Implications
Monitoring unconsciousness at slaughter is mandatory by European legislation and is often determined by the absence of behavioural indicators (i.e. loss of posture), physical signs (i.e. rhythmic breathing) and reflexes. Previous research in sheep has indicated that the absence of certain reflexes at slaughter is not a reliable indicator of unconsciousness. The present study showed that absence of the withdrawal- and threat reflex did not indicate unconsciousness in veal calves subjected to non-stunned slaughter and are therefore not recommended for assessment of unconsciousness. Absence of the eyelid- and corneal reflex, on the other hand, reflected unconsciousness well and are considered acceptable for assessment of unconsciousness during non-stunned slaughter.
Introduction
Animals are stunned before slaughter to minimize pain and distress as required by European law (Council Regulation (EC) No 1099/2009, 2009). Stunning, however, is not always compatible with specific methods of slaughter prescribed by religious rites and is legally determined not to be mandatory in animals subjected to this type of slaughter (Council Regulation (EC) No 1099/2009, 2009). Stunning after neck cutting is practiced in some European countries in preference to no stunning at all to avoid potential animal welfare problems (Farouk, Reference Farouk2013). Assessment of unconsciousness is a legal requirement during the slaughter process in Europe irrespective of the method used (Council Regulation (EC) No 1099/2009, 2009). During non-stunned slaughter, animals are restrained and bled by a transverse incision of the neck, severing the skin, muscles, trachea, oesophagus, carotid arteries, jugular veins and major nerves. The severe decrease in cerebral blood flow is intended to lead to a rapid onset of disorganized brain function and unconsciousness (Mellor et al., Reference Mellor, Gibson and Johnson2009). In comparison to sheep, consciousness after the neck cut is prolonged in cattle, because the vertebral arteries, which are not severed by the neck cut, continue to supply blood to the circle of Willis and provide some blood to the brain (Baldwin and Bell, Reference Baldwin and Bell1963). There is considerable variation between cattle in time to loss of consciousness after the neck cut. Some studies suggest a rapid loss of consciousness (4 to 7 s) in cattle after the neck cut (Nangeroni and Kennett, Reference Nangeroni and Kennett1963; Bager et al., Reference Bager, Braggins, Devine, Graafhuis, Mellor, Tavener and Upsdell1992) whereas other studies report a spontaneous loss of brain activity 75±48 s after the neck cut (range 19 to 113 s) and the possibility of an intermittent return to consciousness for up to 123 to 323 s after slaughter (Newhook and Blackmore, Reference Newhook and Blackmore1982; Daly et al., Reference Daly, Kallweit and Ellendorf1988). There is substantial debate about which indicators most adequately assess unconsciousness at slaughter and which merit further investigation (European Food and Safety Authority (EFSA), 2013). The use of recorded brain activity (as presented in an electroencephalogram (EEG)) is considered to be the most objective method available for assessing unconsciousness and is generally accepted as the current ‘gold standard’ (Erasmus et al., Reference Erasmus, Turner and Widowski2010; EFSA, 2013; Verhoeven et al., Reference Verhoeven, Gerritzen, Hellebrekers and Kemp2015a). Due to the complexity of collecting EEG data at slaughter, absence of behavioural indicators (i.e. loss of posture) physical signs (i.e. rhythmic breathing) and reflexes (i.e. righting-, threat-, withdrawal-, corneal- and eyelid reflex) is often used to assess unconsciousness. However, there is a lack of scientific publications reporting relationships between unconsciousness ascertained by EEG recordings with those assessed using behavioural indicators, physical signs or reflexes that could be used in slaughterhouse conditions in bovines. It has been formally agreed in the Netherlands that animals subjected to non-stunned slaughter should be unconscious within 40 s, based on absence of at least three of the following five indicators: (1) threat reflex; (2) withdrawal reflex; (3) corneal reflex; (4) (spontaneous) eyelid reflex; (5) rhythmic breathing (Staatscourant, 2012). Different studies showed that the corneal- and eyelid reflex and rhythmic breathing were lost long after animals were considered unconscious based on EEG recordings, making them distinctly conservative indicators when assessing unconsciousness in non-stunned slaughtered sheep (Verhoeven et al., Reference Verhoeven, Gerritzen, Kluivers-Poodt, Hellebrekers and Kemp2015b) and veal calves (Lambooij et al., Reference Lambooij, van der Werf, Reimert and Hindle2012). On the contrary, both threat- and withdrawal reflex were often lost before sheep were unconscious according to EEG recordings during non-stunned slaughter (Verhoeven et al., Reference Verhoeven, Gerritzen, Kluivers-Poodt, Hellebrekers and Kemp2015b). The objective of the current study was to assess absence and presence of the following indicators: threat-, withdrawal-, corneal- and eyelid reflex, and determine their relationship to (un)consciousness as identified by EEG recordings during stunned and non-stunned slaughter in veal calves.
Material and methods
Approval
This study was approved (DEC 2014045.b) by the Ethical Committee of the Animal Sciences Group of Wageningen UR, The Netherlands and in compliance with Dutch legislation.
Experimental set-up
On seven occasions, calves subjected to (non)stunned slaughter were observed at an abattoir in the Netherlands in the period from September to November 2014. Eighty-two cattle of mixed breeds (warm carcass weight 201±22 kg) were randomly selected from groups in lairage and held individually in a rotation box (Nawi, Borculo, the Netherlands) while equipped with non-invasive EEG electrodes. Calves were randomly assigned to one of the following four treatments: (1) Captive bolt stunning (Cash Magnum 9000 s) followed by neck cut within 30 s in an inverted position (180° rotation) (n=25); (2) Non-stunned slaughter in an upright position (n=7); (3) Non-stunned slaughter in an inverted position (180° rotation) (n=25); (4) Non-stunned slaughter in an upright position followed by captive bolt stunning 40 s after the neck cut (n=25). Treatment group 2 was limited to seven calves because of animal welfare concerns: that is delayed induction of unconsciousness due to insufficient bleeding. Calves were restrained with a head yoke and chin lift, but without a belly supporting plate. The neck cut was performed as a clean transverse incision of the neck, severing the skin, muscles, trachea, oesophagus, carotid arteries, jugular veins and major nerves. All stunning and slaughter procedures were performed by the same skilled halal slaughter man.
Electroencephalogram recordings
After being positioned in the restrainer, the head of the animal was shaved with an electrical trimmer (Aesculap favorita II GT104, Braun Suhl GmbH, Germany) to enable placement of EEG electrodes. Thereafter, an elastic halter was placed around the calves head. A cross-shaped piece of cotton was attached to the halter containing Velcro parts at each end of the cross. A ‘shooting-hole’ was cut in the middle of the cross, through which the captive bolt could be administered. Five rubber sensor carriers were placed around the ‘shooting-hole’ (Figure 1a and b). Five Ag/Cl pellet electrodes (Twente Medical Systems International (TMSi), Oldenzaal, The Netherlands) were placed in the rubber sensor carriers to make contact with the shaved skin of the calf. Each electrode was wrapped in a small sponge soaked in saline solution, which served as interface with the skin. One electrode was placed over the frontal bone, on the sagittal midline on a line extending between the base of both ears. Two electrodes were placed 2 cm left and right from the sagittal midline and 3 cm frontal to the first electrode, the other two electrodes were placed 2 cm left and right from the sagittal midline and 6 cm frontal to the first electrode. An elastic band was wrapped once around the calves’ head to secure the upper three electrodes, but leaving the ears and eyes free to move. All electrodes were connected via a 140 cm active shielded cable to the 32-channel Porti recording system (TMSi). Porti uses bipolar amplifier technology with high input impedance (>1 GΩ) that amplifies the potential difference between pairs of electrodes. The input amplifier is dimensioned as a multichannel instrumentation amplifier. Electrode impedance was <5 kΩ. The EEG was displayed with a band pass filter of 0.5 and 30 Hz, respectively, and raw, unfiltered, data were saved directly onto a computer. Sample rate was set at 0.5 kHz. Once the electrodes had been positioned correctly and a good live signal had been established, baseline EEG activity was recorded for at least 2 min. At the beginning of the slaughter procedure (T=0 s) calves were stunned (treatment 1) or neck cut in an upright position (treatment 2, 4) or inverted position (treatment 3). Calves of treatment 4 were captive bolt stunned 34±8 s after the neck cut. The EEG was recorded until a flat EEG (<10% baseline amplitude) was observed and all reflexes showed absence of response that was repeated sequentially three times. Hereafter, the equipment was carefully removed from the calf after which it was released from the restrainer to allow further processing of the carcass.
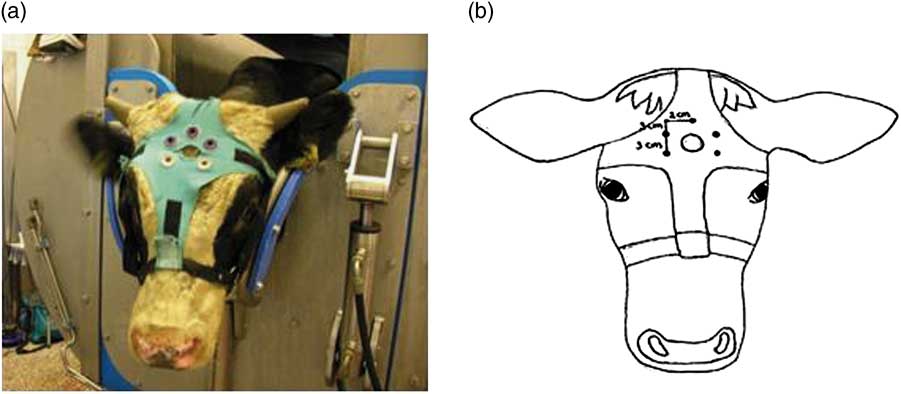
Figure 1 (a, b) Electroencephalogram (EEG) electrode placement on a veal calf.
Measurements around stunning and slaughter
The threat-, withdrawal-, corneal- and eyelid reflex were verified once before the slaughter treatment started. The threat reflex was assessed by checking the presence of a blinking reaction to an abrupt movement of the index finger towards the eye ball without touching the eye. The withdrawal reflex was studied by pinching the calves nose between two fingertips and determining whether or not it responded with a withdrawal of the nose or head. In case of non-stunned slaughter only withdrawal of the nose could be observed after the neck cut. The corneal reflex was assessed by a gentle touch of the cornea with the index finger, and the presence/absence of a blinking reaction was recorded. The eyelid reflex was assessed by a gentle touch of the eyelid, and the presence/absence of a blinking reaction was recorded. Reflexes were assessed every 20 s from T=15 s onwards for all treatments until all reflex tests showed a negative response that was repeated sequentially three times and a flat EEG was recorded. In addition, reflexes were assessed 5 s after captive bolt stunning in calves of treatment 1 and 4. Reflex tests were performed in a random order per calf.
Data analyses
EEG data were displayed, stored and analysed using PolyBench software (TMSI). EEG activity (amplitude and frequency) of each calf was visually assessed to determine the start of the following stages: baseline, transitional, unconscious and minimal brain activity. Figure 2 shows an example of a representative series of 5 s of EEG registration during these different stages. The baseline stage consisted of a low amplitude, high frequency signal, indicating alert calves (Figure 2a). The transitional stage is characterized by low frequency, high amplitude (firing of neurons in a synchronized fashion) becoming more apparent compared with baseline, associated with reduced consciousness (Figure 2b, McKeegan et al., Reference McKeegan, Reimert, Hindle, Boulcott, Sparrey, Wathes, Demmers and Gerritzen2013). When low frequency, high amplitude dominated the EEG trace this was interpreted to indicate unconsciousness (Figure 2c, Baars et al., Reference Baars, Ramsøy and Laureys2003). Minimal brain activity was reflected by a flat signal (<10% of baseline amplitude, Figure 2d). Time to loss of a reflex was defined as the first time at which a reflex showed a negative response that was repeated sequentially three times, since loss and return of a reflex was observed in some calves.
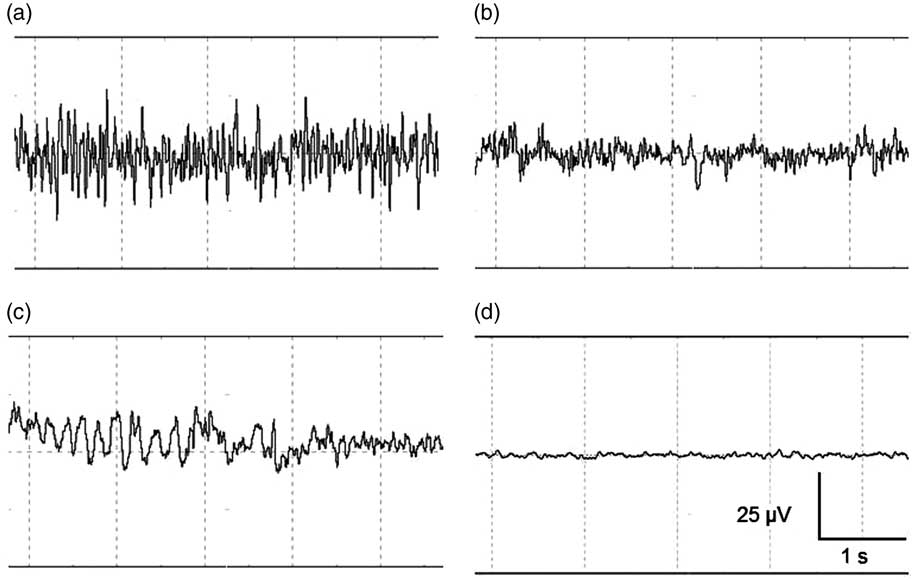
Figure 2 (a–d) Representative examples of the different stages identified with visual assessment of electroencephalogram (EEG) activity before and after stunned and non-stunned slaughter in calves. The four stages from left to right and top to bottom: baseline (a), transitional (b), unconscious (c), and minimal brain activity (d). Total x-axis represents 5 s, y-axis represents amplitude of the EEG-trace (µV).
Statistical analyses
To calculate sample size, a power analysis was performed using the Power Calculation version 2.03 programme, designed by van Wilgenburg and van Schaick Zillesen, 2003 version. Results indicated that a sample of at least 10 calves per treatment would be required to detect a difference of 20 s in loss of consciousness with 90% power using a t-test between means with α at 0.05. The sample size was increased to 25 calves per treatment to allow for possible loss of EEG recordings.
Statistical analyses were performed using SAS (version 9.3; SAS Institute, 2004). Normality of variance was checked (PROC UNIVARIATE) for all the variables examined. Before the analyses, the variables ‘time to lost eyelid reflex’ and ‘ time to lost corneal reflex’ were log transformed to normalize the variance within these variables. In order to determine whether or not non-stunned slaughter in an upright or inverted position had a significant effect on time to onset of different EEG stages and time to loss of reflexes, data were analysed using a GLM procedure according to the model:

where T i is the treatment (2 and 3), and ε ij the residual error term.
Stunned slaughter (treatments 1 and 4) was not taken into account in this procedure, since unconsciousness was immediately induced after stunning in these animals.
Differences were considered to be significant at the 5% probability level.
Results
Animals
The total number of calves used in this study was 82. In one calf from treatment 4, the EEG signal was lost after stunning and consequently assessment of loss of consciousness was impossible. Data from this calf was excluded from further analyses.
Electroencephalogram activity
A clear EEG signal was obtained from all calves during baseline recordings. Based on EEG recordings, calves stunned with a captive bolt (treatment 1) were unconscious 1±0 s after stunning. No transitional stage was observed in these calves. One calf, however, lost consciousness 11 s after stunning based on EEG recordings. Minimal brain activity was observed in all calves from 22±19 s after stunning. Calves of treatments 2 and 3 developed a transitional EEG based on EEG recordings 54±13 and 27±15 s, respectively, after the neck cut. Calves of treatments 2 and 3 were considered unconscious based on EEG recordings 109±32 and 49±25 s, respectively, after the neck cut. Calves of treatments 2 and 3 had minimal brain activity based on EEG recordings 157±50 and 86±34 s, respectively, after the neck cut.
Figure 3 represents the percentage of calves displaying different EEG stages over time subjected to non-stunned slaughter in an upright position (treatment 2) or an inverted position (treatment 3) at T=0 s. The time span for 80% or 100% of the calves to reach a transitional EEG, unconsciousness or minimal brain activity is displayed in Table 1. Eighty per cent of the calves slaughtered in an upright position (treatment 2) lost consciousness on average 61 s later than calves slaughtered in an inverted position (treatment 3). All the calves slaughtered in an upright position (treatment 2) lost consciousness on average 31 s later than calves slaughtered in an inverted position (treatment 3).
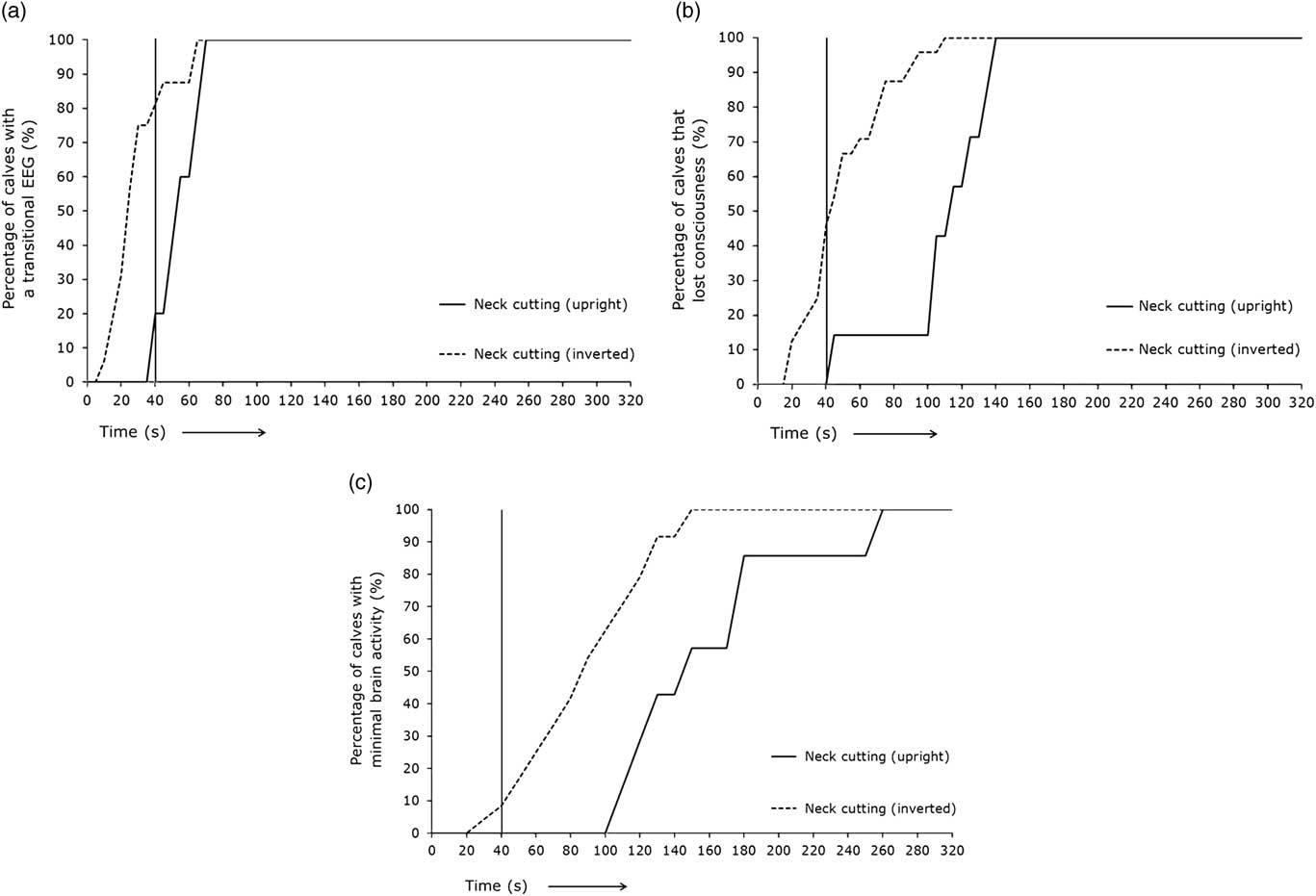
Figure 3 (a–c) Percentage of calves with different electroencephalogram (EEG) stages through time after non-stunned slaughter in an upright position (red lines, n=7) or inverted position (blue lines, n=25) at T=0 s. Vertical black line represents the percentage of calves with a specific EEG stage at 40 s.
Table 1 Time points at which 80% or 100% of the calves subjected to non-stunned slaughter (T=0 s) in an upright (n=7) or inverted position (n=25) had a specific electroencephalogram (EEG) stage

Of the treatment 2 calves, 20% had a transitional EEG, none were considered unconscious and none had minimal brain activity based on EEG recordings 40 s after the neck cut. Of the treatment 3 calves, 81% of the calves had a transitional EEG, 46% were considered unconscious and 8% had minimal brain activity based on EEG recordings 40 s after the neck cut.
Treatment 4 calves (non-stunned slaughter in an upright position followed by captive bolt stunning) were stunned on average 34±8 s after the neck cut. At stunning, 67% of the calves were conscious, 8% of the calves had a transitional EEG, 21% of the calves were unconscious and 4% of the calves displayed minimal brain activity. All calves that were conscious or had a transitional EEG before stunning were unconscious 1±0 s after stunning based on EEG recordings.
Loss of reflexes for unconsciousness
Reflexes were tested and present in all calves during baseline recordings. In calves of treatment 1, threat-, withdrawal-, corneal- and eyelid reflex were all permanently lost at the first testing moment (T=5 s) after captive bolt stunning. Figure 4a presents the range of individual time points at which the different reflexes were lost in relation to onset of the different EEG stages (T=0 min), based on visual assessment of EEG recordings during non-stunned slaughter of calves in an upright position (treatment 2). In all treatment 2 calves, the threat-, and withdrawal reflex were both lost before EEG-based loss of consciousness had been observed. The corneal-, and eyelid reflex were both lost in all calves of treatment 2 after EEG-based loss of consciousness was observed. Corneal- and eyelid reflex were lost in 71% and 86%, respectively, of calves of treatment 2 after onset of minimal brain activity.
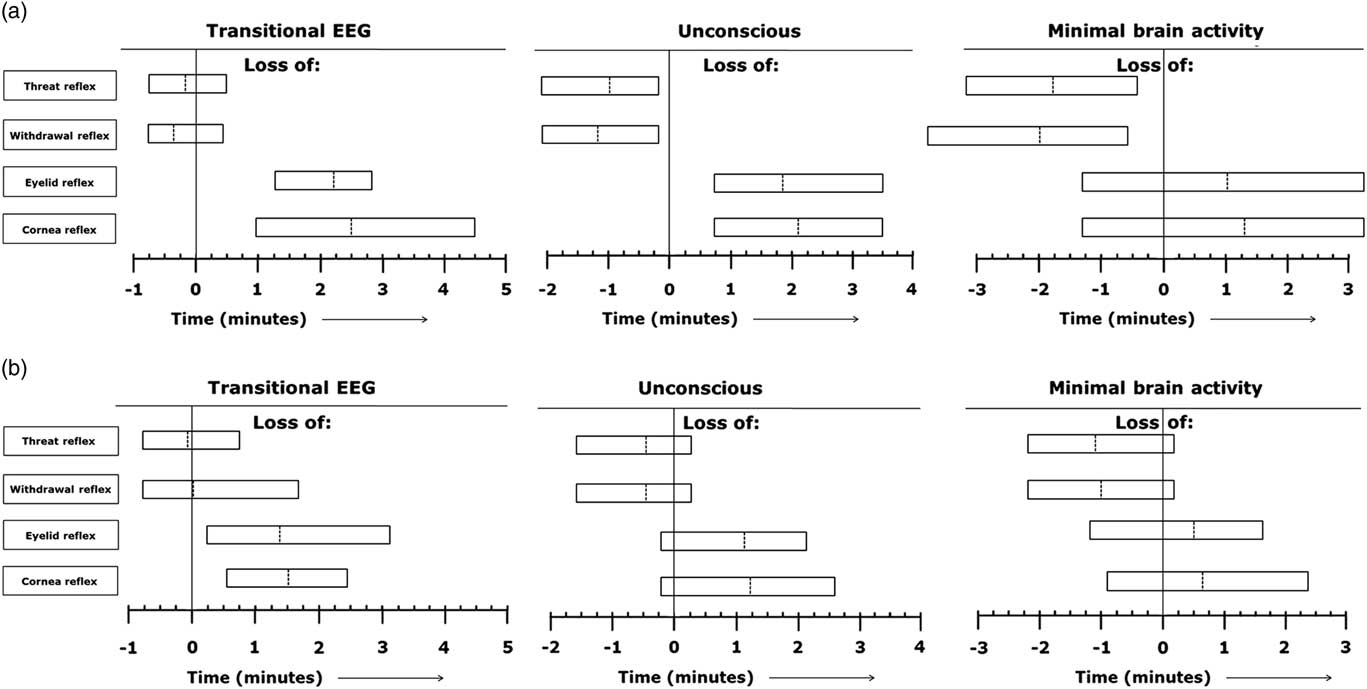
Figure 4 (a, b) Range of individual time points at which the different reflexes are lost in relation to start of the different electroencephalogram (EEG) stages (T=0 min) based on visual assessment of EEG recordings during non-stunned slaughter of calves in an upright position (n=7) (Figure 4a) and an inverted position (n=25) (Figure 4b). Dotted lines indicate average values of all observations and left and right vertical lines of each block indicate minimum and maximum values for all observations.
Figure 4b represents the range of individual time points at which the different reflexes were lost in relation to onset of the different EEG stages (T=0 min), based on visual assessment of EEG recordings during non-stunned slaughter of calves in an inverted position (treatment 3). In calves of treatment 3, the threat- and withdrawal reflex were lost in 88% and 92% respectively, of the animals before EEG-based loss of consciousness was observed. Both corneal- and eyelid reflex were lost in 92% of calves of treatment 3 after EEG-based loss of consciousness had been observed. The threat- and withdrawal reflex were lost in 92% and 96%, respectively, of calves of treatment 3 before minimal brain activity was observed. The corneal- and eyelid reflex were lost in 88% and 67%, respectively, of calves of treatment 3 after minimal brain activity had been observed. Calves of treatments 2 and 3 lost the corneal reflex 235±86 and 123±34 s, respectively, after the neck cut. Calves of treatments 2 and 3 lost the eyelid reflex 218±65 and 115±36 s, respectively, after the neck cut.
All calves of treatment 4, lost both the threat- or withdrawal reflex before EEG-based loss of consciousness. The corneal- or eyelid reflex were lost in 89% and 87%, respectively, of the calves after EEG-based loss of consciousness. The threat-, withdrawal-, corneal- and eyelid reflex were all permanently lost 5 s after the captive bolt stunning.
Discussion
The main aim of this study was to provide insight into the validity of certain reflexes that are used to assess unconsciousness at slaughter in veal calves, that is the threat-, withdrawal-, corneal- and eyelid reflex. Brain activity, as presented in an electroencephalogram or EEG, served as a ‘gold standard’ for our assessment of unconsciousness.
Consciousness involves the cerebral cortex and thalamus, together forming the thalamocortical complex, which is regulated by the brainstem. The central core of the brainstem is formed by the reticular formation, a large network of neural tissue located in the central region of the brain stem. Neuronal projections from the reticular formation to the cortex are called the ascending reticular activating system (ARAS). A good-functioning reticular formation and ARAS are essential for the maintenance of consciousness and damage to (one of) these regions causes rapid loss of consciousness. Unconsciousness induced by penetrating captive bolt stunning is caused by a combination of the concussive force generated by impact of the bolt on the cranium and irreversible physical damage produced by passage of the bolt through the brain. Generally, the pistol is placed on the forehead after which the bolt penetrates the skull to target the brain stem. Immediate loss of consciousness and reflexes should occur from the resulting trauma to the cerebral hemisphere and brain stem (Terlouw et al., accepted a). Depending on the method of stunning, the EEG shows a characteristic pattern of change when animals lose consciousness. Generally, an increase in low frequency activity is accompanied by an increase in amplitude. When neurons depolarize at the same time or frequency, they fire in a synchronized fashion creating slow high amplitude waves as seen in unconscious states suggesting a depression of the reticular formation (Lopes da Silva, Reference Lopes da Silva1983). EEG recordings in captive bolt stunned animals displayed high-amplitude, slow-frequency waves after stunning, indicating immediate unconsciousness, similar to findings of Lambooy and Spanjaard (Reference Lambooy and Spanjaard1981) and Zulkifli et al. (Reference Zulkifli, Goh, Norbaiyah, Sazili, Lotfi, Soleimani and Small2014). One calf in the current study displayed a period of baseline-like EEG activity, lasting for 11 s after captive-bolt stunning. This calf displayed a permanent loss of all reflexes from 5 s after stunning. Reflexes in the conscious calf before the beginning of the slaughter procedure were always present and it is therefore unlikely that these reflexes were lost, though the calf was conscious. The prolonged period of baseline-like EEG activity may have been caused by incorrect positioning of the stunner, a deflected shot, incorrect functioning of the stunning device itself or an ineffective EEG recording.
Effectively shot cattle should collapse immediately due to damage to the reticular formation that also plays a role in maintaining posture (Laureys and Tononi, Reference Laureys and Tononi2009). In our study, an immediate collapse was observed in some of the calves, but tight restraining of the calf often prevented collapse and made observation of this criterion difficult. Five seconds after captive bolt stunning all reflexes were permanently lost in all calves. Rhythmic breathing and the righting reflex are considered indicative of a (partial) return of consciousness and thus that the stunning has not been irreversible (Grandin, Reference Grandin2002). Both rhythmic breathing and the righting reflex could not be assessed because the animals were fully restrained in a rotatory box.
Non-stunned animals that are subjected to neck cutting lose consciousness gradually as a consequence of widespread anoxia in the brain that results in progressive dysfunction of cortical and subcortical structures (Terlouw et al., accepted b). During our experiment, calves in an inverted or upright position lost consciousness, based on EEG recordings, 49±25 and 109±32 s, respectively (mean±SD), after the neck cut. Previous studies in cattle indicated loss of consciousness ranging from 10 s up to over 3 min after the neck cut (Daly et al., Reference Daly, Kallweit and Ellendorf1988; Gregory et al., Reference Gregory, Wenzlawowicz and Von Holleben2010; Bourguet et al. Reference Bourguet, Deiss, Tannugi and Terlouw2011). Newhook and Blackmore (Reference Newhook and Blackmore1982) suggested the possibility of an intermittent return to consciousness for up to 123 to 323 s after non-stunned slaughter in cattle. Detailed study of EEG recordings did not indicate signs of resurgence of consciousness in any of the calves in our study. The corneal- and eyelid reflex, however, were lost and regained in 9% and 16%, respectively, of the non-stunned calves (n=32) during bleeding. Loss and return of the corneal reflex has been observed by Hoffman in an unspecified number of cattle (Hoffman, 1900 cited by Gregory et al. Reference Gregory, Wenzlawowicz and Von Holleben2010). Bourguet et al. (Reference Bourguet, Deiss, Tannugi and Terlouw2011) also observed a temporary return of the palpebral and corneal reflex in some of the non-stunned slaughtered cattle. A temporary return of blood pressure would be necessary for these reflexes to return and may possibly be explained by physiological changes, such as an increased heart rate, to alleviate the drop in blood pressure (Newhook & Blackmore, Reference Newhook and Blackmore1982; Vimini et al., Reference Vimini, Field, Riley and Varnell1983). There is no clear indication why reflexes were regained and lost again in some of the calves in the current experiment.
Because this experiment took place at a commercial slaughter plant, the slaughter man was empowered to decide when an additional cut was necessary. No clear criteria were defined for this. In total 15 of 82 (18%) calves in our study were cut a second time and one of those 15 calves was cut a third time. The arteries of an animal in which poor bleeding is observed, are in practice often re-cut to allow adequate bleeding. Poor bleeding may be caused by occlusion, which is characterized by retraction and contraction of the elastic portion of the arterial wall and thrombus formation around the severed end of the vessel (Gregory et al., Reference Gregory, Shaw, Whitford and Patterson-Kane2006). The prevalence of carotid arterial occlusion in 576 cattle slaughtered at abattoirs in the United Kingdom was found to be 16% and 25%, respectively, for adult cattle and bobby calves (Gregory et al., Reference Gregory, Shaw, Whitford and Patterson-Kane2006). A study by Gregory et al. (Reference Gregory, Wenzlawowicz and Von Holleben2010) showed that 71% of cattle taking longer than 75 s to collapse, had a false aneurysm in the cardiac end of the carotid that was at least 3 cm in diameter. There was no further investigation into the background of this obstruction, but it may provide an explanation for the large variation in time to loss of consciousness between calves.
Duration to loss of consciousness was 50 s longer in non-stunned veal calves restrained upright than by those restrained in an inverted position. During this experiment, all calves were slaughtered in the same rotation box which normally is used for inverted slaughter. Hence, slaughter using a box designed for upright restraint may provide different results. Blood flow in calves slaughtered upright was often obstructed when the head fell limp against the head restrainer. Based on this observation and in consideration of the potential risk to animal welfare, the number of veal calves slaughtered non-stunned in an upright position was limited to seven calves. Inversion of cattle for slaughter in itself is widely debated and thought to cause distress because of a longer delay between entering the restrainer and full restraint. In addition there is discomfort from the inverted position resulting in hypoxemia and rumen pressure on the diaphragm (Dunn, Reference Dunn1990; Petty et al., Reference Petty, Hattingh, Ganhao and Bezuidenhout1994; Tagawa et al., Reference Tagawa, Okano, Sako, Orima and Steffey1994). Another potential welfare problem is aspiration of blood and refluxing gut content after neck cutting in non-stunned animals (EFSA, 2004). Though the latter problem is mainly associated with the inverted position, it has also been observed in non-stunned slaughtered calves in the upright position (Grandin and Regenstein, Reference Grandin and Regenstein1994; Gregory et al., 2009). A report by the Farm Animal Welfare Committee (2012) stated that there is little evidence of any welfare advantages of inversion in terms of speed and efficiency of the cut. Visits to 18 European cattle slaughter plants showed that the mean number of cuts was higher for cattle restrained in an upright position than those in other restraining systems (Dialrel, 2010). Although others claim, that the angle of the head in inverted cattle allows for a more effective downward cut compared to the upward cut in animals in an upright position (Slaughter of Animals (prevention of cruelty) Regulations 1958 – quoted by Dunn, Reference Dunn1990). The present study is, to our knowledge, the first to suggest that the neck cut may be more effective in terms of duration to loss of consciousness in non-stunned slaughtered animals in an inverted position.
Reflexes in non-stunned slaughtered animals were not lost immediately, as in captive bolt stunned animals, but disappeared gradually. Generally, the threat reflex and withdrawal reflex were lost first, followed by the corneal- and eyelid reflex. Loss of reflexes in this order may be dependent on the difference in resistance to anoxia by different parts of the brain. Functions that rely on integration of cortical processes are more susceptible to anoxia than those originating from lower brain structures, such as the brain stem (Hansen, Reference Hansen1985). On average, the threat reflex was lost in non-stunned calves before EEG-based unconsciousness. The rapid loss of the threat reflex can possibly be explained by the sudden drop in blood flow after neck cutting causing hypoxia of the brain cells involved (Bourguet et al., Reference Bourguet, Deiss, Tannugi and Terlouw2011). A similar phenomenon was also observed for the withdrawal reflex that was lost on average before EEG-based loss of consciousness. When the neck is cut, the knife transects veins, arteries and other blood vessels, skin, muscle, trachea, oesophagus, sensory- and motor nerves, and connective tissue. Transecting these soft tissues will send a considerable amount of neural impulses to the brain (Gregory, Reference Gregory2004). The massive stimulation of all sensory nerves after the neck cut may overrule the neural input from other, milder sources of pain, such as pinching the nose. Opinions on pain perception during neck cutting are divided. Some (e.g. Grandin, Reference Grandin1994; Rosen, Reference Rosen2004) state that use of an exquisitely sharp knife, will prevent pain after the neck cut. Others (e.g. Gibson et al., Reference Gibson, Johnson, Murrell, Hulls, Mitchinson, Stafford, Johnstone and Mellor2007 and Reference Gibson, Johnson, Stafford, Mitchinson and Mellor2009; Mellor et al., Reference Mellor, Gibson and Johnson2009) state that the ventral neck cut is a noxious stimulus and that there is strong evidence that this cut would be perceived as painful from the time of the neck cut until loss of consciousness. Until now, neurophysiological methodology has not provided a final and definitive answer to this issue.
Both the corneal- and eyelid reflex require a functional afferent cranial nerve V (trigeminal) and efferent cranial nerve VII (facial) and the relevant eye muscles to function adequately (Adams and Sheridan, Reference Adams and Sheridan2008). The connections between the spinal trigeminal- and facial nuclei pass through the reticular formation and failure of these reflexes often indicates a wider dysfunction of the brain, comprising part of the reticular formation, and thus a state of unconsciousness (Terlouw et al., accepted b). In the present study, the corneal reflex in non-stunned slaughtered calves was lost on average 85±45 s after EEG-based loss of consciousness and on average 47±63 s after minimal brain activity was observed. Lambooij et al. (Reference Lambooij, van der Werf, Reimert and Hindle2012) and Newhook and Blackmore (Reference Newhook and Blackmore1982) observed that non-stunned slaughtered calves lost the corneal reflex up to 1 min after calves were unconscious (EEG-based) and this reflex was even observed in some calves after all EEG activity had been lost. Verhoeven et al. (Reference Verhoeven, Gerritzen, Kluivers-Poodt, Hellebrekers and Kemp2015b) observed that non-stunned slaughtered sheep lost the corneal reflex on average 00:59±00:17 min (mean±SD) after sheep were considered to be unconscious based on EEG recordings. The eyelid reflex in non-stunned slaughtered calves was lost on average 76±50 s after EEG-based loss of consciousness and 37±59 s after minimal brain activity was observed. Other studies report the presence of an eyelid reflex in unconscious sheep (Verhoeven et al., Reference Verhoeven, Gerritzen, Kluivers-Poodt, Hellebrekers and Kemp2015b) and cattle (Newhook and Blackmore, Reference Newhook and Blackmore1982) and also during anaesthesia. The eyelid reflex is known to be present after an animal is considered unconscious (Dugdale, Reference Dugdale2010). The order in which the eyelid- and corneal reflex cease may differ, providing uncertainty which reflex is more resistant to the effects of anoxia (Bourguet et al., Reference Bourguet, Deiss, Tannugi and Terlouw2011; Terlouw et al., accepted b).
The results from this study support the hypothesis that a positive corneal- or eyelid reflex alone does not necessarily indicate consciousness, since positive brain stem reflexes might occur on the basis of residual brain stem activity and do not distinguish clearly between consciousness and unconsciousness (Anil, Reference Anil1991). Consequently, a negative corneal- or eyelid reflex is considered a general indication of unconsciousness (Laureys, Reference Laureys2005; Terlouw et al., accepted b).
Conclusions
In both stunned and non-stunned calves, unconsciousness was induced and absence and presence of reflexes was successfully linked to EEG activity. The use of reflexes to assess unconsciousness can more easily be applied in daily practice, and EEG recordings can be used to validate the interpretation of such reflexes. After captive bolt stunning, absence of threat-, withdrawal-, corneal- and eyelid reflex indicated unconsciousness as determined by EEG recordings. On average, after non-stunned slaughter, the threat- and withdrawal reflex were lost before calves were considered unconscious based on EEG recordings. The eyelid- and corneal reflex were on average lost after calves were considered unconscious based on EEG recordings and appeared to be distinctly conservative indicators of unconsciousness in non-stunned slaughtered calves since they were observed up to 76±50 and 85±45 s (mean±SD) respectively after EEG-based onset of unconsciousness.
Acknowledgements
The authors would like to thank Vincent Hindle and Samantha Surquin for their support while collecting the data. Jan Peuscher is acknowledged for his technical assistance while collecting the data and his help during data analyses. The authors also acknowledge the owner and employees of the slaughter plant for their assistance and hospitality during the study. Funding for this project was provided by the Dutch Ministry of Economic Affairs (KB-12-006.01-002).