Implications
Feeding strategies designed to mitigate enteric CH4 emissions in cattle have been tested by incorporating a range of tropical plants (foliages, pods and seeds), nitrates and vegetable oils to rations based on low-quality tropical grasses. These strategies have proved to be effective in decreasing enteric methane emissions by cattle. This could lead to an improvement in profitability of production by reducing the incorporation of expensive feedstuffs in rations and thus to sustainable ruminant production systems for smallholders in the tropical regions of the world.
Introduction
Animal agriculture, in developing countries, will face tremendous challenges with respect to mitigation and adaptation to climate change in the following years as an increasing demand for meat and milk by the burgeoning population is expected. This increase in demand is projected to result in an increase in livestock numbers in the near future. Considerable research efforts have been invested in accurately predicting enteric methane emissions from cattle (Escobar-Bahamondes et al., Reference Escobar-Bahamondes, Oba and Beauchemin2016; Eugène et al., Reference Eugène, Sauvant, Nozière, Viallard, Oueslati, Lherm, Mathias and Doreau2019; van Lingen et al., Reference van Lingen, Niu, Kebreab, Valadares Filho, Rooke, Duthie, Schwarm, Kreuzer, Hynd, Caetano, Eugène, Martin, McGee, O’Kiely, Hünerberg, McAllister, Berchielli, Messana, Peiren, Chaves, Charmley, Cole, Hales, Lee, Berndt, Reynolds, Crompton, Bayat, Yáñez-Ruiz, Yu, Bannink, Dijkstra, Casper and Hristov2019) as well as in implementing appropriate enteric CH4 mitigation strategies (Beauchemin et al., Reference Beauchemin, Ungerfeld, Eckard and Wang2020) in ruminant production systems.
During the dry season, most tropical grasses have a high NDF and low CP contents, resulting in limited fermentation of DM, long retention time of digesta and low absorption of volatile fatty acids (VFA) from the rumen, leading to modest daily weight gains in growing cattle and considerable emissions of enteric methane. More than 200 000 plant secondary compounds such as condensed tannins, saponins, flavonoids, organosulfur compounds and essential oils (EOs) have been identified as having potential to mitigate CH4 emissions in grazing ruminants (Jafari et al., Reference Jafari, Ebrahimi, Goh, Rajion, Jahromi and Al-Jumaili2019; Molina-Botero et al., Reference Molina-Botero, Arroyave-Jaramillo, Valencia-Salazar, Barahona-Rosales, Aguilar-Pérez, Ayala Burgos, Arango and Ku-Vera2019a). Secondary compounds in plants should induce a reduction in CH4 production (Figure 1) without negatively affecting rumen fermentation or being toxic to the animal, and should ideally improve productive performance, increase economic gains, be easy to handle and be of low cost to farmers. The effectiveness of these plant secondary compounds depends on their type, concentration and ingested amount. In the medium term, it is important to identify cost-effective secondary compounds which could improve rumen fermentation, VFA production and mitigate CH4 emissions with a lasting effect. One effective methane mitigation strategy is the inclusion of low levels of oils in rations which induce biohydrogenation of unsaturated fatty acids, increase energy density and inhibit protozoa (Knapp et al., Reference Knapp, Laur, Vadas, Weiss and Tricarico2014). A number of comprehensive reviews on methane mitigation in ruminants have been published recently (Berndt and Tomkins, Reference Berndt and Tomkins2013; Knapp et al., Reference Knapp, Laur, Vadas, Weiss and Tricarico2014; Beauchemin et al., Reference Beauchemin, Ungerfeld, Eckard and Wang2020), but relatively few have emphasized the current situation in tropical cattle production systems in developing countries.

Figure 1 Metabolic and digestive effects of condensed tannins, essential oils and saponins on methane synthesis and animal production. (−): decrease; (+): increase. Modified from Valencia Salazar (Reference Valencia Salazar2017).
Ruminant production in developing countries (mostly in the tropics) faces enormous challenges to both increase productivity and decrease greenhouse gas (GHG) emissions (Ayantunde et al., Reference Ayantunde, Fernández-Rivera and McCrabb2005). The achievement of CH4 mitigation at the farm level can bring environmental benefits and increase profits for small farmers in tropical regions. Small-scale cattle farmers in the tropical regions of Latin America and the Caribbean, Asia and Africa confront formidable obstacles in achieving sustainable intensification of animal production (Ndung’u et al., Reference Ndung’u, Bebe, Ondiek, Butterbach-Bahl, Merbold and Goopy2019). Poverty, poor literacy, scarcity of financial resources (credits), limited access to markets, lack of technical support and paradoxically, the ominous impacts of climate change (droughts, floods and fires) are just but a few of the present constraints.
The rationale behind this review is to describe and critically discuss the advantages of one of the simplest and most direct options for enteric CH4 mitigation available to small-scale cattle farmers in the tropics, namely the incorporation of foliages, seeds and pods of widely distributed tropical plants, by-products and additives in practical rations.
Enteric methane production by cattle fed tropical grasses
There are many factors influencing CH4 yield such as type of grass (C3 v. C4), chemical composition, forage : concentrate ratio, rate of passage of digesta through the rumen, physiological stage, breed, sex and it is assumed that all those are integrated within the single expression of DM intake (Archimède et al., Reference Archimède, Eugène, Marie Magdeleine, Boval, Martin, Morgavi, Lecomte and Doreau2011 and Reference Archimède, Rira, Eugène, Fleury, Lastel, Periacarpin, Silou-Etienne, Morgavi and Doreau2018). Dry matter intake is the result of both grass and cattle interactions which affect rumen fermentation and, as such, represents a convenient basis for estimating enteric methane production. More work is required by research groups in tropical regions on the establishment of robust data sets from which accurate CH4 yields could be derived for methane inventory purposes (Patra, Reference Patra2017; Castelán Ortega et al., Reference Castelán Ortega, Ku Vera, Ángeles-Hernández, Hernández-Pineda, Benaouda, Molina, Ramírez-Cancino, Castelán-Jaime, Praga-Ayala, Lazos-Balbuena, Montelongo-Pérez, González-Ronquillo, Vázquez-Carrillo, Cardoso-Gutiérrez, Aranda-Aguirre, Villegas-Estrada, Guadarrama-López and Apodaca-Martínez2019; Goopy et al., Reference Goopy, Korir, Pelster, Ali, Wassie, Schlecht, Dickhoefer, Merbold and Butterbach-Bahl2020) and for evaluation of effective enteric CH4 mitigation strategies.
A series of experiments involving methane emissions were carried out in Mexico with cattle housed in open-circuit respiration chambers and fed basal rations of low-quality tropical grass (>70% of ration DM) and a supplement (concentrate). Preliminary results showed a methane yield of 17.4 g CH4/kg DMI in Nellore (Bos indicus) bulls that were fed chopped Pennisetum purpureum grass (Canul-Solís et al., Reference Canul-Solís, Piñeiro-Vázquez, Arceo-Castillo, Alayón-Gamboa, Ayala-Burgos, Aguilar-Pérez, Solorio-Sánchez, Castelán-Ortega, Lachica-López, Quintana-Owen and Ku-Vera2017), a value that compares reasonably well with the predicted methane yield of 18.0 g CH4/kg DM intake (n = 66; R 2 = 0.73) in cattle fed low-quality tropical grass (~70% ration DM) reported by Ku-Vera et al. (Reference Ku-Vera, Valencia-Salazar, Piñeiro-Vázquez, Molina-Botero, Arroyave-Jaramillo, Montoya-Flores, Lazos-Balbuena, Canul-Solís, Arceo-Castillo, Ramírez-Cancino, Escobar-Restrepo, Alayón-Gamboa, Jiménez-Ferrer, Zavala-Escalante, Castelán-Ortega, Quintana-Owen, Ayala-Burgos, Aguilar-Pérez and Solorio-Sánchez2018) and with the methane yield reported by Noguera and Posada-Ochoa (Reference Noguera and Posada-Ochoa2017) who reported 16.1 g CH4/kg DMI in lactating cows fed Pennisetum clandestinum in Colombia. The values reported by the cited studies (Noguera and Posada-Ochoa, Reference Noguera and Posada-Ochoa2017; Canul-Solís et al., Reference Canul-Solís, Piñeiro-Vázquez, Arceo-Castillo, Alayón-Gamboa, Ayala-Burgos, Aguilar-Pérez, Solorio-Sánchez, Castelán-Ortega, Lachica-López, Quintana-Owen and Ku-Vera2017; Ku-Vera et al., Reference Ku-Vera, Valencia-Salazar, Piñeiro-Vázquez, Molina-Botero, Arroyave-Jaramillo, Montoya-Flores, Lazos-Balbuena, Canul-Solís, Arceo-Castillo, Ramírez-Cancino, Escobar-Restrepo, Alayón-Gamboa, Jiménez-Ferrer, Zavala-Escalante, Castelán-Ortega, Quintana-Owen, Ayala-Burgos, Aguilar-Pérez and Solorio-Sánchez2018) represent an approximation for cattle fed tropical grasses, and they are comparable to some extent to the data from Charmley et al. (Reference Charmley, Williams, Moate, Hegarty, Herd, Oddy, Reyenga, Staunton, Anderson and Hannah2016) who reported a methane yield of 19.3 g CH4/kg DMI (n = 133) for Zebu (Brahman) cattle fed tropical grasses (>70% of DM) as a basal ration in Australia. Although it has been claimed that tropical (C4) grasses contribute to enteric methane emissions to a greater extent than temperate (C3) grasses (Archimède et al., Reference Archimède, Rira, Eugène, Fleury, Lastel, Periacarpin, Silou-Etienne, Morgavi and Doreau2018), the evidence hereby presented does not support that contention (Benaouda, Reference Benaouda2018; Goopy et al., Reference Goopy, Onyango, Dickhoefer and Butterbach-Bahl2018; Table 1). The overall enteric methane yield from experiments carried out in Mexico in respiration chambers is 17.0 g CH4/kg DMI (n = 125) for cattle consuming low-quality tropical grasses. This relatively low yield could be partially explained by the low rate and extent of fermentation of DM (~40%) in the rumen of cattle fed tropical grass during the dry season. Strategic grazing management (with a canopy with light interception of 95%) results in reduction in CH4 emission intensity and greater milk yield in grazing cows, as well as 18% less CH4/kg DM intake (Congio et al., Reference Congio, Batalha, Chiavegato, Berndt, Oliveira, Frighetto, Maxwell, Gregorini and Da Silva2018). The so-called rotatinuous grazing management (Savian et al., Reference Savian, Schons, Marchi, de Freitas, da Silva Neto, Mezzalira, Berndt, Bayer and Carvalho2018) also results in a decrease in CH4 emission intensity by carefully controlling grazing behavior of ruminants. Both approaches support the contention that it is through grazing management that CH4 emission intensity in ruminants can be decreased without any other further intervention. Nonetheless, Berça et al. (Reference Berça, Cardoso, Longhini, Tedeschi, Boddey, Berndt, Reis and Ruggieri2019) found no reductions in enteric methane emissions of crossbred dairy heifers grazing Urochloa brizantha cv. Marandu either fertilized or with the inclusion of the legume Arachis pintoi in Brazil. There are several possible strategies to mitigate methane emissions in ruminants that are fed low-quality tropical grasses. The selection criteria for a particular strategy must be based on sound economic grounds, as smallholders are limited economically in their capacity to buy expensive methane mitigating additives (e.g. monensin, 3-nitrooxypropanol). Leucaena leucocephala, a native legume shrub from Mexico and Central America, ranks first among the options available to develop sustainable beef cattle farming systems in tropical regions (Shelton and Dalzell, Reference Shelton and Dalzell2007; Harrison et al., Reference Harrison, McSweeney, Tomkins and Eckard2015; Ramírez-Avilés et al., Reference Ramírez-Avilés, Solorio-Sánchez, Aguilar-Pérez, Ayala-Burgos and Ku-Vera2019). In Colombia, Molina et al. (Reference Molina, Angarita, Mayorga, Chará and Barahona-Rosales2016) fed Lucerna heifers with a Cynodon plectostachyus (74% DM) and Leucaena leucocephala (26% DM) ration while cattle were housed in polytunnels for methane measurements and recorded a lower intensity of methane emissions compared to heifers fed only the tropical grass. Also in Colombia, Sierra-Montoya et al. (Reference Sierra-Montoya, Chará and Barahona-Rosales2017) found a reasonably good milk yield (~10 to 12 l cow/day) in recently calved or early lactating Gyr × Holstein cows grazing a silvopastoral system consisting of Cynodon plectostachyus and Leucaena leucocephala, obtaining a good balance of metabolizable protein to metabolizable energy.
Table 1 Enteric methane emissions of cattle in the tropics of Asia, Africa, Oceania and Latin America as measured in respiration chambers

LW = live weight; DMI = DM intake; CH4 = methane; TMR = total mixed ration; GEI = gross energy intake; Y m = CH4 loss expressed as percentage of GEI.
Enteric methane mitigation strategies in cattle under small-scale farming conditions
Before adoption or implementation at farm level, an enteric methane mitigation strategy must induce a significant extent of mitigation as demonstrated in various experiments, published equations applicable to various types of ruminants, feasibility of adoption by farmers, cost-effectiveness analysis and repeatability (Eugène et al., Reference Eugène, Sauvant, Nozière, Viallard, Oueslati, Lherm, Mathias and Doreau2019), as well as persistency. This review addresses three different options for mitigation: secondary compounds contained in plant species available in tropical regions that can be used in silvopastoral systems, high-energy compounds such as oils and finally a chemical compound exemplified by the feeding of additives such as nitrates. Nitrates require attention, particularly under the conditions of tropical ruminant production, since they supply N, an element which is usually deficient in C4 grasses during the long dry season.
Foliage and pods of tropical plants as a methane mitigation strategy
Foliages, seeds and pods of tropical trees and shrubs have been considered as important components of ruminant rations long ago (Topps, Reference Topps1992; Shelton and Dalzell, Reference Shelton and Dalzell2007). Secondary compounds contained in such plant species exert different effects on the rumen microbial population (Patra and Saxena, Reference Patra and Saxena2009). Piñeiro-Vázquez et al. (Reference Piñeiro-Vázquez, Canul-Solis, Jiménez-Ferrer, Alayón-Gamboa, Chay-Canul, Ayala-Burgos, Aguilar-Pérez and Ku-Vera2018) reported that foliage of the tropical legume Leucaena leucocephala induced a reduction in methane yield when it was included in ration DM in high proportions (40% to 80%) in crossbred heifers (Bos indicus × Bos taurus) housed in respiration chambers that were fed a basal ration of low-quality tropical grass (Table 2). However, the increase in CP intake from L. leucocephala induced higher N excretion (as urea) in the urine, probably leading to higher nitrous oxide emissions (Montoya-Flores et al., Reference Montoya-Flores, Molina-Botero, Arango, Romano-Muñoz, Solorio-Sánchez, Aguilar-Pérez and Ku-Vera2020). This agrees with data reported by Harrison et al. (Reference Harrison, McSweeney, Tomkins and Eckard2015) who also found a reduction in enteric methane emissions, although nitrous oxide emissions increased in cattle fed Leucaena leucocephala in Australia. In a set of experiments involving the incorporation of foliages such as Leucaena leucocephala or Gliricidia sepium (Molina-Botero et al., Reference Molina-Botero, Arroyave-Jaramillo, Valencia-Salazar, Barahona-Rosales, Aguilar-Pérez, Ayala Burgos, Arango and Ku-Vera2019a; Montoya-Flores et al., Reference Montoya-Flores, Molina-Botero, Arango, Romano-Muñoz, Solorio-Sánchez, Aguilar-Pérez and Ku-Vera2020) or ground pods of Enterolobium cyclocarpum or Samanea saman (Lazos-Balbuena, Reference Lazos Balbuena2015; Valencia Salazar et al., Reference Valencia Salazar, Piñeiro Vázquez, Molina Botero, Lazos Balbuena, Uuh Narváez, Segura Campos, Ramírez Avilés, Solorio Sánchez and Ku Vera2018), a reduction in enteric methane yield of different magnitudes was recorded in cattle housed in respiration chambers (Table 2).
Table 2 Methane production, methane yield and methane conversion factor (Y m) in cattle fed tropical plants for enteric methane mitigation as measured in respiration chambers
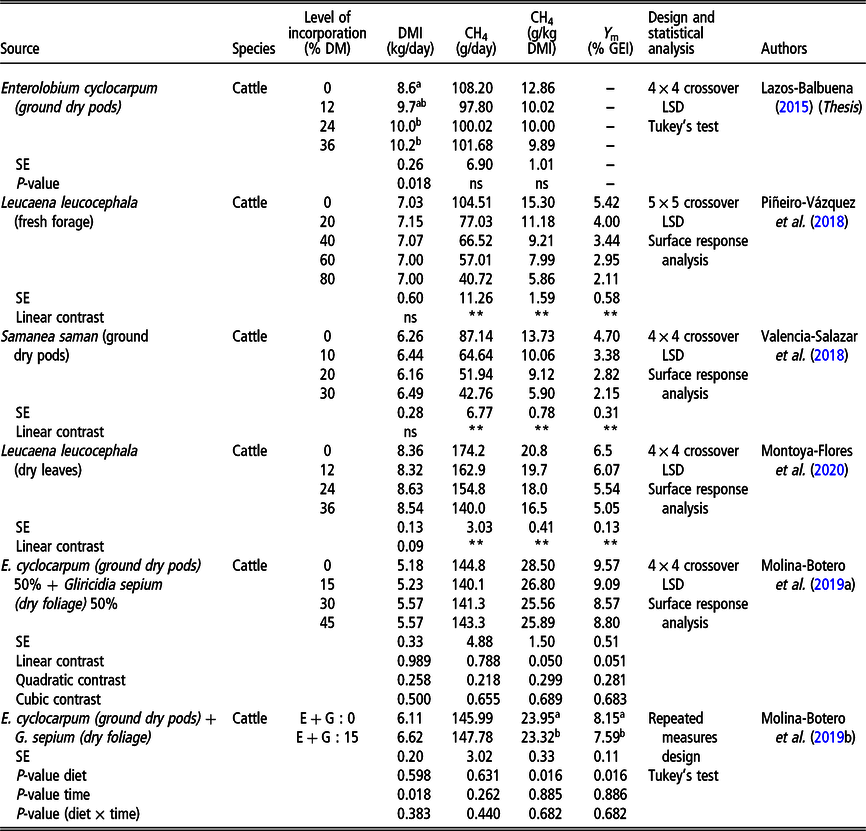
CH4 = methane; DMI = DM intake; Y m = CH4 loss expressed as percentage of gross energy intake (GEI); LSD = Latin square design; ns = non-significant (P > 0.05).
a,bMeans in the same column with different superscript are statistically different according to Tukey’s test (P > 0.05) (for experiments that used Tukey’s test).
**P < 0.01.
Pods of tropical legumes such as Enterolobium cyclocarpum and Acacia pennatula contain either condensed tannins or saponins, and when fed ground at 45% of ration DM, weight gains of up to 240 g/lamb per day were obtained in small-scale sheep farms (Briceño-Poot et al., Reference Briceño-Poot, Ruiz-González, Chay-Canul, Ayala-Burgos, Aguilar-Pérez, Solorio-Sánchez and Ku-Vera2012). Pods from those legumes can be hand collected by smallholders, stored in bags, ground and directly fed to cattle and sheep up to 50% of ration DM (Briceño-Poot et al., Reference Briceño-Poot, Ruiz-González, Chay-Canul, Ayala-Burgos, Aguilar-Pérez, Solorio-Sánchez and Ku-Vera2012). Pods are palatable and they are eagerly consumed by cattle and sheep after falling from the trees during the dry season. Rumen DM degradability of ground pods is high: 87% for Enterolobium cyclocarpum (Piñeiro-Vázquez et al., Reference Piñeiro-Vázquez, Ayala-Burgos, Chay-Canul and Ku-Vera2013) and 80% for Samanea saman (Valencia Salazar et al., Reference Valencia Salazar, Piñeiro Vázquez, Molina Botero, Lazos Balbuena, Uuh Narváez, Segura Campos, Ramírez Avilés, Solorio Sánchez and Ku Vera2018); they provide (through anaerobic fermentation) the necessary ATP for the maintenance and growth of bacteria in the rumen. The high level of rumen DM degradation of tropical pods suggests that they contain a high concentration of readily available carbohydrates (i.e. pectins, starch), which may change the pattern of rumen fermentation (Valencia Salazar et al., Reference Valencia Salazar, Piñeiro Vázquez, Molina Botero, Lazos Balbuena, Uuh Narváez, Segura Campos, Ramírez Avilés, Solorio Sánchez and Ku Vera2018) and decrease methane production per kg of DM consumed. Table 2 shows the magnitude of reduction in enteric methane yield when cattle were fed foliage or pods from tropical trees. Both options are simple and straightforward strategies for reducing methane emissions in farms under smallholder conditions in the tropics.
The proportion of gross energy intake (GEI) lost as methane gas: Y m (Table 2) ranged from 5.0% to 9.5% throughout the experiments, and it generally decreased as the levels of mitigating compounds (i.e. foliage, pods) were incorporated at increasing levels in the rations. Those authors found that Y m fluctuated between 4.8% and 13.7% and that IPCC (2006) procedures underestimated Y m by ~26.1%. Therefore, the derivation of precise Y m values remains an important challenge for estimating CH4 emissions from Bos indicus type of cattle fed low-quality forages in tropical developing countries. In Thailand, Chaokaur et al. (Reference Chaokaur, Nishida, Phaowphaisal and Sommart2015) working with Brahman cattle fed Pangola (Digitaria eriantha) grass as basal ration found that Y m ranged between 7.3% and 11.5% (above the IPCC (2006) default value of 6.5%) and that enteric methane emissions were linearly increased from the maintenance level of feeding up to ad libitum intake.
There are several possible explanations for the reduction of enteric methane emissions as a result of feeding foliage and pods of tropical plant species to cattle and sheep (see Figure 1). Condensed tannins contained in such plants may reduce CH4 in two different ways, first, by directly inhibiting methanogenic archaea, and second by decreasing H2 availability in the rumen and the apparent digestibility of DM and protozoal population (Patra et al., Reference Patra, Park, Kim and Yu2017; Piñeiro-Vázquez et al., Reference Piñeiro-Vázquez, Canul-Solis, Jiménez-Ferrer, Alayón-Gamboa, Chay-Canul, Ayala-Burgos, Aguilar-Pérez and Ku-Vera2018), Aboagye (Reference Aboagye, Oba, Castillo, Koenig, Iwaasa and Beauchemin2018 and Reference Aboagye, Oba, Koenig, Zhao and Beauchemin2019) reported a reduction in fiber digestibility, rather than a decrease in the amount of structural carbohydrates. Nonetheless, Canul-Solís et al. (Reference Canul-Solís, Piñeiro-Vázquez, Briceño-Poot, Chay-Canul, Alayón-Gamboa, Ayala-Burgos, Aguilar-Pérez, Solorio-Sánchez, Castelán-Ortega and Ku-Vera2014) found no effect of saponins (6 g/day) of Yucca schidigera on methane emissions of hair sheep fed low-quality tropical grass; this could be associated with the lack of effect on methanogenic archaea or the relatively low levels of incorporation of the mitigating commercial compound.
Saponins in pods such as those in Samanea saman may form complexes with sterols in the protozoal membrane surface and induce lysis of such membrane (Anantasook et al., Reference Anantasook, Wanapat and Cherdthong2014), thus reducing the population of methanogenic archaea which are usually associated with protozoa. Incorporation of foliage and pods of plants containing condensed tannins and steroidal saponins in ruminant rations can increase the molar proportion of propionic acid in rumen liquor and decrease the availability of metabolic H which is the specific substrate for carbon dioxide reduction to methane (Valencia-Salazar et al., Reference Valencia Salazar, Piñeiro Vázquez, Molina Botero, Lazos Balbuena, Uuh Narváez, Segura Campos, Ramírez Avilés, Solorio Sánchez and Ku Vera2018). Feeding of starch-containing ingredients in the ration such as in the pods of the legume tree Samanea saman (rain tree) may also drive a reduction in methane emissions by changing the pattern of rumen fermentation, increasing the molar proportion of propionic acid and lowering that of acetic acid in the rumen (Valencia-Salazar et al., Reference Valencia Salazar, Piñeiro Vázquez, Molina Botero, Lazos Balbuena, Uuh Narváez, Segura Campos, Ramírez Avilés, Solorio Sánchez and Ku Vera2018). Anantasook et al. (Reference Anantasook, Wanapat and Cherdthong2014) similarly reported an increase in propionic acid concentration in the rumen and a reduction in methane production, when they fed pods of Samanea saman to dairy steers fed urea-treated rice straw as basal ration. Ground pods of Samanea saman have been successfully used for feeding dairy cows (Anantasook et al., Reference Anantasook, Wanapat and Cherdthong2014) in Thailand. Changes in the molar proportions of VFA in the rumen (i.e. increasing propionic acid relative to acetic acid) may improve the efficiency of metabolizable energy utilization by decreasing heat increment and increasing energy retention as protein and fat synthesis (weight gain) in the body (NASEM, 2016) or in milk as fat and lactose. Thus, this kind of mitigation strategy may be more acceptable to smallholders, since they would see their effort compensated in both productivity and probably in financial revenues.
An increase in animal productivity has been observed under production conditions in cattle and sheep in small farms where both leucaena and pod legume incorporation in practical rations were implemented. Growing crossbred bulls showed a daily weight gain of 770 g, while grazing in a silvopastoral system with Leucaena leucocephala (Mayo-Eusebio, Reference Mayo-Eusebio2013) and confined hair sheep gained 240 g/day when pods of Enterolobium cyclocarpum were incorporated at 50% (DM basis) of their ration (Briceño-Poot et al., Reference Briceño-Poot, Ruiz-González, Chay-Canul, Ayala-Burgos, Aguilar-Pérez, Solorio-Sánchez and Ku-Vera2012) under smallholder farming conditions. These results agree with those of Harrison et al. (Reference Harrison, McSweeney, Tomkins and Eckard2015) with grazing cows on leucaena pastures in Australia and Moscoso et al. (Reference Moscoso, Vélez, Flores and Agudelo1995) with confined sheep fed ground pods of Enterolobium cyclocarpum (36% of ration DM) in Honduras (Central America). We do not fully understand the lack of changes in terms of the rumen microbial population when using tropical feedstuffs containing secondary compounds. So far, the work carried out on DNA (qPCR) techniques for counting the rumen microbial population has been inconclusive with regard to changes in the composition of the microbial population. No changes have been observed so far in the structure of the rumen population when cattle were fed either Leucaena leucocephala or Enterolobium cyclocarpum. Nonetheless, the length of time of feeding apparently does not lead to an adaptation of the rumen microbial population to secondary compounds (such as the saponins contained in pods of Enterolobium cyclocarpum) (Molina-Botero et al., Reference Molina-Botero, Montoya-Flores, Zavala-Escalante, Barahona-Rosales, Arango and Ku-Vera2019b). Disposal of H2 in the rumen through methanogenesis is aimed at maintaining the partial rumen pressure of H2 low so that fermentation can proceed efficiently. So far, there is still not a plausible explanation for the fate of the excess metabolic H as a result of methane mitigation in ruminants fed tropical C4 grasses (4 mol H2 remains unused per mol CH4 decreased; Hegarty and Gerdes, Reference Hegarty and Gerdes1999). No alternative H2 sink has been proposed as yet, although it is recognized that there may be an excess H2 in the rumen when methane mitigating compounds are fed (up to 35.9 g/day in heifers; Romero-Perez et al. (Reference Romero-Perez, Okine, McGinn, Guan, Oba, Duval, Kindermann and Beauchemin2015)). Some of the excess H2 in the treatments involving incorporation of mitigating compounds may have been incorporated into rumen microbes (Kennedy and Charmley, Reference Kennedy and Charmley2012). Nevertheless, more work has to be done on this subject. Rumen pH of cattle fed foliages and pods of tropical plants where substantial reductions in methane emissions have been recorded showed no apparent changes 4 h after feeding (Valencia-Salazar et al., Reference Valencia Salazar, Piñeiro Vázquez, Molina Botero, Lazos Balbuena, Uuh Narváez, Segura Campos, Ramírez Avilés, Solorio Sánchez and Ku Vera2018; Montoya-Flores et al., Reference Montoya-Flores, Molina-Botero, Arango, Romano-Muñoz, Solorio-Sánchez, Aguilar-Pérez and Ku-Vera2020). Since rumination is normally carried out in such conditions, rumen pH generally remains within the physiological range (6 to 7), as well as the osmotic pressure (i.e. 250 to 300 mosmol/kg).
Essential oils are secondary compounds (alcohol, ester or aldehyde derivatives of phenylpropanoids and terpenoids) present in some tropical plants. They are volatile, aromatic substances. Essential oils display antimicrobial actions related to processes in the bacterial cell membrane involving electron transport, ion gradients, phosphorylation and protein translocation (Benchaar et al., Reference Benchaar, Calsamiglia, Chaves, Fraser, Colombatto, McAllister and Beauchemin2008). Essential oils exert a high affinity for lipids in cell membranes of bacteria which results from their hydrophobic nature and their antibacterial actions are related to their lipophilic character; thus, they can disrupt the cytoplasmic membrane either directly or by damaging proteins in the membrane, resulting in increased permeability of the membrane and then leakage of cytoplasmic constituents thus affecting the proton motive force (Hart et al., Reference Hart, Yañez-Ruiz, Duval, McEwan and Newbold2008). Scientific evidence on the potential of EOs to reduce enteric methane in ruminants in vivo in tropical regions is still scarce (Wang et al., Reference Wang, Liu, Guo, Huo, Ma, Zhang, Pei, Zhang and Wang2018). Effective doses, mode of action, voluntary intake and the cost-benefit ratio must be assessed. Most of the studies involving EOs have been conducted under in vitro conditions (Ye et al., Reference Ye, Karnati, Wagner, Firkins, Eastridge and Aldrich2018) and those results must be validated in vivo at the farm level under smallholder conditions. Recent work, carried out at the University of Yucatan in Mexico, with crossbred heifers held in respiration chambers (Jiménez-Ocampo, R. unpublished results) has shown that EO from Citrus sinensis (0.5% DM) reduced methane yield in heifers fed low-quality tropical grasses by up to ~18% (compared to a control ration without oil), which agrees with experiments carried out in Hu sheep supplemented with EO from grapefruit peels (Wu et al., Reference Wu, Koerkamp and Ogink2018). Figure 1 shows the mechanisms of action of some secondary compounds for mitigation of enteric methane in ruminants. The mechanisms are rather different: condensed tannins may both affect feed digestibility and inhibit archaea, saponins may disrupt protozoa membranes and reduce the population of methanogenic archaea, while EOs may affect protozoa and methanogens, depending on the type and concentration. In the tropics, there is a wide range of plants which may induce such mechanisms in the rumen of cattle under practical feeding conditions.
Nitrate supplementation as a methane mitigation strategy
Nitrate (NO3−) has been proposed as a replacement for urea (non-protein N) as a source of rumen degradable N for the rumen microbes (Lee and Beauchemin, Reference Lee and Beauchemin2014). Therefore, nitrate could work well under dry season cattle farming conditions in the tropics when grasses are characterized by deficient N concentration for optimal microbial growth supplying N essential for adequate bacterial protein synthesis (Leng, Reference Leng1990). Nitrate is an alternative H sink which competes with methane synthesis; the stoichiometric potential of 1 mol of nitrate can reduce methane synthesis by 1 mol (Leng, Reference Leng2008) and nitrate can decrease the number of rumen protozoa resulting in CH4 reduction. Nitrate reduction in the rumen to nitrite and then to NH3 is energetically more favorable than carbon dioxide reduction for methane synthesis, which implies that 4 mol H2 is redirected toward nitrate reduction. Therefore, methane production can be mitigated by 1 mol per each mole of nitrate reduced, which is equivalent to a CH4 emission reduction of 25.8/100 g nitrate fed. However, high levels of nitrate in the rumen may induce toxicity, as nitrate is transformed into nitrite and an excess may induce an increase in methemoglobin (MetHb) in the bloodstream, leading to hypoxia, dyspnea and even death if the animal is not treated immediately (Callaghan et al., Reference Callaghan, Tomkins, Benu and Parker2014). Nitrate toxicity and its potentially hazardous consequences can be avoided by supplying less than 9 g NO3−/kg DM intake in cattle, but the concentrations of basal NO3− in the consumed grass must also be accounted for.
Concentrations of NO3− are related to plant species, phenological stage and other plant stressors (e.g. drought, fertilization). According to Callaghan et al. (Reference Callaghan, Tomkins, Benu and Parker2014), in Northern Australia they recommended ~15 g/day of NO3− in breeding beef cows grazing dry season pastures. Nonetheless, there is a risk in the control of the intake of the supplement and the heterogeneous structure of herds of grazing cattle with different feed intakes. An experiment was carried out to evaluate the effect of dietary nitrate on enteric methane emissions in dairy cows grazing pastures during the summer and it was found that 8 g NO3−/kg DM in grazing dairy cows led to a decrease in CH4 emissions (van Wyngaard et al., Reference van Wyngaard, Meeske and Erasmus2018). Hulshof et al. (Reference Hulshof, Berndt, Gerrits, Dijkstra, van Zijderveld, Newbold and Perdok2012) using sugarcane-based rations reported that 22 g NO3−/kg DM reduced enteric methane emission by 32%.
In the dry season, tropical grasses usually contain low concentrations of CP and nitrates could supply the N required for improving rumen fermentation of low-quality basal rations. A gradual adaptation period of 15 days and the use of slow-release NO3− in the ration are alternative ways of reducing the risk of toxicity (Alemu et al., Reference Alemu, Romero-Pérez, Araujo and Beauchemin2019). El-Zaiat et al. (Reference El-Zaiat, Araujo, Soltan, Morsy, Louvandini, Pires, Patino, Correa and Abdalla2014) showed that encapsulated nitrate was not toxic to lambs (4.51% encapsulated nitrate product (60.83% nitrate in the product DM)). At the same time, those authors identified higher risks of toxicity in rations with low levels of readily fermented carbohydrates. For that reason, a practice that reduces feeding problems is to supply non-structural carbohydrates in the ration, as grains (i.e. starch) which appear to exert some prophylactic effect. Nitrates can be offered twice a day or mixed with molasses in roller drums to reduce MetHb synthesis (Benu et al., Reference Benu, Callaghan, Tomkins, Hepworth, Fitzpatrick and Parker2016). However, those practices are complicated in pastoral systems in the tropics, since forage quality varies with season. Villar et al. (Reference Villar, Hegarty, Nolan, Godwin and McPhee2020) found that mature steers fed a basal ration of lucerne chaff and supplemented with a mixture of NO3− and canola oil reduced daily methane production by 26%, which suggested a synergistic effect of the two mitigating compounds, when combined.
Recent work, conducted at the University of Yucatan-Mexico, with crossbred heifers (Bos indicus × Bos taurus) housed in open-circuit respiration chambers showed that supplementation with increasing levels of NO3− (0.1%, 0.2% and 0.3% DM) to a basal ration of low-quality chopped tropical grass (Pennisetum purpureum) resulted in a substantial reduction in enteric CH4 emissions from 135.5 g/day (0% NO3−) to 93.3 g/day (with 0.3% NO3− DM), although a reduction in DM intake was also noticed. Nitrate seems to be a viable methane mitigating additive which simultaneously supplies N to the rumen microorganisms during the dry season when N concentration in tropical grasses is deficient for optimal microbial protein synthesis in the rumen.
Lipid supplementation as a methane mitigation strategy
Lipid sources as a methane mitigation strategy under tropical conditions (rations with high NDF and low digestibility and intake) can play an important role in the improvement of cattle performance and therefore in farm productivity. In their comprehensive review, Grainger and Beauchemin (Reference Grainger and Beauchemin2011) suggested that the mitigation potential of fats is 1 g CH4/kg DM intake for each 10 g fat/kg DM in the ration and this has shown persistent results, although no effect of the chain length of the fatty acids on the magnitude of mitigation has been detected. Feeding sunflower oil can effectively reduce enteric methane emissions of dairy cows (Bayat et al., Reference Bayat, Ventto, Kairenius, Stefański, Leskinen, Tapio, Negussie, Vilkki and Shingfield2017). Similar effects of vegetable oils on reducing methane emissions have been reported under in vitro conditions (Vargas et al., Reference Vargas, Andrés, López-Ferreras, Snelling, Yáñez-Ruíz, García-Estrada and López2020). Recent work carried out at the University of Yucatan, Mexico (Flores-Santiago, E. unpublished results), has shown that by feeding palm oil (6% of ration DM) to hair Pelibuey sheep housed in respiration chambers, which were fed a low-quality basal ration of tropical C4 grass (Brachiaria brizantha) hay, methane yield was reduced by ~14%, compared to a control treatment without palm oil. Palm oil contains saturated fatty acids (50%; palmitic acid: 44% and stearic acid: 5%) and unsaturated fatty acids (oleic acid: 40% and polyunsaturated linoleic and α-linoleic acid: 10%). Palm oil is available for ruminant feeding in several Latin American and other tropical countries.
A number of experiments have been carried out in Brazil with grazing cattle supplemented with diverse sources of lipids. Mata e Silva et al. (Reference Mata e Silva, Lopes, Pereira, Tomich, Morenz, Martins, Gomide, Paciullo, Maurício and Chaves2017) found that sunflower oil (383 g/day) given to lactating dairy cows grazing a tropical pasture (Urochloa brizantha) decreased enteric CH4 by ~23%. Neto et al. (Reference Neto, Messana, Ribeiro, Vito, Rossi and Berchielli2015) reported that grazing Nellore bulls supplemented with a concentrate containing oil was an effective means for reducing enteric methane emissions as a percentage of GEI by 12.8%. Similarly, Carvalho et al. (Reference de Carvalho, Fiorentini, Berndt, Castagnino, Messana, Frighetto, Reis and Berchielli2016) supplemented Nellore steers grazing Brachiaria brizantha with linseed oil and found a reduction in enteric methane emissions. In Nellore bulls grazing Brachiaria brizantha supplemented with starch, the use of oil (~198 g soybean grain/kg DM) reduced enteric CH4 emissions (Neto et al., Reference Neto, Messana, Rossi, Carvalho and Berchielli2019).
Practical implications of methane mitigation in tropical cattle systems
There is a shortage of information on enteric methane emissions from dairy and beef cattle production systems in the tropics (Bartl et al., Reference Bartl, Gómez and Nemecek2011; Niu et al., Reference Niu, Kebreab, Hristov, Oh, Arndt, Bannink, Bayat, Brito, Boland, Casper, Crompton, Dijkstra, Eugène, Garnsworthy, Haque, Hellwing, Huhtanen, Kreuzer, Kuhla, Lund, Madsen, Martin, McClelland, McGee, Moate, Muetzel, Muñoz, O’Kiely, Peiren, Reynolds, Schwarm, Shingfield, Storlien, Weisbjerg, Yáñez-Ruiz and Yu2018). Enteric CH4 mitigation in small-scale cattle farms may be a rather straightforward practice, from a purely technical standpoint. For instance, if it is assumed that crossbred (Bos indicus × Bos taurus) dual-purpose cows grazing on silvopastoral systems in the tropics consume ~30% of total daily DM intake (measured with the n-alkane technique) as Leucaena leucocephala (Bottini-Luzardo et al., Reference Bottini-Luzardo, Aguilar-Pérez, Centurión-Castro, Solorio-Sánchez and Ku-Vera2016), and by using the results obtained by Montoya-Flores et al. (Reference Montoya-Flores, Molina-Botero, Arango, Romano-Muñoz, Solorio-Sánchez, Aguilar-Pérez and Ku-Vera2020) who found a reduction in CH4 yield of ~20% in crossbred cows fed Leucaena leucocephala (36% DM; measured in respiration chambers). If those data are combined and extrapolated as a first approximation, it follows that the actual mitigation of enteric CH4 emissions in silvopastoral systems with leucaena may possibly range from ~15% to ~20%, compared to an only-grass, monoculture system. In dual-purpose cattle production systems in the tropics, average milk yield per cow usually ranges between 7 and 10 litres/day, and there is an urgent need to increase productivity. Under those conditions, financial feasibility improves in the silvopastoral systems because the farmer relies less on imported (expensive) grains (i.e. concentrates) when using locally available high-quality forages such as leucaena (Bottini-Luzardo et al., Reference Bottini-Luzardo, Aguilar-Pérez, Centurión-Castro, Solorio-Sánchez and Ku-Vera2016; Kumari et al., Reference Kumari, Fagodiya, Hiloidhari, Dahiya and Kumar2020). Reviewed data clearly show the range of methane yields (16.1 to 34.1 g CH4/kg DM intake) which can be expected from cattle fed tropical grasses, with values at the lower end, probably reflecting the slow rate and low extent of fermentation of organic matter in the rumen (i.e. dry season grasses). Suybeng et al. (Reference Suybeng, Charmley, Gardiner, Malau-Aduli and Malau-Aduli2019) have advanced the potential of Desmanthus leptophyllus as a promising legume for methane mitigation and improvement of cattle productivity under tropical grazing conditions in Australia.
Strategies based on the incorporation of natural resources such as vegetable oils, foliages, pods and seeds of tropical plants in the ration represent an approach for enteric CH4 mitigation in ruminants in the vast areas of land covered with low-quality tropical grasses. Recently, Ali et al. (Reference Ali, Wassie, Korir, Merbold, Goopy, Butterbach-Bahl, Dickhoefer and Schlecht2019b) observed that when Holstein × Boran heifers were fed a basal ration of low-quality Rhodes hay and supplemented with sweet potato vine silage or a urea-molasses block, enteric CH4 emissions were lowered while cattle were kept in respiration chambers in Kenya. In developing countries, it is important to work more intensively on technology transfer (extension) and farmer organization (cooperatives) in order to realize actual changes in cattle production, by introducing technologies with low environmental impact through the mitigation of GHG emissions such as CH4 and N2O. It is likely that in the near future it will be necessary to reach decreases in net methane emissions of cattle (g CH4/head per day), to comply with international agreements (United Nations Framework Convention on Climate Change) where commitments towards absolute reductions in national GHG inventories have been signed by governments (Beauchemin et al., Reference Beauchemin, Ungerfeld, Eckard and Wang2020).
At low levels of nutrition of cattle, a situation which is common in the tropics during the dry season, it has been claimed that the slow rate of passage of solid digesta through the rumen may play a role in methane emissions (Ali et al., Reference Ali, Wassie, Korir, Goopy, Merbold, Butterbach-Bahl, Dickhoefer and Schlecht2019a), implying that if solid digesta is retained for a long time in the rumen, methane emissions may increase. These data have obvious implications for methane inventories from tropical, developing countries, if this is substantiated further. In the event of that information being validated by further research, it would be rather easy to increase passage rate of digesta by feeding highly rumen fermentable forages (i.e. Brosimum alicastrum, Leucaena leucocephala, Gliricidia sepium and Desmanthus spp.) which have been proven to stimulate DM intake and thus passage rate in ruminants. In developing tropical countries, reduction of methane emissions in cattle by decreasing retention time of digesta in the rumen seems an achievable goal in the short-term, simply by supplementing highly rumen fermentable forages.
Productivity is expected to increase to some extent as a result of rechanneling energy (H2) saved from CH4 synthesis into protein and fat stored in the body or secreted in milk (van Zijderveld et al., Reference van Zijderveld, Gerrits, Dijkstra, Newbold, Hulshof and Perdok2011). Indeed, some tropical plants (i.e. pods of Samanea saman; Valencia Salazar et al., Reference Valencia Salazar, Piñeiro Vázquez, Molina Botero, Lazos Balbuena, Uuh Narváez, Segura Campos, Ramírez Avilés, Solorio Sánchez and Ku Vera2018) with mitigation potential may be particularly useful for significantly reducing enteric CH4 production in cattle fed basal rations of low-quality grasses. Greening et al. (Reference Greening, Geier, Wang, Woods, Morales, McDonald, Rushton-Green, Morgan, Koike, Leahy, Kelly, Cann, Attwood, Cook and Mackie2019) have advanced the concept that the increase in animal productivity while mitigating CH4 emissions relies on the control of H2 use by methanogenic archaea. It seems then that redirection of H2 flux by manipulating rumen fermentation can increase animal production by recovering energy that would be otherwise lost through methane synthesis in the rumen. This is a subject which requires further attention by animal scientists in the tropics, along with work on kinetics of digesta passage through the rumen (Ali et al., Reference Ali, Wassie, Korir, Goopy, Merbold, Butterbach-Bahl, Dickhoefer and Schlecht2019a), since mean retention time of solid digesta in the rumen is expected to contribute in a given extent to animal variation in feed efficiency (Cantalapiedra-Hijar et al., Reference Cantalapiedra-Hijar, Abo-Ismail, Carstens, Guan, Hegarty, Kenny, McGee, Plastow, Relling and Ortigues-Marty2018).
Conclusions
Foliages, pods and seeds of tropical trees and shrubs containing either condensed tannins, EOs, saponins or starch, as well as other compounds such as nitrates and by-products such as vegetable oils can be fed to cattle to mitigate enteric methane emissions under smallholder conditions. Methane yields can be reduced by approximately 10% to 25% by adopting those practices in ruminant production systems. Silvopastoral systems with high-quality legumes (i.e. leucaena, desmanthus), intensification of grazing management (i.e. rotatinuous) and supplementation with nutritional blocks containing CH4 depressors (pods of Enterolobium cyclocarpum or Samanea saman or seeds of Brosimum alicastrum) are practical options available to smallholders for mitigating enteric methane emissions in cattle raised under tropical conditions.
Acknowledgements
A preliminary version of this paper was published in an abstract form at the 7th Greenhouse Gas and Animal Agriculture Conference 2019, held at Iguassu Falls, Brazil. We are indebted to CONACYT-Mexico for granting fellowships to students to carry out MSc and PhD studies, as well as postdoctoral research at the University of Yucatan, Mexico. We thank the State Government of Yucatan (SIIDETEY)-Mexico for financial support to keep the respiration chambers running. We are grateful to CONACYT-Mexico for support (CB: A1-S-23910; Convocatoria 2018-1) to carry out experiments on energy metabolism of cattle. This work was implemented as part of the CGIAR Research Programs on Livestock and Climate Change, Agriculture and Food Security (CCAFS), which are carried out with support from CGIAR Fund Donors and through bilateral funding agreements. For details please visit https://ccafs.cgiar.org/donors. The views expressed in this document cannot be taken to reflect the official opinions of these organizations. We thank all donors that globally support our work through their contributions to the CGIAR system. We are indebted to the anonymous referees of this paper, for their kind suggestions towards its improvement.
R. Jiménez-Ocampo 0000-0003-2171-1357
Declaration of interest
The authors declare no conflict of interest.
Ethics statement
The authors did not use any live animal to conduct this review.
Software and data repository resources
None of the data were deposited in an official repository.