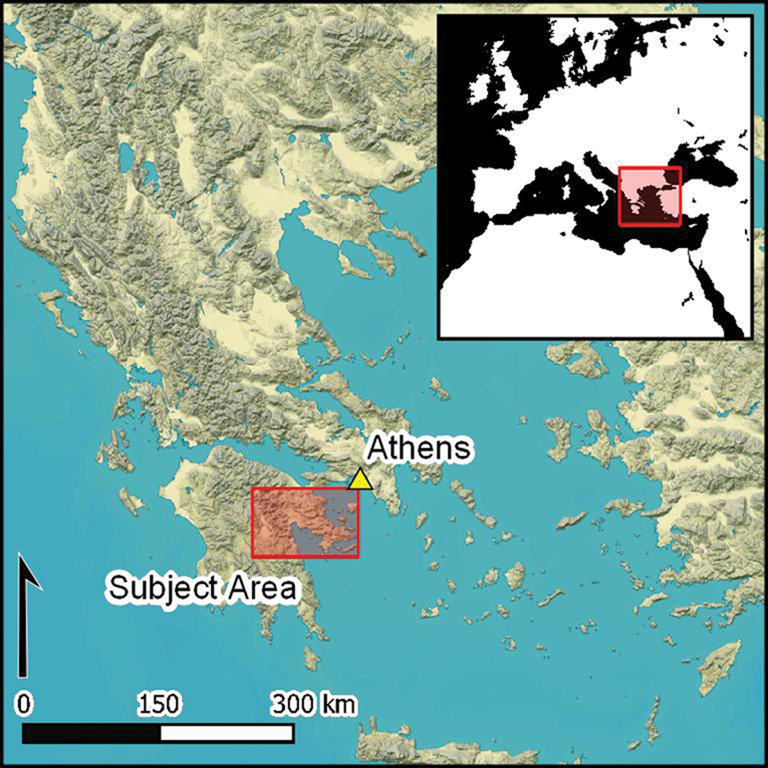
Introduction
The current study discusses the archaeological evidence for changing agricultural land-use patterns in the north-eastern Peloponnese during the Late Hellenistic and Roman periods (c. 150 BC–AD 300), in combination with new, high-resolution palaeoclimatological data. Our aim is to investigate the effects of past climate variability on socio-economic trajectories in a period during which we also observe an increasing external political impact on regional economies. The growing corpus of published archaeological survey data from Greece provides a substantial body of research on rural settlement structures, agricultural economies and landscape dynamics. This research has greatly emphasised the pivotal role played by political agency in shaping the organisation of the countryside during the period of Roman rule (see Alcock Reference Alcock1993). Environmental factors have previously been considered as part of such dynamics; in particular, soil degradation and erosion have been discussed in relation to land-use contraction patterns (e.g. van Andel et al. Reference van Andel, Zangger and Demitrack1990; Bintliff et al. Reference Bintliff, Howard and Snodgrass2007: 180; Fuchs Reference Fuchs2007). A more detailed discussion of the potential impact of climate variability on land use is nevertheless required to understand how human-environment interactions can be more effectively integrated into the narrative of socio-economic development in the Peloponnese during these periods.
Recent studies concerning prehistoric mainland Greece have contributed to an increasingly detailed discussion of climate variability and landscape dynamics, stressing the number of complex forces that shaped human-environment interactions and socio-economic processes on a local level (e.g. Finné et al. Reference Finné, Holmgren, Shen, Hu, Boyd and Stocker2017; Weiberg & Finné Reference Weiberg and Finné2018). Comparatively less research has addressed the potential impact of past climate variability in later periods in Greece. In part, this is because local palaeoclimatological proxy data have only recently become available, but also because the methodologies for integrating palaeoclimatological, archaeological and historical datasets require further development. The results presented here contribute to a broader discussion on how archaeologists can integrate palaeoclimatological data into their research, using the north-eastern Peloponnese as a specific case study. Here, we argue for the use of a contextualised approach integrating relevant datasets, and discuss how past climate variability may have worked in tandem with politically driven socio-economic processes. Specifically, we examine the factors involved in the diachronic reconfiguration of land use as part of minor climate shifts, rather than responses to specific climate ‘events’.
Landscape dynamics in Hellenistic and Roman Greece
Several studies using the results of intensive archaeological surveys have emphasised how Roman rule transformed the rural landscape of the southern Greek mainland. Such change is visible through a reduction in the number of small rural settlements and the development of rural villas, which are interpreted as the centres of larger agricultural estates (e.g. Alcock Reference Alcock1993; Bintliff & Howard Reference Bintliff and Howard1999; Bintliff Reference Bintliff and Grandjean2008, Reference Bintliff, Kerig and Zimmermann2013; Rizakis Reference Rizakis, Rizakis and Touratsoglou2013, Reference Rizakis2014; Stewart Reference Stewart2013). The picture provided by these surveys, however, is not uniform. While reductions in site numbers are evident in certain regions—primarily central Greece and the north-eastern Peloponnese—the picture elsewhere is more varied (Bintliff Reference Bintliff1997; Stewart Reference Stewart2013). In Messenia in the south-western Peloponnese, for example, a broad increase in the number of rural sites during the Hellenistic period was followed by a slight expansion in site numbers in the succeeding Roman imperial period (Alcock et al. Reference Alcock, Berlin, Harrison, Heath, Spencer and Stone2005; Stewart Reference Stewart2013: 71; see also Davis & Bennet Reference Davis and Bennet2017). The high number of rural settlements in Messenia compared to the north-eastern Peloponnese can partly be explained by the previously limited settlement expansion under the territorial and political dominance by Sparta until the fourth century BC. Sparta required Messenian helots (subjugated communities) to be concentrated into nucleated villages (Alcock et al. Reference Alcock, Berlin, Harrison, Heath, Spencer and Stone2005; for a discussion of the exploitation of Messenian helots in the Spartan agrarian economy, see Hodkinson Reference Hodkinson, Luraghi and Alcock2003). The increase in site numbers during the Hellenistic period recorded by the Pylos Regional Archaeological Project should thus be understood in the light of the Messenian liberation, which resulted in new land being allocated to the liberated population, with rural settlement structures being maintained throughout the Hellenistic and possibly into the Roman period (Alcock et al. Reference Alcock, Berlin, Harrison, Heath, Spencer and Stone2005). These differences in socio-political processes fundamentally emphasise the effect on landscape dynamics and varying settlement trajectories between different parts of the Peloponnese.
Changes in land-use strategies during the Late Hellenistic and Roman periods have also been explored through geoarchaeological research in certain areas of the Peloponnese. Changing economic strategies in the Southern Argolid, for example, are argued to have resulted in the abandonment of terraced agriculture and increasing sedimentation during the later Hellenistic period (Runnels & van Andel Reference Runnels and van Andel1987; van Andel et al. Reference van Andel, Zangger and Demitrack1990). Although high rates of sedimentation during the Roman period have also been identified through geoarchaeological work in the Phlious Basin in the north-eastern Peloponnese, this has been attributed to intensified human pressure on the landscape (Fuchs Reference Fuchs2007). While these studies present two different interpretations for sedimentation, both link to the environmental impact of anthropogenic activity and the restructuring of land use. Fuchs (Reference Fuchs2007: 352) discusses the possibility that changes in climate could have caused increasing erosion, but emphasised that the available palaeoclimatological sequence provided insufficient evidence to confirm this hypothesis. Fuchs's (Reference Fuchs2007) discussion of the possible impacts of changing climate, however, was hampered by the use of non-local, Eastern Mediterranean proxy data, which obscure the effects in specific Greek or Peloponnesian contexts. Although it is unlikely that drier conditions directly triggered increasing erosion, climate variability in combination with changing land use could have increased the susceptibility to erosion (Walsh et al. Reference Walsh2019). New palaeoclimatological data from the Peloponnese enable these questions to be further addressed (Finné et al. Reference Finné, Bar-Matthews, Holmgren, Sundqvist, Liakopoulos and Zhang2014, Reference Finné, Holmgren, Shen, Hu, Boyd and Stocker2017; Boyd Reference Boyd2015; Weiberg et al. Reference Weiberg2016).
Speleothem proxy data and climate variability
In the context of the Peloponnese, data on past climate variability come predominantly from proxies recorded in cave speleothems (primarily stalagmites) and lake/wetland sediments (for an overview, see Finné & Weiberg Reference Finné, Weiberg, Ekblom, Isendahl and Lindholm2018). Here, we use information from stalagmites extracted from three different Peloponnesian caves (Figure 1): Mavri Trypa Cave situated off the south-west coast of Messenia; Alepotrypa Cave located on the south-west coast in Laconia; and Kapsia Cave in central Arcadia (Finné et al. Reference Finné, Bar-Matthews, Holmgren, Sundqvist, Liakopoulos and Zhang2014, Reference Finné, Holmgren, Shen, Hu, Boyd and Stocker2017; Boyd Reference Boyd2015; Weiberg et al. Reference Weiberg2016).

Figure 1. Location of the new Peloponnesian speleothem records used for the current study (figure by A. Bonnier & M. Finné).
The stalagmites in question have been dated using uranium-thorium dating; climate information derives from stable oxygen isotope (δ18O) analysis (Finné et al. Reference Finné, Bar-Matthews, Holmgren, Sundqvist, Liakopoulos and Zhang2014, Reference Finné, Holmgren, Shen, Hu, Boyd and Stocker2017; Boyd Reference Boyd2015). As the δ18O signal in stalagmites from the Central and Eastern Mediterranean are often interpreted to reflect moisture variability, we can use the signal to infer changes in the availability of moisture over time (e.g. Bar-Matthews et al. Reference Bar-Matthews, Ayalon and Kaufman1997; Jex et al. Reference Jex, Baker, Fairchild, Eastwood, Leng, Sloane, Thomas and Bekaroğlu2010). Although there are many similarities between the δ18O results from the three caves, which reflect regional trends, there are also differences in relation to the period under consideration here (150 BC–AD 300) (Figure 2).

Figure 2. Comparison of the δ18O records from Kapsia, Mavri Trypa and Alepotrypa. In Mavri Trypa Cave it is likely that the δ18O values appear less negative (i.e. drier) in the period between 120 BC and AD 80 due to sampling issues (Finné et al. Reference Finné, Holmgren, Shen, Hu, Boyd and Stocker2017). Therefore, the δ18O values from Mavri Trypa in this period have been manually adjusted by −0.3 ‰ before the proportion of dry points was calculated. The shaded area highlights the focus period here (figure by A. Bonnier & M. Finné).
The observed differences could be the result of poor chronological matching between the cave records due to dating uncertainties—an issue that is particularly relevant for the Kapsia speleothem (see Finné et al. Reference Finné, Bar-Matthews, Holmgren, Sundqvist, Liakopoulos and Zhang2014; Finné & Weiberg Reference Finné, Weiberg, Ekblom, Isendahl and Lindholm2018). Local, site-specific factors, such as human activity that altered the cave atmosphere (e.g. the use of fire) and the cave's natural bedrock properties, could also explain some of the observed differences between the δ18O data. Variations between the records could, however, relate to actual climatic differences, meaning that in the Late Hellenistic–Roman periods, more than one climate trajectory existed over the Peloponnese, with shorter periods of drought in the south-west compared to the central inland region. Proxy data from earlier periods in the north-eastern and south-western Peloponnese suggest the possible existence of different climate trajectories that were driven by shifting atmospheric circulation patterns, which differentially affected areas of the peninsula (Katrantsiotis et al. Reference Katrantsiotis, Norström, Smittenberg, Finne, Weiberg, Hättestrand, Avramidis and Wastegård2019). Even if the cave records could be understood as providing a single climate signal, however, we still require regional contextualisation of the possible impact of climate variability on agricultural land use.
Precipitation variability in the Peloponnese
Modern rainfall patterns in the Peloponnese show consistent east–west variation in annual precipitation amounts (Figure 3; Hellenic National Meteorological Service 2017–2020). The majority of rain falls between October and April, with potential evapotranspiration at its strongest during the summer months. The geographic distribution of precipitation results from a combination of the peninsula's topography and the dominant west–east transport of moisture. Moist air generally makes first landfall on the western side of the peninsula, where mountains and hills force orographically induced precipitation. Once the air reaches the eastern and north-eastern parts of the Peloponnese, much of its original moisture is lost. These parts of the peninsula are characterised by the driest conditions as measured by the ‘Aridity Index’ of precipitation and potential evapotranspiration (Nastos et al. Reference Nastos, Politi and Kapsomenakis2013).

Figure 3. Annual precipitation (in mm) in the Peloponnese, showing the location of speleothem archives, interpolated using recent data from meteorological stations in and close to the Peloponnese (figure by A. Bonnier & M. Finné).
The modelling of future scenarios emphasises that areas characterised by drier conditions are more vulnerable to further reductions in precipitation (amplified or modulated by possible shifts in potential evapotranspiration) that will cause a decline in the moisture content of soils available for plants (Nastos et al. Reference Nastos, Politi and Kapsomenakis2013). Field research and modelling have demonstrated the effects of reduced amounts of precipitation, including water stress and drought on modern yields of rain-fed winter wheat and barley in Greece (Halstead Reference Halstead, Halstead and O'Shea1989: 72–73; Kosmas et al. Reference Kosmas, Danalatos, Moustakas, Tsatiris, Kallianou and Yassoglou1993; Aschonitis et al. Reference Aschonitis, Lithourgidis, Damalas and Antonopoulos2013; Tigkas & Tsakiris Reference Tigkas and Tsakiris2015). Starting from a lower baseline value for precipitation, the impact of drier conditions would have been stronger in the already drier north-east, where reduced precipitation levels could affect agricultural output directly. A drier period at around AD 50 at the Mavri Trypa and Alepotrypa Caves that lasted for 20 and 65 years, respectively, should—following the above reasoning—have had a stronger impact in the north-east than compared to the more humid southern areas of the peninsula.
Climate variability and land-use dynamics in the north-eastern Peloponnese
We focus our study of land-use dynamics on the north-eastern Peloponnese due to the potentially greater impact of arid conditions on agriculture in this region. In order to examine land-use dynamics in combination with the available palaeoclimatic evidence, we use GIS-based kernel density estimation (KDE), which allows us to quantify shifts in land-use patterns beyond a reliance on changes in site numbers. The premise of the KDE analysis is to define the possible extent of land use in different periods by modelling density surfaces based on the location of sites recorded by archaeological surveys (Bonnier et al. Reference Bonnier, Finné and Weiberg2019). The density surfaces produced through KDE analyses are presented as heat maps, which provide a means of visualising expansion and contraction patterns of hypothetical land use within the specific survey areas, and can be used to approximate the spatial configuration of agricultural land use in different periods. We base our analyses on digitised site data from five archaeological surveys (Figure 4). Using a 2.5km radius analysis for each dataset, representing an idealised catchment zone for Greek agricultural settlements (see Bintliff Reference Bintliff2012: 271), we created a three-tiered kernel division representing a maximum extent of possible land use, a medium extent and a minimum extent, the latter of which shows only the high density of land use (following the method presented in Bonnier et al. Reference Bonnier, Finné and Weiberg2019). The results of the individual analyses carried out for each dataset were then merged to provide an aggregated quantification of trends in land-use expansion and contraction.

Figure 4. Location of surveys in the north-eastern Peloponnese used for the GIS-based kernel density estimate analysis: the Southern Argolid Survey (Jameson et al. Reference Jameson, Runnels, van Andel and Munn1994); the Methana survey (Mee & Forbes Reference Mee and Forbes1997); the Berbati-Limnes survey (Wells & Runnels Reference Wells and Runnels1996); the Nemea Valley Archaeological Project (Wright et al. Reference Wright, Cherry, Davis, Mantzourani, Sutton and Sutton1990); and the Phlious Valley survey (Casselmann & Maran Reference Casselmann and Maran2004) (figure by A. Bonnier & M. Finné).
Due to differences in the resolution of the ceramics-based chronologies used by the different survey projects, we grouped the site data for each dataset into two broad time frames, namely Classical–Hellenistic (480–150 BC) and Late Hellenistic–Roman (150 BC–AD 300). These chronological divisions have been used in order to accommodate roughly the chronologies employed by the different surveys, where ceramic assemblages are often reported to span several relative periods. The two time frames used here therefore represent a reasonable division of visible aggregate site trends, even though more detailed chronologies have been provided by some survey projects. The chronological break between these two periods also coincides with the historical context of increasing Roman influence in the Peloponnese, following the defeat of the Achaean League in 146 BC. For the current analysis, the different sites were assigned to one of the two time frames based on the ceramic chronologies provided by the original surveys (Figure 5). Sites of insecure identification or imprecise date ranges are excluded from the analysis.

Figure 5. Number of sites used for the GIS-based kernel density estimate (figure by A. Bonnier & M. Finné).
Climate variability and changes in the extent of possible land use
The δ18O signal is usually presented against a modelled age-scale using an absolute chronology that can be effectively compared with historical events. This method, however, presents problems when addressing evidence relating to medium- and long-term landscape dynamics. As survey data are frequently structured according to relative ceramic phases, it is often impossible to determine whether all sites included in the specific phases were operating contemporaneously in the landscape. We should therefore view the site data as an aggregate per period, which can be more appropriately compared with the palaeoclimate data if the latter are organised in the same way. Thus, we aggregated the comparatively high-resolution climate data from the three speleothems to fit the two time frames of the survey data (similar to Ingram Reference Ingram, Sulas and Pikirayi2018).
The number of stable isotope measurements that report a value below the long-term average proxy value in each individual stalagmite is considered to indicate a drier climate. The proportion of measurement points below the average in each time frame was calculated and considered a proxy for drier climate conditions. This process reduces the resolution of the climate data to fit the survey data, in that it is adjusted to fit the least resolved dataset. Mean δ18O values for the Late Hellenistic–Roman time frame also indicate drier conditions, thus corroborating the results. A Peloponnese-wide climate picture is represented by averaging the proportions of dry points from all three stalagmites, highlighting more arid phases, which can be compared with trends in ceramic chronologies derived from the archaeological surveys (Figure 6). Generally, there is little palaeoclimatic evidence for any major changes in the hydro-climate of the Central and Eastern Mediterranean during the Classical–Hellenistic and Late Hellenistic–Roman time frames. Palaeoclimate proxy data from the Balkan Peninsula and the Aegean Sea, for example, suggest that the Late Hellenistic–Roman period sustained overall wetter conditions, although a small shift towards drier conditions occurred c. 150 BC (Finné et al. Reference Finné, Woodbridge, Labuhn and Roberts2019).

Figure 6. Proportion of dry points in all cave records in each archaeological time frame. Due to the uncertainties introduced by the manual adjustment of the δ18O values in Mavri Trypa between 120 BC and AD 80, the average proportion of dry points excluding Mavri Trypa Caves is also presented (right) (figure by A. Bonnier & M. Finné).
Using a combination of survey data and palaeoclimate records, it is possible to highlight trends of expansion and contraction in land use related to specific climate conditions. Here, it is important to emphasise that substantial expansion of possible land-use areas in the north-eastern Peloponnese during the Classical–Hellenistic time frame occurred when the climate was generally wetter (Figure 7). The subsequent contraction of land-use areas in the north-east Peloponnese is evident in the Late Hellenistic–Roman time frame, when records suggest that the peninsula experienced drier conditions. Thus, there is synchronic correspondence between trends observed in the palaeoclimatic and archaeological records, in terms of the extent of land use.

Figure 7. Extent of possible land use at the different density levels compared with the average amount of dry points per relative period (based on the three speleothem records).
Climate and topographic variability
The density surfaces produced by KDE analyses further allow us to identify topographical variability of the potential land-use areas, which can then be used to quantify changes in the use of specific topographies over time (Bonnier et al. Reference Bonnier, Finné and Weiberg2019). For the current study, we have used a high-resolution (5m) DEM, through which we have created a slope raster, which we have reclassified according to the threefold division previously described by Whitelaw (Reference Whitelaw, Francovich, Patterson and Barker2000: 234), and categorised according to the agricultural potential based on the gradient factor of the slope (Figure 8). Studies of modern rain-fed wheat cultivation have shown that deeper soils on lower gradient land have a better water-storage capacity and are more fertile compared to thinner soils in steeper areas (e.g. Kosmas et al. Reference Kosmas, Danalatos, Moustakas, Tsatiris, Kallianou and Yassoglou1993: 341 & 344). In drier periods, the use of sloping ground may therefore have proven increasingly problematic, particularly in terms of cereal cultivation. The realignment of land use towards deeper soils may therefore serve as an indicator of a response towards drier climate conditions.

Figure 8. Extent and proportion of slope categories in the north-eastern datasets (defined using Whitelaw Reference Whitelaw, Francovich, Patterson and Barker2000: 234) (figure by A. Bonnier & M. Finné).
In all datasets, low-gradient land (slope class 1) always forms a high proportion of the KDE surfaces, together with the steepest gradient category (slope class 3), although proportional differences between these categories shift between periods (Figure 8). As class 3 probably includes a substantial amount of non-agricultural land, such as steep limestone outcrops, this class is less useful for our current analysis. In terms of understanding land-use dynamics in high-gradient areas, which are potentially less productive due to thinner soil cover, it is more appropriate to focus on changes in the proportion of intermediate-gradient land (slope class 2) within the KDE surfaces. This comprises land that could have been cultivated without terracing, but with a severe risk of soil erosion (Whitelaw Reference Whitelaw, Francovich, Patterson and Barker2000: 234). Here, we observe a higher proportion of class 2 land during the wetter Classical–Hellenistic time frame, with a sharp reduction during the drier Late Hellenistic–Roman time frame.
The KDE approach allows us to identify specific areas of land that were in use during one time frame, but then abandoned in the next. The identification of such areas within the KDE model further allows us to define the slope composition of abandoned land. Extensive abandonment occurred in the Late Hellenistic–Roman time frame and the slope composition of these areas shows the abandonment of a higher proportion of class 2 land during this period, compared with the preceding Classical–Hellenistic time frame (Figure 9). The extent and slope composition of abandoned land thus emphasise a pattern of contraction away from high-gradient land in the drier Late Hellenistic–Roman time frame.

Figure 9. Extent and proportion of slope categories in abandoned areas. For an explanation of slope categories, see Figure 8 (figure by A. Bonnier & M. Finné).
Discussion
The combined palaeoclimate and archaeological data suggest that general land-use contraction and, in particular, the abandonment of high-gradient areas in the north-east Peloponnese occurred during a period of increasingly dry conditions that could have had a negative effect on agricultural yields. It is therefore likely that a drier climate during the Late Hellenistic–Roman time frame would have lowered agricultural output and potentially threatened the stability of thinner soils situated on medium- and high-gradient land, thereby limiting the usability of steeper areas (see Kosmas et al. Reference Kosmas, Danalatos, Moustakas, Tsatiris, Kallianou and Yassoglou1993). Our results for the north-eastern Peloponnese provide a suitable point of departure for discussing how climate variability may have worked in tandem with socio-political developments to influence changes in the spatial configuration of land use.
In the Classical–Hellenistic time frame, much of the cultivated land in Greece, and particularly in the north-eastern Peloponnese, belonged to farms established as part of specific polis-territories. While inequalities in the size of landholdings certainly existed, archaeological survey highlights a significant presence of sites interpreted as small, family farms aimed at both subsistence and limited cash-cropping (for a general discussion on the nature of family farms in Greek survey assemblages, see Winther-Jacobsen Reference Winther-Jacobsen2010: 269–73; Bintliff Reference Bintliff2012: 277–81). The growth of such citizen groups would eventually have necessitated the expansion into high-gradient areas due to the limited availability of flatter land in the north-eastern Peloponnese. Here, this process can therefore be understood as the result of socio-political developments in the region. Increasingly wet conditions in the Classical–Hellenistic time frame, however, would also have made such land-use expansion successful due to the improved agricultural potential of the thinner soils located on sloping ground.
Bintliff and colleagues (Reference Bintliff, Howard and Snodgrass2007) have argued that in Classical–Early Hellenistic Boiotia, in central Greece, rural expansion resulted in soil degradation that negatively affected agricultural yields, leading to a strategy of intensive manuring. It is tempting to argue for similar developments in the north-eastern Peloponnese, even if we lack the detailed off-site survey data to examine such processes there. Climate shifts following extended periods of overuse and soil degradation caused by increasing population pressure may nonetheless have accelerated or enhanced land-use contraction processes. Stress on agricultural production systems and supply issues due to intermittent dry years was already present in the generally wetter Classical–Early Hellenistic. Epigraphic evidence, for example, emphasises the transfer of grain to Peloponnesian communities in the fourth century BC (see, for example, Bresson Reference Bresson, Archibald, Davies and Gabrielsen2011), highlighting food-security issues connected with single drought years. A drier climate during the Late Hellenistic–Roman time frame would probably have increased the occurrence of such dry years, potentially destabilising agricultural production linked to estates and family farms and fields situated on sloping ground. Thus, increasing aridity would have contributed to a reorganisation of land-use strategies in the region, particularly when combined with other political and ecological processes.
Ancient written sources suggest various stages of landscape reorganisation in Greece during the Hellenistic and Roman periods. A third-century BC increase in economic inequalities and the growth of larger estates that absorbed smaller landholdings can be observed historically and archaeologically, even though older landownership structures were also maintained (Shipley Reference Shipley2005). The increasing appropriation and redistribution of land seem more specifically to have occurred in the later first century BC onwards—coinciding with the formal establishment of the Roman province of Achaea in 27 BC (Rizakis Reference Rizakis, Rizakis and Touratsoglou2013: 23). Literary and epigraphic sources further provide evidence for the increased presence of commercial agents, such as negotiatores and freedmen, as well as other individuals who seem to have actively invested in agricultural production throughout the province of Achaea, from the early imperial period and onwards (Bintliff Reference Bintliff, Kerig and Zimmermann2013; Rizakis Reference Rizakis, Rizakis and Touratsoglou2013, Reference Rizakis2014; Zoumbaki Reference Zoumbaki, Rizakis and Touratsoglou2013; Kay Reference Kay2014: 139–40).
Investment in agriculture would, to a large extent, have been influenced by market opportunities and cash-cropping strategies, as well as external imperial forces such as taxation that could have stimulated active investment in the production of cash-crops (including bread-wheat) to generate income in order to meet tax requirements (for taxation in Roman Greece, see Alcock Reference Alcock1993: 19–24). In the north-eastern Peloponnese during the Late Hellenistic–Roman period, the production of cereals by family farms would have been restricted by the limited availability of low-gradient soils with a higher water table (i.e. more fertile soils). Small-scale producers operating on sloping ground may therefore have been increasingly challenged by the shifts towards a drier climate, as well as demands set by new imperial market systems.
The results of our KDE analyses suggest a high degree of spatial diversification and high-gradient land use in the Classical–Hellenistic time frame, as compared to the Late Hellenistic–Roman period, when contraction was coupled with a proportional decrease in slope class 2 (see Figures 8–9). The data therefore seem to indicate a move towards more focused strategies of arable agriculture in the later period. Marston (Reference Marston2011) has observed similar processes outside the Peloponnese, at Gordion, where changes in agricultural strategies in the Roman period were linked to increased cultivation of bread-wheats for the urban market, supported by the use of irrigation. Due to a general lack of archaeobotanical data, evidence for changes in the proportion of specific crops is not available for the Roman Peloponnese. The contraction of land-use areas and, in particular, the proportional decrease of slope class 2 may, however, suggest a realignment of production strategies in the north-eastern Peloponnese similar to those suggested for Gordion.
Socio-political developments could therefore be used to explain the shifts in land-use patterns between the two time frames, even without the palaeoclimatological evidence for dry periods. When adding an increasingly dry climate during the Late Hellenistic–Roman time frame to this scenario, however, it seems probable that continued agricultural investment in high-gradient land in the north-east would have been both unsustainable for small, family farms and unattractive for new, elite landowners. The data provided by both the KDE analyses and the palaeoclimatological records can therefore help to explain differences in land use between the two time frames—especially when integrated with the evidence for the restructuring of landownership and changing socio-economic trajectories.
Conclusion
Our multi-stranded study emphasises the importance of adopting a regional perspective in order to contextualise past climate variability and its potential impact on agricultural strategies in environmentally diverse regions. The integrated analyses of climate variability and land-use dynamics presented in the current study highlight the potential role of such variability in the spatial organisation of agricultural land-use strategies.
In the context of the north-eastern Peloponnese, the shifts in climate should not be understood as a triggering factor or primary driver behind landscape change. The wetter climate observed in the Classical–Hellenistic time frame, however, could, potentially, have pushed land-use expansion to its maximum extent and capacity, including the use of higher-gradient landscapes. The drier conditions during the Late Hellenistic–Roman time frame may have influenced a realignment of agricultural production strategies away from sloping ground. The analytical approach presented here highlights the potential within landscape archaeology for examining climate impact on landscape dynamics beyond cataclysmic shifts in socio-economic structures linked to specific climate events. Our research further demonstrates the importance of investigating human-environmental interactions, and the effects of climate variability in particular, on the regional and even micro-regional levels.
Acknowledgements
We are very grateful to Jack Davies, Jim Wright and Christian Cloke for providing unpublished data from the ‘Nemea Valley Archaeology Project’. We also thank two anonymous reviewers for offering comments that improved the quality of the manuscript. Erika Weiberg provided several useful comments on a draft version of the manuscript.
Funding statement
The authors acknowledge funding from the Swedish Research Council (grant 421-2014-1181) provided as part of the ‘Domesticated Landscapes of the Peloponnese Project’.