Introduction
Qinghai-Xizang (Tibetan) Plateau is located in middle-to lower-latitude regions with a mean elevation about 4000–4500 m a.s.l. Because of its geographical location and huge size it is a region that is extremely sensitive to changes in glaciers, lake levels, and permafrost, and thus can provide critical information about changes in climate and environment. Therefore, over the last ten years, many international cooperative investigations have been carried out on the plateau. Access to the west Kunlun mountains, however, is very difficult, and few field studies are available. Published information on the palaeoenvironmental history of the area is relatively scarce (for example, Reference Li, Qingsong and FubaoLi Jijun and others, 1986; Reference Li, Benxing and KeqinZheng, 1987; Reference Li, Benxing and XijinLi Shijie and others, 1989; Reference Li, Qingsong and FubaoLi Bingyuan and others, 1991).
This paper, based on field investigations and laboratory analyses of samples, provides some new evidence for glacier variations and lake changes since 45 000 a BP and uses this evidence as a basis for reconstructing palaeoclimatic and palaeoenvironmental changes.
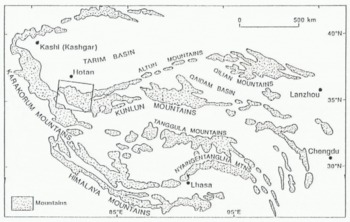
Fig. 1. Location of the west Kunlun mountains. Boxed region is enlarged in Figure 2.
Regional Characteristics
The west Kunlun mountains are a huge interior mountain range on the northwest margin of the Qinghai-Xizang Plateau (Fig. 1). They face the Tarim basin to the north and are adjacent to the Karakoram mountains to the west, and to a vast highland stretching to the south, with many peaks over 6000 m a.s.l. The highest peak in the range is Kunlun Peak, 7167 m a.s.l. (latitude 35°19′N, longitude 80°55′E).
The climate is very cold and variable, owing to the high altitude, with a mean annual temperature below −5°C. Thus, it is an area with one of the highest concentrations of existing glaciers in the world. According to statistical data of the Lanzhou Institute of Glaciology and Geocryology (1988), there are 4306 glaciers occupying a combined area of 8438 km2, of which 244 glaciers occupy 1347.67 km2 in the area along the south slope from Tianshuihai to Keriya Pass (Fig. 2). Most are valley glaciers, but some larger ice caps exist on the flat-topped summits. The glacial tongues generally reach to the mountain foot, about 4900–5300 m a.s.l. and the elevation of the modern snowline is about 5900–6100 m a.s.l.
Modern lakes lie mainly in tectonically depressed basins with an east–west orientation. From west to east, these are: north Tianshuihai, Tianshuihai, Aksayqin, Guozha, and Bangda Lakes (Fig. 2). Basic characteristics of these lakes are listed in Table 1. The water source is mainly glacial meltwater. In the summer season, torrential meltwater streams flow into the lakes, causing them to rise. For example, the water level of Guozha Lake rose 0.14 m from 19 July to 28 August 1987, measured by the Sino-Japanese Joint Expedition using a fluviograph.

Fig. 2. Glacier distribution and location of lakes on the south slope of the west Kunlun mountains.
This required a minimum of 3416 × 104 m3 water, ignoring evaporation.
Geological Evidence For Glacial Variations
Glacial deposits
Moraine systems can indicate glacial changes directly and record climatic fluctuations indirectly. But, in western China, it is more difficult to determine the ages of moraines because the till is composed mainly of boulders, sand and silt. Fortunately, stratigraphic sequences consisting of alternating till and lacustrine deposits, the latter composed of dark-grey clay containing macrofossils of aquatic grasses, have been discovered on the south slope of the west Kunlun mountains. Radiocarbon dates have been obtained from key sections. The following discussion is based on this chronology.
Table 1. Characteristics of present-day lakes on the southern slope of the west Kunlun mountains


Fig. 3. Map of late Quaternary and Holocene deposits in the area of Chongce Glacier.
In front of the Chongce and East Chongce Glaciers (“1” in Fig. 2), north of Guozha Lake, there is a series of moraine ridges. Based on the stratigraphic sequence and chronology, they can be divided into several stages as shown in Figure 3. The first moraine, the LGM deposit, is arcuate in shape and lies about 6.5 km from the end of the Chongce Glacier. It has been eroded extensively by subsequent meltwater, and many boulders between 0.8 and 1.0 m in diameter are scattered on its surface. A 3 to 5 m thick outcrop of dark-grey lacustrine clay has been found beneath the moraine. At 1 m depth, the 14C date is 30 935 ± 1700 a BP. In addition, another exposure of lacustrine clay was also discovered between the first and the second moraine. Its 14C date is 14930 ± 370 a BP (Fig. 3b).
The second moraine, of Early Holocene age, is located about 3.5 km from the end of the glacier. It is composed of boulders, gravels, sand and silt. Streams have cut it into several separate hills with relief of about 5 m, but the hills still surround the glacier tongue in an arcuate shape. On the surface, a thin soil layer has developed, and grasses grow on it. This moraine has not been dated, but an end moraine of similar character has been found in front of the Keriya Glacier (“2” in Fig. 2) at the Keriya Pass (Fig. 4). The latter’s 14C age is 8287 ± 160 a BP. Beneath this moraine, there are dark-grey lacustrine clay deposits with microlaminations. The 14C age of organic material near the top of the clay is 15 550 ± 150 a BP and at 1 m depth it is 21 995 ± 600 a BP. Some patches of dark-grey clay with l4C dates of 15 790 ± 384, 17 180 ± 425 and 18 140 ± 319 a BP were incorporated into the moraines formed by later glacier advances (Fig. 4). A synchronous end moraine, with a 14C date, 8455 ± 265 a BP, has also been found at the Dunde Ice Cap in the Qilian mountains. This ice advance in China appears to be coincident with a cold period in the Early Holocene suggested by Reference Denton and Karlén.Denton and Karlén (1973).

Fig. 4. Longitudinal profile through deposits at the end of Keriya Glacier. The 15–18 ka dates from inner moraines are from older lacustrine material incorporated into moraines.
The third and the fourth moraines of Chongce Glacier are neoglacial deposits, nearly connected with each other, about 1.2 km from the end of the glacier (Fig. 3). Distal parts have been destroyed by outwash. The well-preserved lateral moraine ridges are about 5–8 m high and 30–50 m wide. Some grass has grown in patches where a thin soil has developed on the fine material. The l4C ages are 3983 ± 120 a BP and 3522 ± 117 a BP, respectively. In the Tien Shan mountains, northwest China, a similar end moraine of about 4080 ± 150 a BP 14C age has also been found (Reference ChenChen Jiyang, 1987). Interestingly, this is consistent with the dramatic demise of pine in Scotland at about 4000 a BP (Reference BennettBennett, 1984; Reference Bridge, Haggart and LoweBridge and others, 1990). This may suggest that climate deterioration was contemporaneous in the northern hemisphere at that time.
The last three moraines represent the Little Ice Age and are about 1.2 km from the existing glacier terminus. Grasses have barely colonized these moraines, in distinct contrast with those formed earlier. This pattern is general along the termini of existing glaciers in west China. It appears to be identical with the results of glacial fluctuations during the Little Ice Age all over the world (Reference GroveGrove, 1988).
Changes of glacier length and equilibrium line altitude (ELA)
From the locations of moraine ridges, length changes of Chongce Glacier since the LGM can be reconstructed (Table 2). During the LGM the glacier was only 23% longer than at present. Based both on moraine position and accumulation area ratios, the estimated maximum ELA lowering during the LGM was about 300 ± 50 m. This is similar to the ELA lowering during the LGM (≤ 400 ± 100 m) in the Rongbuk valley on the northern flank of Mount Everest (Reference Burbank and JianchengBurbank and Kang, 1991), but strongly contradicts Kuhle’s model (Reference Kuhle1985, Reference Kuhle1987) for ice-sheet growth across the Tibetan Plateau.
There are two possible reasons for the unusually slight recession of glaciers in this area during the post-glacial period. (1) The rapid uplift of the Tibetan Plateau may have greatly reduced the post-glacial climate warming and glacier retreat, while, at the same time, the large-scale uplift of the peripheral mountains reduced the precipitation increase. (2) The conservative behaviour of glaciers of the extreme continental type, especially the low ice temperature and velocity as well as the low level of mass balance, results in smaller glacier variations.
Stratigraphic Record of Lake Changes
The evolution of the lakes on the southern slope of the west Kunlun mountains is a cumulative result of both the uplift of the Qinghai-Xizang (Tibetan) Plateau and global climate change. Intensive uplift of the plateau during the Quaternary has produced a barrier, obstructing transport of water vapour carried by the south Asia monsoon into the interior, and leading to a gradually drier climate. Meanwhile, this process has been super-imposed on a background of global climatic change, which has resulted in regional climate changes similar to those of other places in the world, except for their amplitude.
Table 2. Length, of Chongce Glacier at different times since the LGM

Ρ = present.
Based on a vast area of lacustrine deposits, high lakeside terraces and a few age estimates, there was a large lake, termed palaeo-Tianshuihai Lake, in the foreland basin of the south slope of the west Kunlun mountains in earlier times. It was approximately 3000 km2 in area, whereas the total area of present-day lakes is only 410 km2, or ~ 14% of the ancient lake area. By the Late Pleistocene, the palaeo-Tianshuihai Lake had shrunk, and the original water-filled basin changed into a vast lacustrine plain. This event is consistent with the history of most lakes on the Qinghai-Xizang Plateau (Reference Chen, Shaoxiu and XiyuChen Kezao and others, 1981). This is also demonstrated by two cores drilled, respectively, on a high terrace and at the present-day lakeside in the Tianshuihai area. One, SI1, was 15 m deep and the other, SI2, was 9.5m deep (Fig. 5a, b). Core SI1, from the high terrace, is wholly composed of dark-grey lacustrine clay representing a stable lake sedimentary environment. A 14C date of bulk sediment near the top is about 46 850 ± 2970 a BP, measured by the scintillation count method. The normal count time was doubled to obtain sufficient data to calculate the age. Thus, this age is probably unreliable. But there is no doubt that the core consists of older sediments.

Fig. 5. Stratigraphic profiles of lacustrine deposits on the southern slope of the west Kunlun mountains.
The stratigraphic changes in core SI2, from the sand–gravel layer at the bottom to a sand layer, thence to a dark-grey clay layer, thence to a sand-gravel layer, and finally to a dark-grey clay layer at the top, are interpreted to be sedimentary facies changes resulting from lake-level variations. The 14C age of organic material in the lower dark-grey clay layer is about 36 750 ± 1320 a BP, and those of the upper clay layer are 18 720 ± 155 a BP and 16 210 ± 195a BP (Fig. 5b). There is also a layer of lacustrine clay in the high terrace section on the north shore of Aksayqin Lake. 14C dating of organic material in it gives an age of 34 735 ± 820 a BP. Based on the facies, these dates represent high stands of the lake, whereas the intervening sand and gravel layer represents a low stand.
Around all lakes, terraces are well developed and contain macrofossils of aquatic grasses which can be dated. In the first terrace section composed of lacustrine clay deposits around Aksayqin Lake, about 3 to 4 m higher than present-day lake level, 14C dates of 18 520 ± 305 a BP at 1.25 m depth and 16 235 ± 120 a BP at the top have been obtained (Fig. 5, profile c). In a section of the first terrace around Bangda Lake, consisting of alternate layers of dark-grey clay and fine-grained yellow sand, we obtained 14C dates of 15 900 ± 120 a BP and 15 880 ± 115 a BP (Fig. 5, profile d). There are also several well-developed lakeside terraces around north Tianshuihai Lake. The sedimentary section from the lakeside terrace to the basin bottom consists of sand and gravels of near-shore facies, sand and silt of shallow-water facies, and clay of deep-water facies (Fig. 6). 14C dates on bulk sediments gave age estimates of 17 480 ± 155 a BP and 17 700 ± 175 a BP in the upper terrace, and 17 360 ± 180 a BP in the lower one. These dates suggest that the terrace, about 40 m higher than present-day lake level, was formed during the period of the LGM, while the others formed subsequently as the lake was shrinking.

Fig. 6. Profile across lakeside terrace on north Tianshuihai Lake.
Discussion
(1) Glacier advances and retreats depend mainly on mass-balance changes resulting from climatic change. Under the extreme continental conditions in the west Kunlun mountains, glacier variations are more sensitive to precipitation changes than to temperature changes because of the very cold average temperatures at these high altitudes. Thus, the period of glacier advance at the beginning of the LGM might indicate colder, wetter conditions, while glacier retreat during the late glacial suggests a dry climate. During the transition period, glacier stability could represent cold and dry climatic conditions. Moraine deposition should occur during periods of glacier stability and/or retreat under cold conditions.
Based on the record of glacial sediments during the LGM in the west Kunlun mountains, we can divide the glaciation into three main episodes: (i) glacier expansion, 23 000 to 20 000 a BP; (ii) glacier stability, about 20 000 to 16 000 a BP and (iii) glacier retreat, from 16 000 a BP.
Regarding the glacier advance proposed for Early Holocene, our results represent only initial Chinese discoveries at a few sites. More detailed work is required to confirm it.
Following the climate optimum in the Middle Holocene, glaciers advanced in the west Kunlun mountains at about 4000 a BP. This may be regarded as the beginning of a so-called neoglaciation.
(2) Lake-level changes in the west Kunlun mountains are closely related to both climate and glaciofluvial activity. Because most of these lake basins are closed hydrologic systems, their water balance depends on the balance between the amount of precipitation and meltwater influx, and the amount of evaporation. Lake-level changes can be identified by changes in sedimentary facies. Based on lacustrine stratigraphic records, there are two main periods of lake expansion during the last 45 000 years. One was 40 000 to 30 000 a BP, which is coincident with the warmer and wetter climate during the last interstadial. The level of Tianshuihai Lake during this stage was 60 m higher than at present (Li Bingyuan and others, 1991). During this period, Qarhan salt lake in the Qaidam basin, one of the driest closed basins in China, contained fresh water and was much larger than at present (Reference Kezao and BowlerChen Kezao and Bowler, 1987). Another period of lake expansion occurred within the LGM. The level of north Tianshuikai Lake was then 40 m higher than at present (Reference Li, Benxing and XijinLi Shijie and others, 1989). This is correlated with the high lake-level stage of 22 500 to 20 000 a BP throughout China suggested by Reference FangFang (1991).
The causes for the lake-level rises in the different stages are not the same. During the last interstadial, lake-level rise resulted from the warm and wet climate, while the lake-level rise in the earlier stage of the LGM, being synchronous with glacial expansion, indicates a cold and wetter climate. This was a period when a great quantity of water was transferred from the sea to the continents and stored in the form of ice. However, the maintenance of a high lake level later in the stage, when the climate was warmer and drier, could result directly from the amount of meltwater flowing into the lake basins exceeding the amount of evaporation.

Fig. 7. Relationships among the glacial, lake, and climatic changes on the south slope of the west Kunlun mountains.
(3) Based on the discussion above, we summarize the relationship among the glacier, lake-level and climate changes in the west Kunlun mountains in Figure 7. Because Kuhle’s estimates of the equilibrium line altitude depression and glacier geometries are completely irreconcilable with the results from this area, we suggest that his unified ice-sheet model for the Tibetan Plateau is not viable.
Acknowledgements
Many thanks to the Sino-Japanese Joint Glacial Expedition to the west Kunlun mountains, 1987, for offering us an opportunity to do the field investigation. The authors thank especially Dr R. LeB. Hooke and two anonymous reviewers for their very helpful suggestions for improving the manuscript and for their careful revision of the English text. Thanks also to Mr Zhang Yutian and Mr Cao Jixiu in the 14C Laboratory of the Geography Department of Lanzhou University, and Mr Gu Gonshu in the 14C Laboratory of Lanzhou Institute of Glaciology and Geocryology, for dating samples, and to Mr J. Jacyno for drafting.