The contribution of microenvironment host cells to tumour progression is now well established, and fibroblasts are one of the most frequent cell types capable of organising a dialogue with tumour cells( Reference Witz 1 – Reference Calorini and Bianchini 4 ). Fibroblasts represent an extremely multifunctional cell type that may play an important regulatory role in several steps during tumour progression( Reference Kalluri and Zeisberg 2 , Reference Orimo, Gupta and Sgroi 5 , Reference Erez, Truitt and Olson 6 ). They are capable of producing various paracrine inflammatory mediators such as peptide growth factors, cytokines, chemokines, several proteases and their inhibitors. Fibroblasts undergo important modifications in the tumour stroma, in particular the population of fibroblasts adjacent to transformed epithelial cells as reported in several tumour histotypes. In addition, human studies describing genetic alterations in breast cancer tissues showed that some alterations in fibroblasts precede those in the epithelial component, suggesting a role of fibroblasts in epithelial cell transformation( Reference Allinen, Beroukhim and Cai 7 ). Similarly, in prostate cancers, loss of p53 in fibroblasts precedes loss of p53 in the epithelium( Reference Hill, Song and Cardiff 8 ).
The phenotype of these so-called cancer-associated fibroblasts (CAF) has been intensely investigated in recent years. Dvorak( Reference Dvorak 9 ) showed that a certain population of tumour stroma fibroblasts shares similarities with inflammatory fibroblasts present in the granulation tissue, including structural features recalling smooth-muscle cells, such as expression of α-smooth muscle actin (α-SMA). These CAF have been referred to as ‘myofibroblasts’( Reference Hinz, Phan and Thannickal 10 ). It is interesting to note that α-SMA expression is not mandatory for fibroblast activation and populations of fibroblasts, whether expressing α-SMA or not, have been recognised in tumour tissues. However, the common trait of these heterogeneous fibroblast populations is their reactivity and secretion of factors affecting tumour aggressiveness( Reference Giannoni, Bianchini and Masieri 11 , Reference Sugimoto, Mundel and Kieran 12 ).
Epithelial–stromal interactions are considered to be particularly important for the development and progression of prostate cancer, one of the most frequent cancers in industrialised nations( Reference Chung, Baseman and Assikis 13 – Reference Verona, Elkahloun and Yang 15 ). Epithelial–stromal cross-talk is necessary for normal prostate development, during which the mesenchyme of the urogenital sinus determines the growth and differentiation of the prostate gland, and epithelial–mesenchymal interactions are maintained during life. De Wever & Mareel( Reference De Wever and Mareel 16 ) reviewed the interplay between cancer cells and stromal cells, and proposed that two pathways, called ‘efferent’ and ‘afferent’, determine the final effect on tumour progression. We recently demonstrated that in the efferent pathway, prostate cancer cells stimulate a reactive response in human prostate fibroblasts, which undergo a clear activation( Reference Giannoni, Bianchini and Masieri 11 ). In turn, activated fibroblasts stimulate through an matrix metalloproteinase (MMP)-dependent pathway an epithelial–mesenchymal transition (EMT) in prostate cancer cells, associated with an enhanced in vivo tumour growth and spontaneous metastatic dissemination. In addition, an enhanced expression of stem cell markers was found to characterise prostate cancer cells undergoing the EMT upon contact with CAF. Fibroblasts, expressing α-SMA, from the tumour region of an adenocarcinoma-bearing prostate gland stimulate tumour formation, metastatic spread and stem cell markers in prostate cancer cells with an efficiency similar to that of in vitro transforming growth factor-β (TGF-β)-activated prostate fibroblasts( Reference Giannoni, Bianchini and Masieri 11 ).
A recent study on cancer risk indicated that environment and lifestyle play a significant role in the development and progression of certain neoplastic disease, including prostate cancers( Reference Chavarro, Stampfer and Li 17 ). The most important factor modulating prostate cancer risk appears to be the diet, and also epidemiological and experimental studies have implicated fat and meat as the major risk factors. A diet enriched in SFA and n-6 PUFA usually correlates with an increased risk and progression of tumours( Reference Neuhouser, Barnett and Kristal 18 ), while n-3 PUFA, mainly EPA (20 : 5n-3) and DHA (22 : 6n-3), were found to exert a protective effect against some common cancers, including prostate carcinoma( Reference Reddy 19 – Reference McEntee, Ziegler and Reel 22 ). Findings that n-3 PUFA-enriched diets inhibited spontaneous and chemically induced carcinogenesis confirm their protective effects( Reference Rose and Connolly 23 ). In addition, our previous work demonstrated that murine metastatic melanoma cells reduced their capacity to colonise the lung after an endovenous injection into animals fed a fish oil diet( Reference Mannini, Kerstin and Calorini 24 ). The lung-colonising potential of murine melanoma cells was also reduced after previous treatment with media enriched with EPA( Reference Reich, Royce and Martin 25 ).
The mechanisms underlying the beneficial effects of n-3 PUFA on prostate cancer are still poorly understood, and there are no studies analysing whether the malignant phenotype of prostate cancer cells promoted by fibroblasts might be inhibited by n-3 PUFA. We used primary skin fibroblasts as a representative stromal-derived cell model of fibroblast-to-myofibroblast differentiation and subsequently we used prostate-derived stromal cells as a more appropriate model for the human prostate stroma( Reference Giannoni, Bianchini and Masieri 11 ). In particular, prostate fibroblasts were isolated from both healthy and cancerous regions of an adenocarcinoma-bearing prostate gland, called non-CAF (NCAF) and CAF, respectively, to test the hypothesis that inhibition of prostate stromal fibroblast differentiation into myofibroblast (a mesenchymal-to-mesenchymal transition, MMT) by n-3 PUFA is capable of blocking tumour-promoting activities and prostate cancer progression.
Materials and methods
Cell lines and culture conditions
Human prostate carcinoma cell line (PC3) was obtained from the American Type Culture Collection and grown in Dulbecco's modified Eagle's medium (DMEM) containing 4500 mg glucose/l (DMEM 4500; Lonza) supplemented with 10 % fetal calf serum (complete medium; Invitrogen), at 37°C in a 10 % CO2 humidified atmosphere. Cultures were periodically monitored for mycoplasma contamination using Chen's fluorochrome test( Reference Chen 26 ).
Human dermal fibroblasts were kindly donated by Daniela Monti, Department of Experimental Pathology and Oncology, University of Florence, while human prostate fibroblasts were isolated from surgical explantations( Reference Giannoni, Bianchini and Masieri 11 ). Briefly, tissue samples from healthy and cancerous regions of prostate carcinoma-bearing patients (Gleason 4+5) were obtained aseptically from patients undergoing radical prostatectomy, and digested overnight with 1 mg collagenase I/ml in PBS. NCAF and CAF collected from the healthy and cancerous regions, respectively, were grown in 10 % fetal calf serum-DMEM medium.
Ethyl esters of 20 : 4n-6 arachidonic acid (AA), EPA (20 : 5n-3) or DHA (22 : 6n-3) were provided from Cayman Chemical in ethanol solution, shipped in dry ice. Fatty acids were stocked under N2 and stored at − 80°C. For the experiments, fibroblasts (passages 2–4) were grown to subconfluence in complete standard medium, and exposed for the next 24 h to PUFA in a serum-free medium with the addition of 250 μg/ml bovine serum albumin (BSA; Sigma; DMEM-BSA). AA, EPA or DHA were diluted from a stock solution to the final desired concentration in a DMEM-BSA medium and used immediately; an equal amount of ethanol, not exceeding 0·001 %, was used for untreated fibroblasts. Fibroblasts were next exposed for 24 h to a fresh DMEM-BSA medium in the absence or in the presence of recombinant human TGF-β (PeproTech, 10 ng/ml; TGF-β-NCAF) which is known to induce fibroblast differentiation( Reference Giannoni, Bianchini and Masieri 11 ). Fibroblast and myofibroblast cultures were then grown in fresh DMEM-BSA media for an additional 24 h (conditioned media, CM).
Cell viability assay
NCAF were seeded in 60 mm plates in standard conditions. After 24 h, cells were exposed to a DMEM-BSA medium and divided into control and treatment groups (AA, EPA or DHA (30–90 μm)). Again, after 24 h, cells were exposed to a fresh DMEM-BSA medium in the absence or in the presence of recombinant TGF-β, and 24 h later, cells were harvested, stained with trypan blue and counted under an inverted-phase microscope (quadruplicate haemocytometer counts of duplicate cultures were performed).
Invasion assay
PC3 cells (1·5 × 105) were either resuspended in fibroblast CM or in a DMEM-BSA medium, and then placed inside 12 mm, 8·0 μm pore, Millicell Culture Plate Inserts (Millipore), coated with Matrigel (50 μg/filter; BD); standard medium was placed in twenty-four-well culture plates. Migration was allowed to occur for 4–6 h at 37°C in 10 % CO2 humidified atmosphere. Inserts were fixed in cold methanol and stained with DiffQuick solution. Cells on the upper surface of the filter were removed with a cotton swab. Migrated cells were counted by a light microscope (10 × ) in ten random fields per each well. In separate experiments, PC3 cells were grown in fibroblast CM for 72 h, and then allowed to migrate in DMEM-BSA medium. Each treatment was performed at least in duplicate. Mean values of the migrated cells for each point were calculated from three independent experiments.
Zymography
Aliquots of different fibroblast cultures CM and human HT1080 fibrosarcoma cell medium, used as markers of molecular weight, were fractionated by 8 % SDS–PAGE gels co-polymerised with 0·1 % (w/v) type A gelatin. After electrophoresis, the gels were washed in 2·5 % (v/v) Triton X-100 for 30 min to remove sodium dodecyl sulfate. Gelatin substrate gels were then incubated in 50 mm-Tris–HCl, pH 7·4, 200 mm-NaCl and 5 mm-CaCl2 for 24 h at 37°C. After incubation, the gels were stained with 0·1 % Coomassie brilliant blue for 60 min at room temperature in a solution of acetic acid, methanol and distilled water (1:2:3, by vol.). After destaining, the gels were immersed in distilled water, scanned immediately and analysed with Image J for Windows (http://imagej.nih.gov/ij/index.html). Gelatinolytic activity was demonstrated as clear bands on a blue background.
Western blot analysis
PC3 cells exposed to fibroblast CM for 24 h and fibroblast cultures were lysed for 10 min on ice in complete radioimmunoprecipitation assay lysis buffer (0·1 % SDS, 0·5 % deoxycholate, 50 mm-Tris–HCl, pH 7·5, 150 mm-NaCl, 1 % Nonidet P-40, 2 mm-ethylene glycol tetraacetic acid, 1 mm-sodium orthovanadate, 1 mm-phenyl-methanesulphonyl-fluoride and 1:1000 Protease Inhibitor Cocktail Set III (Calbiochem)). The lysates were clarified by centrifugation. An equal amount of protein from each sample was run on SDS–PAGE and transferred onto a polyvinylidene fluoride membrane. Immunoblots were probed first with specific primary antibodies diluted 1:1000 (mouse anti-human α-SMA, α-SMA, A2547 from Sigma; rabbit anti-human Met, SC-161, rabbit anti-human E-cadherin SC-7870, mouse anti-human α-tubulin SC-58 667 from Santa Cruz) in 1 % BSA, 0·05 % Tween 20 in Tris-buffered saline buffer (10 mm-Tris, pH 7·5, 100 mm-NaCl), and then with secondary antibodies conjugated with horseradish peroxidase (AP132P and AP124P, 1:10 000; Millipore) washed, and developed with the ECL Advance Western blotting detection kit (GE Healthcare).
Immunofluorescence
Cells, cultured on 25 mm coverslips, were fixed in 4 % paraformaldehyde and membranes were permeabilised in 0·1 % Triton X-100 solution. Coverslips were incubated in blocking solution (PBS supplemented with 4 % BSA and 1 % horse serum) and then incubated at 4°C overnight with anti-α-SMA primary antibodies, washed and incubated for 1 h with sheep Cy3 anti-mouse antibodies (C2181; Sigma).
Xenograft experiments
Male severe combined immunodeficient-bg/bg mice (6–8 weeks old; Charles River Laboratories International) were fed with a regular chow diet (Harlan Laboratories) and water ad libitum. The animals were injected subcutaneously with 100 μl of PBS containing 1 × 106 PC3 cells plus 0·5 × 106 fibroblasts, incubated for 1 h at room temperature before injection. The animals were monitored daily and tumour size was measured every 2–3 d by a caliper; tumour volumes were determined by the formula:

where L and W are the length and width of the masses.
Mice were killed before the tumour masses exceeded a size to produce evident physical discomfort such as rough hair coat, lack of grooming activity or abnormal posture (huddling, hunching or being stiff).
Statistical analysis
Densitometric data were analysed by a multiple-comparison Student–Newman–Keuls test, after demonstration of significant differences among medians by non-parametric variance analysis, according to the Kruskal–Wallis test. Data are expressed as means and standard errors of the mean, depicted by vertical bars, of the indicated number of experiments.
Ethics approval
All experimental procedures involving animals were performed in accordance with national guidelines, approved by the ethical committee of Animal Welfare Office of Italian Work Ministry and conformed to the legal mandates and Italian guidelines for the care and maintenance of laboratory animals.
Results
DHA prevents differentiation of transforming growth factor-β-stimulated fibroblasts into myofibroblasts
In the present study, before using our experimental model of different populations of prostate fibroblasts, we tested whether the parent n-3 compounds DHA and EPA or a prototype of n-6 PUFA, AA, inhibited the TGF-β-stimulated differentiation of skin fibroblasts into myofibroblasts.
To determine the potency of DHA, EPA and AA as inhibitors, we used morphological evidences and Western blot analyses of α-SMA expression levels correlating with the myofibroblast-like phenotype. Among the long-chain fatty acids used in the experiments, DHA inhibited the differentiation of skin fibroblasts into myofibroblasts. In TGF-β-stimulated fibroblasts, 30 μm-DHA abrogated the typical myofibroblast morphology characterised by a large number of actin stress fibres as well as α-SMA expression (Fig. 1(A) and (B)).
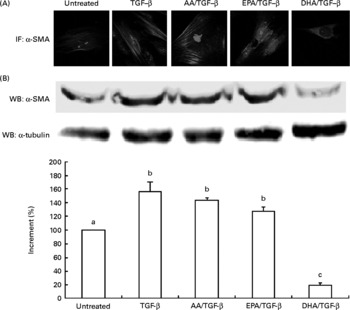
Fig. 1 (A) Immunofluorescence (IF; magnification × 100) and (B) Western blot (WB) analysis of α-smooth muscle actin (α-SMA) expression in human dermal fibroblasts treated with 30 μm of 20 : 4n-6 arachidonic acid (AA), EPA or DHA and then stimulated with transforming growth factor-β (TGF-β). Values are means of three independent experiments, with their standard errors of the mean represented by vertical bars. α-SMA expression was quantified by a densitometric analysis of three blots, normalised against α-tubulin and expressed as the percentage of increment. a,b,c Mean values with unlike letters were significantly different (P < 0·005). A colour version of this figure can be found online at http://www.journals.cambridge.org/bjn
Organ-specific fibroblasts were isolated from the healthy region of a prostate gland resected from prostate adenocarcinoma patients (NCAF) and grown in vitro as reported in a previous paper( Reference Giannoni, Bianchini and Masieri 11 ). NCAF did not show aspects of myofibroblast differentiation, and only after the TGF-β treatment, they expressed α-SMA and the typical myofibroblast morphology. First, we investigated the effect of the AA, EPA or DHA treatment on NCAF viability, and we found that, among the PUFA used in the experiment, 60 and 90 μm-DHA induced cell death both in NCAF and in TGF-β-stimulated NCAF (20–50 % of cells; Fig. 2(A)). We also found that neither EPA nor AA was able to inhibit TGF-β-induced NCAF differentiation as demonstrated by the high levels of α-SMA expression in these fibroblasts (Fig. 2(B)). Immunofluorescence and Western blot analysis of α-SMA demonstrated that 30 μm-DHA, a dose that did not modify viability of NCAF, inhibited NCAF differentiation into myofibroblasts (Fig. 2(C) and (D)). In addition, both EPA and AA at 60 and 90 μm increased α-SMA, a finding indicating that the effect of DHA is not mediated through n-6 PUFA or AA, but acts through a different mechanism, otherwise EPA would have the same effects as DHA since they both antagonise AA equally (Fig. 2(B)).
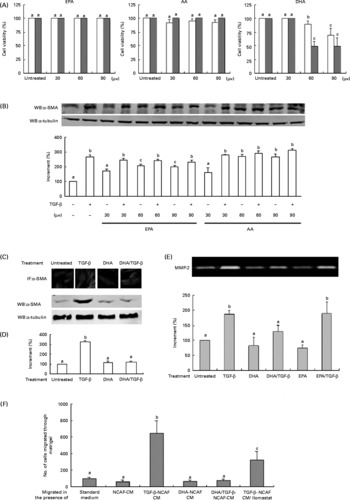
Fig. 2 Effect of PUFA on non-cancer-associated fibroblast (NCAF) differentiation. (A) Cell viability. Cell viability was determined by trypan blue exclusion assay in NCAF treated with 20 : 4n-6 arachidonic acid (AA), EPA or DHA at a dose range from 30 to 90 μm and then stimulated with transforming growth factor-β (TGF-β) (quadruplicate haemocytometer counts of duplicate cultures). a,b,c Mean values with unlike letters were significantly different (P < 0·01). □, Unstimulated;
, TGF-β stimulated. (B) α-Smooth muscle actin (α-SMA) expression in TGF-β-stimulated NCAF treated with EPA or AA (30–90 μm) and densitometric analysis of three independent experiments (determined as in Fig. 1). a,b,c Mean values with unlike letters were significantly different (P < 0·05). (C) α-SMA expression in (30 μm) DHA-treated TGF-β-stimulated NCAF determined by immunofluorescence and (D) Western blot (WB) and densitometric analysis of five independent experiments (determined as in Fig. 1). a,b Mean values with unlike letters were significantly different (P < 0·005). (E) Change in matrix metalloproteinase-2 (MMP-2) release in media conditioned by (30 μm) DHA- or EPA-treated TGF-β-stimulated NCAF, zymogram and densitometric analysis of three independent experiments normalised by the number of NCAF. a,b Mean values with unlike letters were significantly different (P < 0·002). (F) Invasiveness through Matrigel of PC3 prostate adenocarcinoma cells exposed to fibroblast conditioned media (CM). Values are means of three independent experiments, with their standard errors of the mean represented by vertical bars. a,b,c Mean values with unlike letters were significantly different (P < 0·005). PC3, human prostate carcinoma cell line. A colour version of this figure can be found online at http://www.journals.cambridge.org/bjn
In a previous paper( Reference Giannoni, Bianchini and Masieri 11 ), we demonstrated that TGF-β-stimulated NCAF promote aggressiveness of prostate adenocarcinoma cells; here we demonstrated that media conditioned by DHA-treated TGF-β-stimulated NCAF failed to promote the invasiveness of PC3 cells. Furthermore, we ascribed this effect to the reduced MMP-2 release by DHA-treated TGF-β-stimulated NCAF. Indeed, DHA, but not EPA, was able to inhibit MMP-2 release in TGF-β-stimulated NCAF (Fig. 2(E)). The dependence of PC3-enhanced migration from MMP-2 secreted by TGF-β-stimulated NCAF was strengthened by Ilomastat, a broad range MMP inhibitor, which reduced the increased capacity of PC3 cells to cross Matrigel layers (Fig. 2(F)).
DHA prevents the epithelial–mesenchymal transition in prostate adenocarcinoma cells
We previously demonstrated that activated fibroblasts through the secretion of MMP may promote the EMT in PC3 cells, thereby enhancing cancer cell aggressiveness( Reference Giannoni, Bianchini and Masieri 11 ). To verify whether the MMT inhibited by DHA was also associated with an abrogation of the capacity of TGF-β-stimulated NCAF treated with DHA to stimulate the EMT in PC3 tumour cells, we analysed cell morphology, some specific markers of EMT and invasiveness of PC3 cells grown under different CM. The PC3 cells, which have undergone the EMT in media conditioned by TGF-β-stimulated NCAF, expressed a mesenchymal morphology, invaded Matrigel-coated filters in association with an enhanced expression of the proto-oncogene c-Met and reduction of E-cadherin (EMT promotes in tumour cells a mesenchymal phenotype, a reduced cell-to-cell contact as well as an increased migration exploiting degradation of three-dimensional matrices). Pre-treatment of TGF-β-stimulated NCAF with 30 μm-DHA abolished the PC3 expression of mesenchymal morphology, c-Met expression and invasiveness (Fig. 3(A), (B) and (D)), and levels of E-cadherin were restored although not completely (Fig. 3(C)). Thus, the DHA treatment inhibited not only the MMT in NCAF but also the capacity of TGF-β-stimulated NCAF to induce several properties involved in the EMT of prostate cancer cells.

Fig. 3 Change in morphology, epithelial–mesenchymal transition markers and invasiveness of PC3 prostate adenocarcinoma cells grown in a medium conditioned by (30 μm) DHA-treated transforming growth factor-β (TGF-β)-stimulated non-cancer-associated fibroblasts (NCAF). (A) Contrast microscopy images of PC3 cells exposed for 72 h to different NCAF conditioned media (CM). (B) c-Met (
) and (C) E-cadherin (□) Western blot densitometric analysis of three independent experiments (determined as in Fig. 1). a,b,c Mean values with unlike letters were significantly different (P < 0·05). (D) Invasiveness through Matrigel of PC3 cells exposed for 72 h to the different CM. Values are means of three independent experiments, with their standard errors of the mean represented by vertical bars. a,b,c Mean values with unlike letters were significantly different (P < 0·005). PC3, human prostate carcinoma cell line.
Xenografts of PC3 prostate adenocarcinoma cells co-injected with transforming growth factor-β-stimulated non-cancer-associated fibroblasts treated with DHA
Consequently, we performed an in vivo experiment in order to clarify the possibility that DHA exposure influences the capacity of TGF-β-stimulated NCAF to promote xenografts of PC3 prostate adenocarcinoma cells. The animals injected with either PC3 cells alone or PC3 cells together with NCAF (data not shown) did not produce subcutaneous tumours, while PC3 cells injected with TGF-β-stimulated NCAF induced tumours with a short latency (15 d) and high incidence (100 %). On the other hand, animals injected with PC3 cells together with 30 μm-DHA-treated TGF-β-stimulated NCAF induced tumours with a significantly reduced growth rate, although incidence and latency were unchanged (Fig. 4). This implies that DHA-treated TGF-β-stimulated NCAF were unable to sustain a proper in vivo growth of prostate cancer cells.

Fig. 4 Tumour growth of PC3 prostate adenocarcinoma cells co-injected with transforming growth factor-β (TGF-β)-stimulated non-cancer-associated fibroblasts (NCAF) or (30 μm) DHA-treated TGF-β-stimulated NCAF. In the inset, α-smooth muscle actin (α-SMA) expression in TGF-β-stimulated NCAF and in DHA-treated TGF-β-stimulated NCAF analysed before co-injection with tumour cells. Comparison between regression coefficients (slopes) was performed by two-tailed Student's t test (n 4; P < 0·02). ◆, PC3 co-injected with TGF-β-NCAF; ■, PC3 co-injected with DHA/TGF-β-NCAF. PC3, human prostate carcinoma cell line. WB, Western blot.
DHA reverses the myofibroblast phenotype of prostate adenocarcinoma-associated fibroblasts
In order to demonstrate that DHA may also have the capacity to revert myofibroblast phenotype, we used CAF, obtained from a tumour region of an adenocarcinoma-bearing patient, confirmed by Western blot to be strongly positive for α-SMA. Since CAF promote PC3 invasiveness through an MMP-dependent pathway, there was no need for in vitro activation by TGF-β to elicit aggressiveness. Treatment of CAF with 30 μm-DHA reversed α-SMA expression, MMP-2 release and PC3 migration through Matrigel, thereby confirming the capacity of DHA not only to inhibit fibroblast-to-myofibroblast differentiation, but also to revert some crucial aspects of the myofibroblast phenotype involved in the progression of prostate cancer cells (Fig. 5).

Fig. 5 Effect of (30 μm) DHA on prostate cancer-associated fibroblasts (CAF). (A) α-Smooth muscle actin (α-SMA) expression in DHA-treated CAF determined by immunofluorescence (IF) and (B) Western blot (WB) and densitometric analysis of three independent experiments (determined as in Fig. 1). a,b Mean values with unlike letters were significantly different (P < 0·005). (C) Zymogram and densitometry analysis of matrix metalloproteinase-2 (MMP-2) released in media conditioned by CAF and DHA-treated CAF of three independent experiments normalised by the number of CAF. a,b Mean values with unlike letters were significantly different (P < 0·002). (D) Invasiveness of PC3 tumour cells exposed for 72 h to media conditioned by CAF or DHA-treated CAF. Values are means of two independent experiments, with their standard errors of the mean represented by vertical bars. a,b,c Mean values with unlike letters were significantly different (P < 0·005). PC3, human prostate carcinoma cell line. CM, conditioned media. A colour version of this figure can be found online at http://www.journals.cambridge.org/bjn
Discussion
A growing body of literature describes the reciprocal influence of CAF and tumour cells in prostate cancer progression( Reference Hill, Song and Cardiff 8 , Reference Giannoni, Bianchini and Masieri 11 , Reference Chung, Baseman and Assikis 13 – Reference Verona, Elkahloun and Yang 15 ). These cellular interactions stably induce genetic and behavioural changes that can contribute to cancer progression, and this may provide a molecular basis for a therapeutic approach to change the natural history of prostate cancer. Thus, combining therapeutic strategies that co-target cancer cells as well as CAF will guide new opportunities to delay the progressive phenotype of prostate cancers.
We tested the hypothesis that DHA, a n-3 PUFA, directly inhibits the tumour-promoting effect on fibroblasts affecting their differentiation into myofibroblasts, and found that it does by blocking the prostate fibroblast MMT and also by reverting the myofibroblast phenotype.
The observation that DHA, unlike other long-chain fatty acids analysed (EPA and AA), blocks the MMT in skin fibroblasts stimulated by TGF-β prompted us to test DHA in our prostate fibroblast experimental model. In fact, DHA repressed myofibroblast transition in prostate NCAF upon their stimulation with TGF-β, considered one of the most potent, either in vitro or in vivo, mediators of the MMT. This is in keeping with the capacity of DHA to inhibit osteoclast differentiation, activation and function( Reference Rahman, Bhattacharya and Fernandes 27 ) and to modulate pro-inflammatory gene expression associated with colon carcinogenesis( Reference Narayanan, Narayanan and Simi 28 ).
We also showed that abrogation of the MMT in NCAF by DHA was associated with a repression in the MMP-mediated enhanced invasiveness of PC3 cells. In parallel experiments, we demonstrated that abrogation of the MMT in prostate NCAF by DHA led to the inhibition of TGF-β-stimulated NCAF, induction of typical properties of the EMT in PC3 cells, such as a mesenchymal morphology, a reduced E-cadherin and an increased c-Met and invasiveness through Matrigel. It is well known that many types of cancer cells secrete molecules that enhance the production of hepatocyte growth factor, a ligand for c-Met receptor tyrosine kinase, by fibroblasts, and fibroblast-derived hepatocyte growth factor, in turn, stimulate in c-Met-expressing tumour cells an increased invasiveness towards the host's tissues. Overexpression of c-Met and increased production of hepatocyte growth factor in tumour tissues have been related to aggressive tumour growth and to a poor therapeutic outcome( Reference Matsumoto and Nakamura 29 ).
Finally, the DHA-treated TGF-β-stimulated NCAF co-injected with PC3 cells produced tumours in immunodeficient mice with a lower growth rate with respect to that of PC3 cells with TGF-β-stimulated NCAF. It is worth noting that this result is not applicable to a DHA dietary intervention in tumour prevention; however, together with in vitro results, it strengthens the concept of the inhibition of prostate myofibroblast differentiation by DHA.
A number of mechanisms might be involved in the DHA inhibition of prostate fibroblast MMT, including the influence on transcription factor activity and gene expression. It is possible that DHA might abrogate the reactive oxygen species-dependent expression of α-SMA and the pro-invasive signals in TGF-β-stimulated prostate fibroblasts. It has been shown that TGF-β increases the reactive oxygen species level in stromal fibroblasts, which initiate the MMT( Reference Cat, Stuhlmann and Steinbrenner 30 ), and DHA enhances the antioxidant response of human fibroblasts by up-regulating γ-glutamyl-cysteinyl ligase and glutathione reductase( Reference Arab, Rossary and Flourié 31 ).
In conclusion, the present findings suggest a new activity of DHA in preventing the pro-tumorigenic effect of prostate adenocarcinoma-associated fibroblasts that may delay the progressive phenotype of prostate cancer cells.
Acknowledgements
This study was supported by grants from the Istituto Toscano Tumori and Fondazione Cassa di Risparmio di Firenze. The authors have no potential personal, commercial, political, academic conflict-of-interest and no financial relationship with any commercial organisation. The authors' responsibilities were as follows: F. B. was involved in the conception and design of the study, performed the research and animal studies, analysed the data and drafted the manuscript; E. G. was involved in performing the research and analysed the data; S. S. helped in performing the research; P. C. helped in the study supervision and provision of significant advice; L. C. designed and supervised the study, and along with F. B. contributed to the final version of the manuscript; all the authors contributed to the data interpretation, critical review and revision of the manuscript.