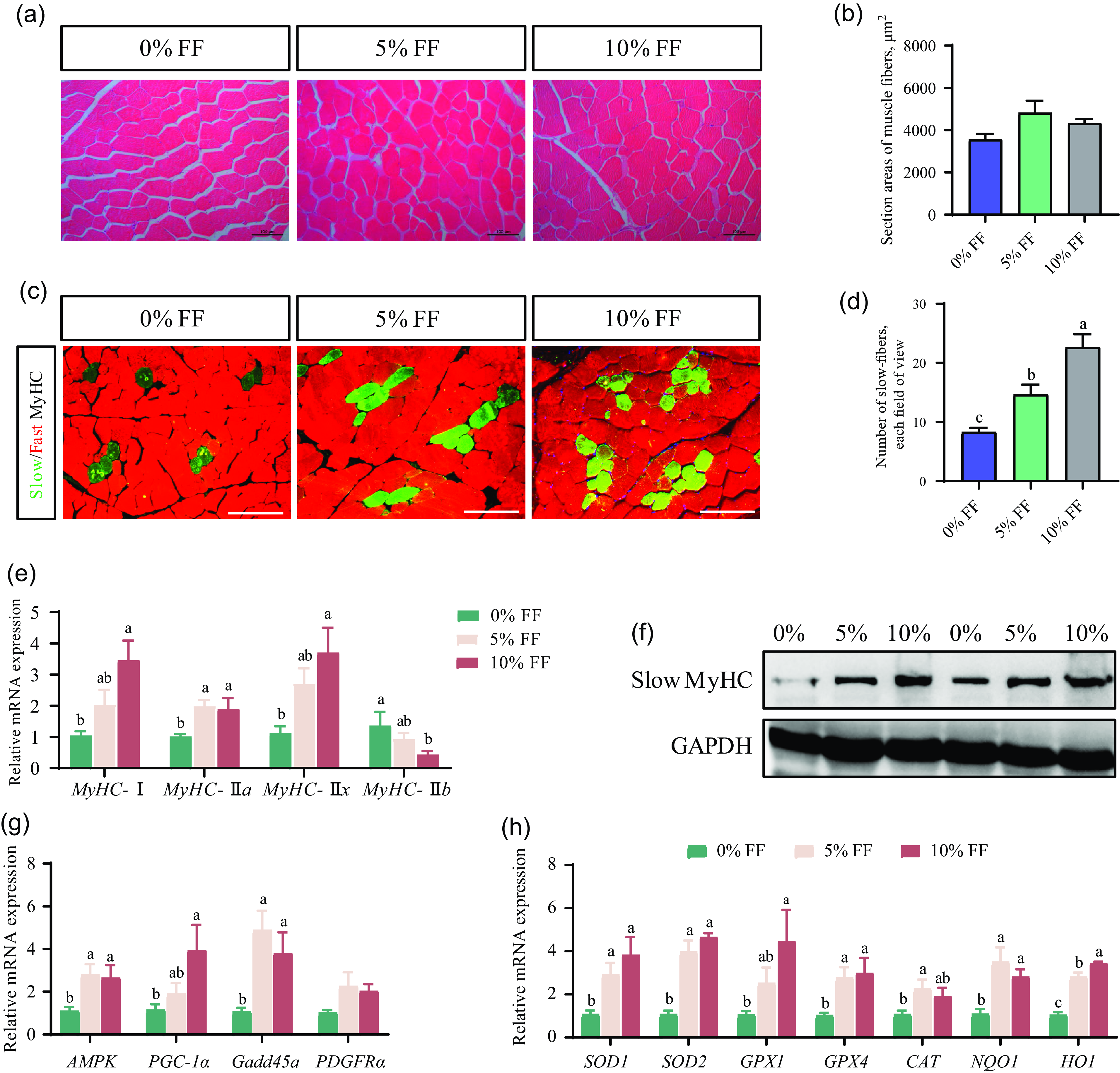
Fig. 1. Effects of FF on muscle fibre histology and characteristics. (a) The morphology of myofibres by H&E staining. (b) The section average area of muscle fibres. (c) The immunofluorescence staining of slow-MyHC and fast-MyHC in muscle section. (d) Statistics on the percentage of slow-twitch fibres in each field of view. (e) The mRNA expression levels of genes associated with muscle fibre type. (f) The expression levels of protein expression associated with muscle fibre type. (g) The gene relative expression levels of AMPK-PGC1α signalling pathway. (h) The expression levels of genes associated with antioxidant capacity. Data are expressed as the mean values with their standard errors (n 6). Values with different superscript letters are significantly different (P < 0·05). FF, fermented feed; H&E, haematoxylin–eosin.
Effects of fermented feed on antioxidant activity and malondialdehyde content
As shown in Table 4, the results revealed that the SOD activity (P < 0·05) in the serum and CAT activity (P < 0·05) in the muscle in 10 % FF group were significantly higher than 0 % FF. There was no significant difference in the MDA content among the three groups (Table 3). Furthermore, the mRNA levels of antioxidant-related genes in the muscle were detected. The mRNA expression of SOD1, SOD2, GPX4, NQO1 and HO1 was significantly up-regulated in 5 % FF and 10 % FF group. In comparison with 0 % FF, 10 % FF significantly increased the GPX1 mRNA expression levels. Moreover, 5 % FF markedly up-regulated the CAT mRNA levels (Fig. 1(h)). These data suggest that FF can improve the antioxidant activity of finishing pigs.
Table 4. Effects of FF on antioxidant enzyme activities and MDA content in serum and muscle (Standard error of the means)
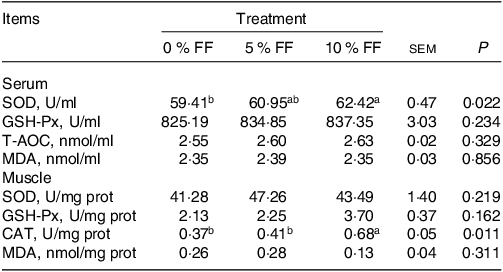
FF, fermented feed; MDA, malondialdehyde; SOD, superoxide dismutase; GSH-Px, glutathione peroxidase; T-AOC, total antioxidant capacity; CAT, catalase.
a, b Within a row, means assigned different superscripts indicate differences among diets (treatment 1 v. treatment 2 v. treatment 3). Data are expressed as means ± sem, n 6.
Effects of fermented feed on longissimus thoracis muscle lipid profiles
To further investigate the effects of FF on lipo-nutritional quality of pork, we conducted lipidomic assays to determine the lipid profiles in the longissimus thoracis. As shown in Fig. 2(a), there were a total of forty-four different lipid classes and 2240 different lipid species detected in the longissimus thoracis, including 721 TAG, 227 diglycerides, 256 PC, 212 phosphatidylethanolamine (PE), ninety-five phosphatidylserines and others. Our findings demonstrated that 5 % FF group had a higher proportion of glycerophospholipids and a lower proportion of glycerolipids than 0 % FF group, as shown in Fig. 2(b). Furthermore, 5 % FF displayed the highest percentage of PC and PE, while 10 % FF showed the widest distribution of TAG (Fig. 2(c)–(e)). 10 % FF also increased the percentage of MUFA and PUFA and decreased the percentage of SFA compared with those in 0 % FF group (Fig. 2(f)). The contents of TAG with different carbon atoms are shown in Fig. 2(g)–(i). In 5 % FF group, the contents of TAG with carbon atoms 32, 34, 40, 44, 52, 55, 59 and 74 were significantly reduced compared with those in 0 % FF. 10 % FF significantly decreased the contents of TAG (carbon atoms = 40, 44, 59 and 74) compared with the contents in 0 % FF group. 5 % FF and 10 % FF significantly increased the contents of TAG with thirty-three carbon atoms (Fig. 2(g)–(i)). Additionally, the contents of TAG with different acyl chain bonds showed that 5 % FF and 10 % FF decreased the contents of acyl chain with two double bond contents (Fig. 2(j)). These results highlight that 5 % FF increased the percentage of glycerophospholipids, PC and PE, and 10 % FF increased the percentage of TAG while decreasing the percentage of SFA in TAG.
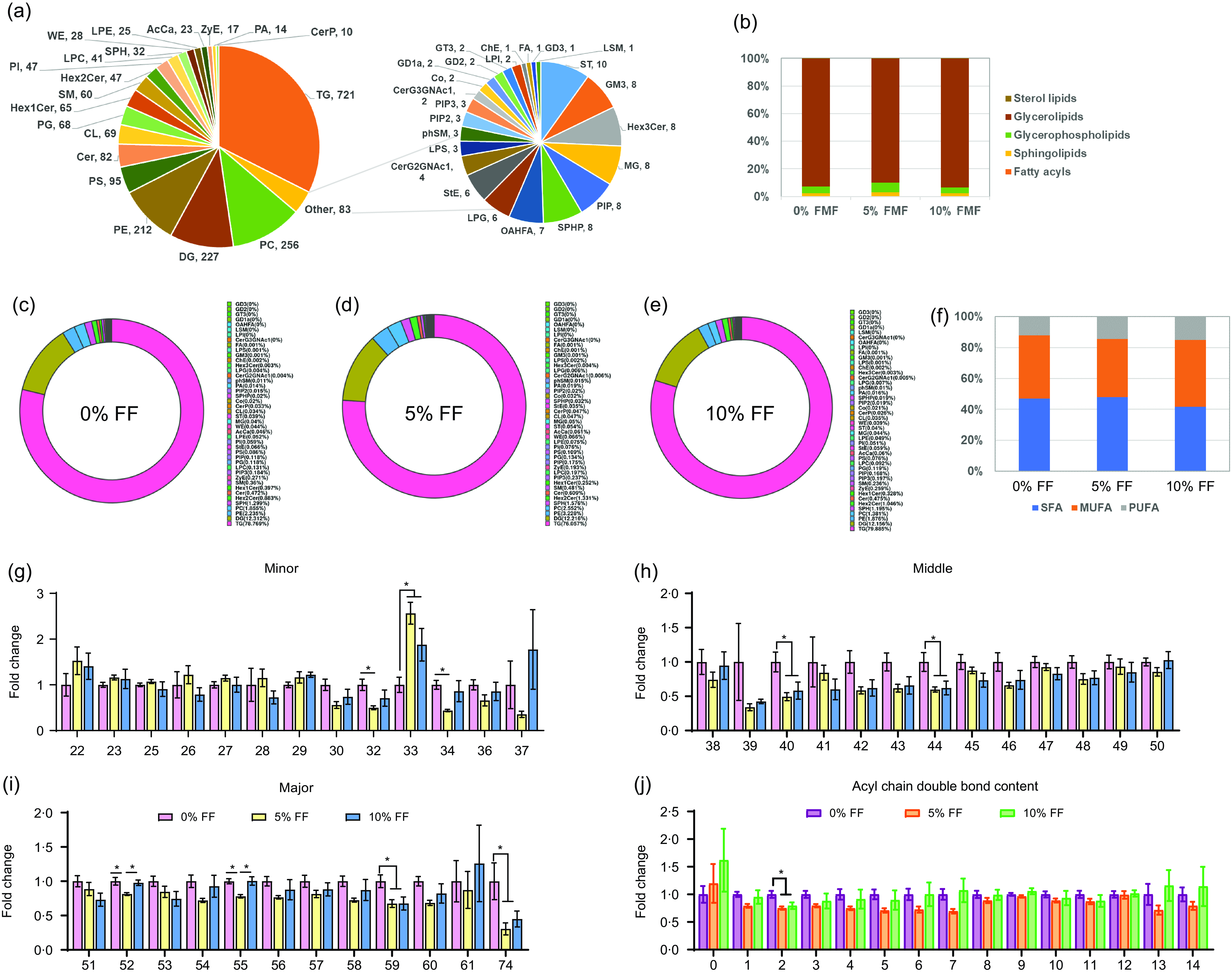
Fig. 2. Effects of FF on the overall lipid composition and distribution in muscle. (a) Distribution of lipid classes that were considered for subsequent analysis in all of the samples. (b) The composition of total lipids in the longissimus thoracis. (c)–(e) Proportion of different lipid species among the three groups. (f) TAG acyl chain percentages at different saturation levels. (g)–(i) TAG with different numbers of carbon atoms. (j) TAG with different numbers of double bond contents. Significant differences are indicated by ‘*’ and ‘**’ (*P < 0·05 and **P < 0·01) (n 6). FF, fermented feed.
Correlations between significantly differential lipids and meat quality indicators
To investigate the correlation between lipid molecules and meat quality, we first analysed the differential lipids among the three groups. A partial least squares discriminant analysis was conducted to investigate the effects of FF on muscle lipid profiles at the species level. The results showed that FF supplementation had limited effects on lipid profiles, as indicated in Fig. 3(a). Differential lipids were identified based on a variable importance for projection score > 1 and P < 0·05. As shown in Fig. 3(b), the Venn diagram showed the number of differential lipids among the three groups, and there are 254 differential lipids among the three groups. The top 20 differential lipids among the three groups were visualised through a heatmap in Fig. 3(c). The heatmap revealed that 5 % FF and 10 % FF significantly increased the contents of PA (49:4) and CL (72:8), while 10 % FF significantly increased the contents of PC (36:4e) and PE (36:1p) in comparison with 0 % FF. To further explore the correlations between the top 20 significantly differential lipids and muscle fibre characteristics, a Pearson correlation analysis was performed.
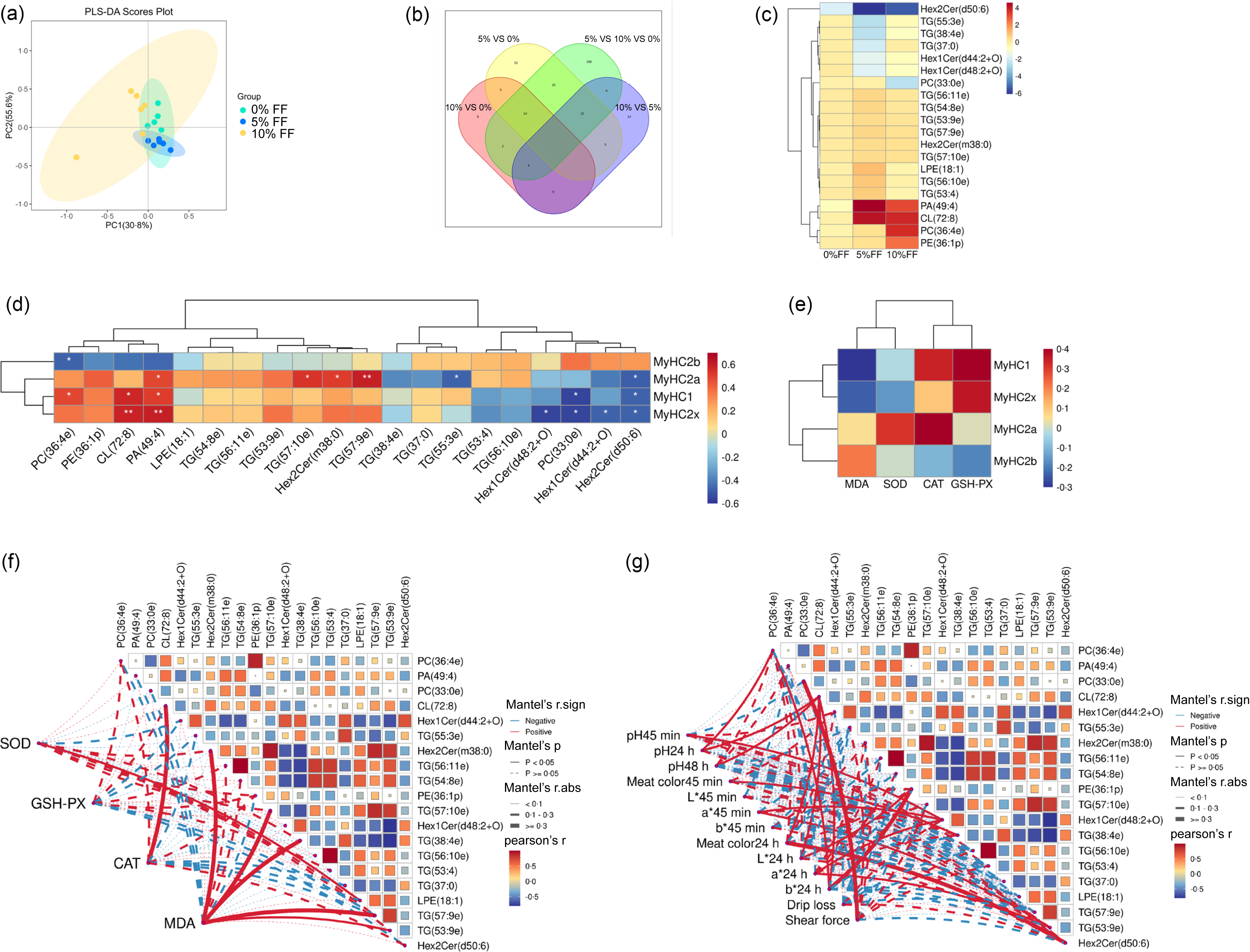
Fig. 3. Correlation analysis between differential lipids and meat quality indicators. (a) Score plots of lipid composition of the three groups were obtained by partial least squares discriminant analysis (PLS-DA). (b) The number of common and special lipids among groups is shown by Venn diagram. (c) The most significantly different twenty lipids were shown in the heatmap. (d) Pearson correlation analysis between significantly different lipids and expression levels of genes associated with muscle fibre type. Blue and red represent negative and positive correlations, respectively. (e) Pearson correlation analysis between antioxidant activity and expression levels of genes associated with muscle fibre type. (f) Pearson correlation analysis between significantly different lipids and antioxidant activity. (g) Pearson correlation analysis between significantly different lipids and meat quality indicators. Meat quality data cited from our previous research(Reference Liu, Du and Tu15). Significant correlations are indicated by ‘*’ and ‘**’ (*P < 0·05 and **P < 0·01) (n 6).
As shown in Fig. 3(d), PA (49:4) showed strongly positive correlations with MyHC I, MyHC IIa and MyHC IIx mRNA expression in muscle, and Hex2Cer(d50:6) was negatively related to MyHC I, MyHC IIa and MyHC IIx mRNA expression. CL (72:8) showed strongly positive correlations with MyHC I mRNA and MyHC IIx mRNA expression, but PC (33:0e) was negatively related to MyHC Iand MyHC IIx mRNA expression (Fig. 3(d)). These results suggested that PA (49:4), Hex2Cer (d50:6), CL (72:8) and PC (33:0e) may be molecular markers indicating muscle fibre characteristics. Correlations between muscle fibre characteristics and antioxidant activity are shown in Fig. 3(e), the activity of SOD, CAT and glutathione peroxidase showed positive correlations with MyHC I, MyHC IIa and MyHC IIx mRNA expression and the content of MDA was positively related to MyHC IIb mRNA expression. As shown in Fig. 3(f), CL (72:8) showed strongly positive correlations with CAT activity, and LPE (18:1) showed positive correlations with SOD activity. Hex2Cer (m38:0), TAG (57:10e), TAG (38:4e) and TAG (57:9e) showed strongly positive correlations with MDA content. Further, we also analysed the correlations between significantly differential lipids and meat quality indicators. As shown in Fig. 3(g), PC (33:0e) showed strongly positive correlations with shear force. CL (72:8) was positively related to pH24 h, pH48h, meat colour24 h, L*24 h and b*24 h. Hex2Cer (d50:6) showed strongly positive correlations with meat colour24 h.
Discussion
Recently, several studies have explored the effects of different types of FF on animal growth performance, immunity, intestinal health and animal products quality. Some of these studies have found that fermented Chinese herbal medicine(Reference Chen, Li and Liu22), fermented maize germ meal(Reference He, Zheng and Piao23), fermented mulberry leaves(Reference Zhang, Yin and Schroyen24) and fermented mixed feed(Reference Liu, Tu and Sun25,Reference Su, Jiang and Wang26) have positive effects on pig husbandry. However, the effects of FF based on maize–soyabean meal–wheat bran on muscle fibre types and muscle lipid composition in finishing pigs remain unclear. Our data can provide crucial theoretical evidence for utilising FF to enhance pork quality.
The presence of anti-nutritional factors in feed ingredients affects nutrient utilisation. Maize and soyabean meal are the major feed ingredients in Chinese pig production, and one of the primary measures to address the current shortage of feed ingredients and improve production efficiency is to mitigate the negative impact of anti-nutritional factors(Reference Wang, Lin and Su17). In this study, we chose maize, soyabean meal and wheat bran as fermentation substrates, and the co-fermentation by B. subtilis and E. faecium effectively reduced the content of anti-nutritional factors. The primary reason may be that B. subtilis could secrete extracellular enzymes degrading anti-nutritional factor(Reference Yao, Li and Li27).
The pH of meat is an essential indicator of its quality and is closely related to muscle denaturation(Reference Lerfall, Roth and Skare28). Lactate is an important contributor to the pH of meat, and the content of lactate is significantly negatively correlated with pH and positively correlated with drip loss(Reference Edwards, Engle and Correa29). Our findings demonstrated that FF supplementation reduced lactate content, which is considered a positive sign for improving meat quality. During the early postmortem period, glycolysis-based ATP production is crucial in maintaining muscle biological activity; however, this process is negatively correlated with pH value(Reference Yan, Nian and Zou30). To further investigate the effects of FF on muscle glycolytic potential, we analysed the LDH activity and glucose content. Our data revealed that FF supplementation did not significantly affect the LDH activity and glucose content. We speculate that FF may reduce the lactate content by influencing other steps in glycolysis.
Muscle fibre type is an essential factor that affects various aspects of meat quality, including tenderness, water-holding capacity, meat colour and pH value(Reference Zhang, Luo and Yu31). Four different types of skeletal muscle fibres can be classified based on their metabolic and contractile characteristics, which are type I expressing MyHC I, type IIa expressing MyHC IIa, type IIx expressing MyHC IIx and type IIb expressing MyHC IIb(Reference Xu, Chen and Huang2,Reference Schiaffino and Reggiani32) . Recently, we have previously found that supplementing FF significantly reduces shear force and improves tenderness(Reference Liu, Du and Tu15). In this study, we further demonstrated that FF supplementation increased slow-twitch fibre percentage and promoted skeletal fibre switching from glycolytic type II to oxidative type I. Similarly, another study demonstrated that FF reduced shear force and up-regulated MyHC I mRNA expression(Reference Qiu, Li and Zhao33). According to the results of this study, one possible reason for FF increasing the proportion of slow-twitch muscle fibres may be the activation of the AMPK/PGC1α signalling pathway. However, the specific molecular mechanism how FF alters the muscle fibre types via AMPK/PGC1α signalling remains unclear. It would be worthwhile to explore whether any unknown active factors in FF could regulate the muscle fibre switching process. Additionally, lipid is one of the most important components of pork, in addition to storing energy, it can also be involved in signal transduction(Reference Yoon, Shaw and Haigis10). For instance, accelerated PC turnover in macrophages promoted adipose tissue inflammation in obesity(Reference Petkevicius, Virtue and Bidault34). CL metabolism regulated muscle differentiation through MyoD1(Reference Vo, Schmidtke and Da Rosa-Junior35). In this study, we used lipidomics to uncover a few key lipid molecules that may be closely related to muscle fibre types, such as PA (49:4), Hex2Cer (d50:6), CL (72:8) and PC (33:0e). Although this correlation needs to be further verified, it at least indicates that there is an interaction between lipids and muscle development and muscle fibre types. Further research on the relationship between lipids and muscle development may provide new ideas for improving pork quality.
It is well known that improving the antioxidant capacity by dietary treatments can enhance meat quality and preservation properties of meat products(Reference Xu, Chen and Huang2,Reference Shakoor, Zhang and Xie12) . Our data demonstrated that FF supplementation increased the antioxidant capacity of muscle and serum. Similarly, a recent study also reported that supplementing 8 % FF in finishing pig models increased the activities of SOD and glutathione peroxidase and decreased MDA content in serum, indicating an improved antioxidant capacity of both muscle and serum(Reference Hao, Su and Zhang14). A recent study suggested that supplementation with n-3 fatty acids improved calf growth and antioxidant status(Reference Śpitalniak-Bajerska, Szumny and Pogoda-Sewerniak36). In our previous study, we observed that microbial co-fermentation increased the content of PUFA and n-3 PUFA in feed ingredients(Reference Liu, Du and Tu15), which may be one of the reasons why FF increased the antioxidant capacity of finishing pigs.
Although Duroc × Berkshire × Jiaxing Black pigs are a popular commercial pig breed in the Jiangnan region of China, their muscle lipid composition has not been widely studied. Furthermore, there is a lack of research on the effect of FF on the lipid composition and lipo-nutritional quality of pork, and the relationship between lipids such as glycerolipids and sphingolipids and pork quality remains unclear. This study identified glycerolipids and glycerophospholipids as the two most abundant lipids in the longissimus thoracis muscle. A similar profile of predominantly glycerolipids and glycerophospholipids was also found in the muscles of Heigai pigs(Reference Wang, Li and Duan37). The major glycerolipids in pork are TAG and diglycerides, of which TAG are mostly stored in adipose and muscle tissue and are one of the decisive factors of intramuscular fat contents(Reference Frayn, Arner and Yki-Järvinen38). Our results demonstrated that 10 % FF supplementation increased the percentage of TAG in total lipids. Previous studies have shown that glycerophospholipids play a key role in the Maillard reaction during the late heating process, and their oxidative degradation contributes to the formation of aldehydes, ultimately improving meat flavour(Reference Zhang, Zang and Zhang39). Our data showed that the percentage of total glycerophospholipids was increased in 5 % FF group. Notably, we have recently found that Gadd45a promotes fat deposition in skeletal muscle(Reference You, Liu and Ji40). In this study, it was observed that 10 % FF may increase the proportion of TAG by up-regulating Gadd45a expression. In this study, the increase in the proportion of TAG in the 10 % FF group may be related to the up-regulation of Gadd45a expression. Another interesting point is what kind of relationship exists between lipids and meat quality, which has gradually attracted the attention of researchers. Two glycerol phospholipids, phosphatidylinositol and phosphatidylserine, were thought to contribute to intramuscular fat deposition(Reference Zhou, Zhang and Wu41). In this study, we found that 10 % FF decreased PC (33:0e) content, and PC (33:0e) was strongly positively correlated with shear force and negatively correlated with MyHC I and MyHC IIx mRNA expression. PC (36:4e) was positively correlated with pH24 h and b*24 h. These results suggested that PC were closely related to meat quality, especially muscle fibre type. Further research is needed to investigate the interaction mechanisms between lipids and muscle fibre types, which will provide new insights into the regulation of meat quality.
Conclusion
Our results showed that 10 % FF increased the antioxidant capacity and slow-twitch fibre percentage of finishing pigs, contributing to the improvement of meat quality and preservation properties (Fig. 4). 10 % FF resulted in an increase in the percentage of TAG. The Pearson correlation analysis indicated that certain molecular markers (PA(49:4), Hex2Cer(d50:6), CL (72:8) and PC(33:0e)) could be used to indicate the characteristics of muscle fibres. According to the results of this study, supplementing 10 % FF is recommended as a better choice for enhancing the meat quality of finishing pigs. These results indicate that FF may be an effective nutritional strategy for improving the tenderness and nutritional quality of pork and may provide new insights for further production of high-quality pork by understanding the relationship between muscle fibre characteristics and lipid composition. However, the specific mechanisms by which FF regulates meat quality and the interaction between lipids and meat quality still need be further explored.
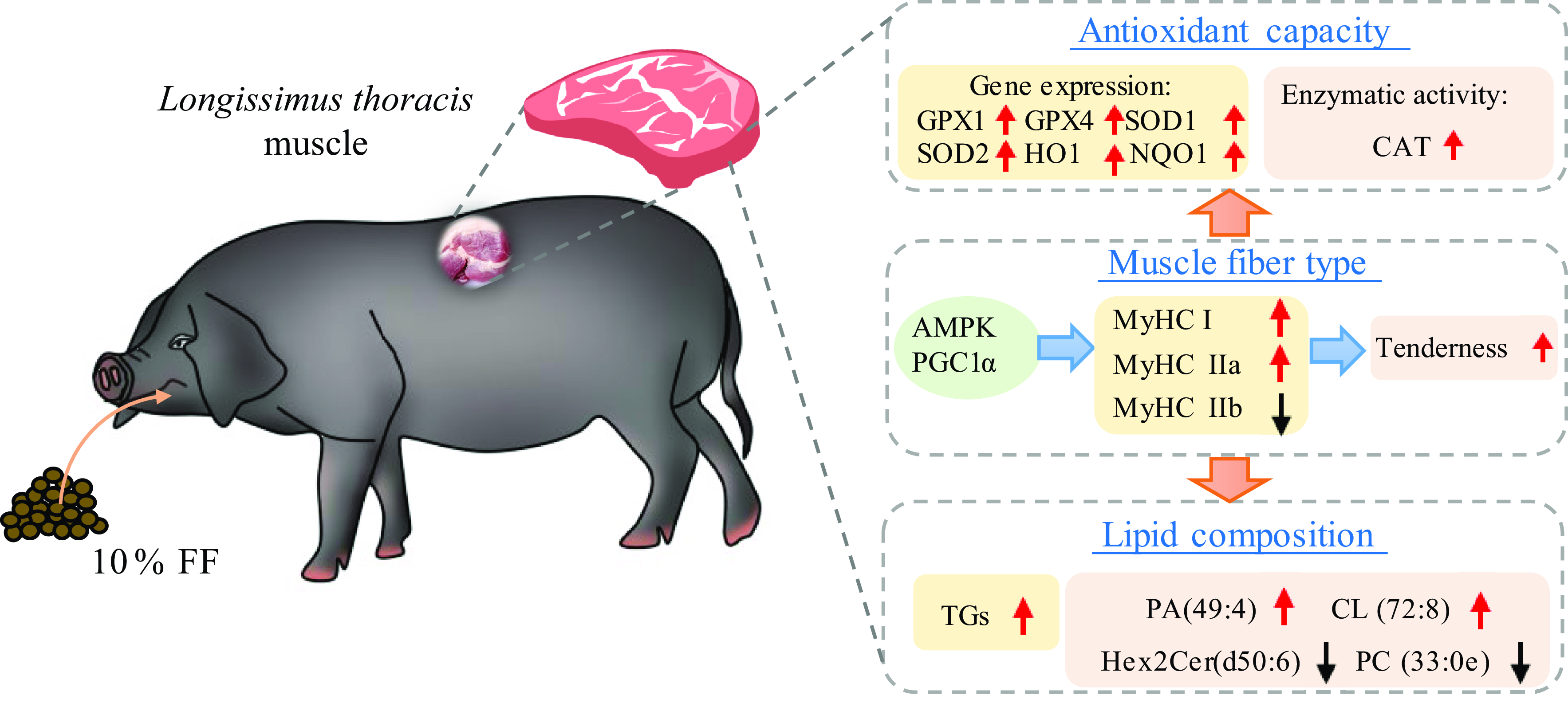
Fig. 4. A working model of the effects of FF on the muscle fibre characteristics, antioxidant capacity and lipid composition of pork. FF increases the slow-twitch fibre percentage and tenderness through AMPK/PGC-1α signalling pathway and improves antioxidant capacity and the portion of TAG in pork. FF, fermented mixed feed.
Acknowledgements
This work was financially supported by the National Key R&D Program of China (2021YFC2103005) and the Zhejiang Provincial Key R&D Program of China (2021C02008) to TZS. We thank the members of the Shan Laboratory and the employees of Zhejiang Qinglian Food Co. Ltd for help.
T. S. conceived and designed the experiments. S. L. performed the experiments and wrote the manuscript. M. D., J. S. and Y. T. helped perform the experiments. X. G. and P. C. did the laboratory analyses. Z. L. and Y. W. reviewed the manuscript. All authors have read and approved the final manuscript.
The authors declare that there are no conflicts of interest.