Implications
Inflammatory signals are elevated in dairy cows in the days around parturition, but these signals are not always pathological. Physiological roles of inflammatory mediators may include directing metabolic shifts that enable adaptation to lactation, although excessive elevation of these signals likely has net negative impacts on nutrient availability through suppression of appetite and activation of immune responses. The management of inflammatory tone through dietary or pharmacological means may reduce periparturient disorders while enhancing productivity in dairy cattle.
Introduction
In dairy cattle, the transition to lactation represents a perilous time period when the incidence of disease is high. This increase in disease incidence is associated with a chronic inflammatory response (Sordillo and Raphael, Reference Sordillo and Raphael2013). At the onset of lactation, a depression of feed intake occurs simultaneously with an increase in energy demand, resulting in metabolic stress. Low-grade inflammation has been associated with metabolic and infectious diseases in early-lactation cattle (Bradford et al., Reference Bradford, Yuan, Farney, Mamedova and Carpenter2015), and therefore, the metabolic effects of inflammation have been the focus of much research in recent years. An accelerating field of research has now demonstrated that immune cells are directly involved in a surprising array of metabolic functions (Man et al., Reference Man, Kutyavin and Chawla2017), including the maintenance of gastrointestinal function (Ferreira et al., Reference Ferreira, Forsyth, Richman, Wells, Spencer and MacDonald1990; Hoytema van Konijnenburg et al., Reference Hoytema van Konijnenburg, Reis, Pedicord, Farache, Victora and Mucida2017), control of adipose tissue lipolysis (Kosteli et al., Reference Kosteli, Sugaru, Haemmerle, Martin, Lei, Zechner and Ferrante2010) and regulation of insulin sensitivity in multiple tissues (Olefsky and Glass, Reference Olefsky and Glass2010). In fact, the immune system is so deeply embedded in metabolic physiology that some suggest that the liver, adipose tissue and the immune system developed from a single ancestral organ (Hotamisligil, Reference Hotamisligil2006). In recent years, the vast majority of research on low-grade inflammation associated with metabolic disorders, also termed metabolic inflammation, has focused on human diseases that are, in part, initiated by inflammatory signals, including diabetes, hepatic steatosis and atherosclerosis (Medzhitov, Reference Medzhitov2008). Recent advancements in bovine research have also illuminated the intersection of immune function and metabolism. Yet, many questions remain, including:
Are there adaptive roles for metabolic inflammation during the transition to lactation?
Is metabolic inflammation homeostatically regulated, and if so, what are the mechanisms behind this regulation?
If these mechanisms are manipulated, what are the potential benefits and consequences?
How can this information be harnessed on-farm to advance dairy cattle health and productivity?
Several recent reviews have provided updates on the role of immune cells in gastrointestinal tissue of cattle and downstream impacts of their activation, including on hepatic function (Steele et al., Reference Steele, Penner, Chaucheyras-Durand and Guan2016; Garcia et al., Reference Garcia, Bradford and Nagaraja2017; Khiaosa-Ard and Zebeli, Reference Khiaosa-Ard and Zebeli2018). Therefore, this review presents some targeted perspectives, rather than an exhaustive overview, on adipose tissue and inter-organ integration of inflammatory signals. We focus on the concept of inflammatory tone, which we define as the regulatory process to strike a balance between immune activation and tolerance.
Immune cells perform an intricate inflammatory balancing act in adipose tissue
The mechanisms underlying sentinel functions of the immune system have become far better described in the past decade, particularly with respect to the need to strike an appropriate balance between immune tolerance and responsiveness to microbial or damage-associated signals. In the gut especially, this balance is critical to health, even in the absence of a true pathogenic challenge (Chistiakov et al., Reference Chistiakov, Bobryshev, Kozarov, Sobenin and Orekhov2015). One of the primary ways that this balance is achieved is through immune cross-talk in the gut, primarily utilizing pro- and anti-inflammatory cytokines to regulate proliferation and activity of specific immune cells (Omenetti and Pizarro, Reference Omenetti and Pizarro2015). This regulatory process, which we refer to as inflammatory tone, is clearly critical to gut homeostasis. An important question is whether inflammatory tone is similarly important for organs that are not protecting an epithelial surface. Although the need for tolerance is perhaps less obvious in tissues that are not routinely exposed to commensal microbiota (Desruisseaux et al., Reference Desruisseaux, Nagajyothi, Trujillo, Tanowitz and Scherer2007), there is strong evidence that maintaining a balance between inflammatory and regulatory immune cells is critical for the metabolic health of adipose tissue and, in turn, the animal.
Immune cells resident in many tissues regulate nutrient use on a routine basis (Iyer et al., Reference Iyer, Brown, Whitehead, Prins and Fairlie2015) in addition to the broad systemic effects of cytokine storms that occur during disease. The mechanisms behind metabolic inflammation remain speculative in bovines; however, in rodents, the nexus of immune cells and metabolism are far better described (Figure 1). Tissue-resident macrophages play a central role in metabolic stress; and in obesity the proportion of macrophages in adipose tissue increases from approximately 10% of all cells to 50% (Weisberg et al., Reference Weisberg, McCann, Desai, Rosenbaum, Leibel and Ferrante2003, Lumeng et al., Reference Lumeng, Bodzin and Saltiel2007). These cells can be classified as either pro-inflammatory (M1) or anti-inflammatory (M2), although these classifications have limitations as macrophages exhibit immense plasticity in adipose tissue (Morris et al., Reference Morris, Singer and Lumeng2011). In obese mice, adipocyte hypertrophy triggers the invasion of M1 macrophages into adipose tissue. Some of these adipocytes will atrophy, resulting in the release of fatty acids which are engulfed by macrophages to prevent lipotoxicity. The net effect is a low-grade inflammatory tone due to the accumulation of M1 macrophages in crown-like structures, which also include necrotic adipocytes and lipid remnants (Bai and Sun, Reference Bai and Sun2015). However, in bovine, an overt inflammatory state was not found in subcutaneous adipose tissue of overfed non-pregnant non-lactating cows (Lopreiato et al., Reference Lopreiato, Hosseini, Rosa, Zhou, Alharthi, Trevisi and Loor2018), although a mildly inflammatory state was noted in mesenteric adipose tissue (Moisá et al., Reference Moisá, Ji, Drackley, Rodriguez-Zas and Loor2017). Moreover, contrary to observations from rodents, macrophages do not accumulate in crown-like structures in early-lactation dairy cows (Akter et al., Reference Akter, Häussler, Germeroth, Von Soosten, Dänicke, Südekum and Sauerwein2012; Contreras et al., Reference Contreras, Kabara, Brester, Neuder and Kiupel2015). Yet, a greater number of adipose tissue macrophages in diseased early-lactation dairy cattle (particularly M1 macrophages) was found compared to non-lactating dairy cows in addition to an increase in pro-inflammatory cytokine mRNA abundance, although it should be noted that there was also a smaller increase in the number of M2 macrophages (Contreras et al., Reference Contreras, Kabara, Brester, Neuder and Kiupel2015). When comparing late-lactation cows in either positive or negative energy balance, an increase in macrophage infiltration in adipose was noted in cows experiencing negative energy balance; however, there were minimal changes in inflammatory markers between groups (Contreras et al., Reference Contreras, Thelen, Schmidt, Strieder-Barboza, Preseault, Raphael, Kiupel, Caron and Lock2016). Lastly, an invasion of macrophages in adipose was associated with a greater degree of body condition loss in transition dairy cows (Newman et al., Reference Newman, Miller, Yepes, Bitsko, Nydam and Mann2019). Collectively, these data suggest a role for M1 macrophages in the development of a low-grade chronic inflammatory state that can occur in diseased early-lactation dairy cattle (Contreras et al., Reference Contreras, Strieder-Barboza and De Koster2018). In agreement with these findings, an increased mRNA abundance of the M1 cytokine, tumor necrosis factor-α (TNF-α), which is primarily produced by M1 macrophages, was found in adipose tissue from obese rats (Hotamisligil et al., Reference Hotamisligil, Shargill and Spiegelman1993) and postpartum dairy cattle (Sadri et al., Reference Sadri, Bruckmaier, Rahmani, Ghorbani, Morel and Van Dorland2010).
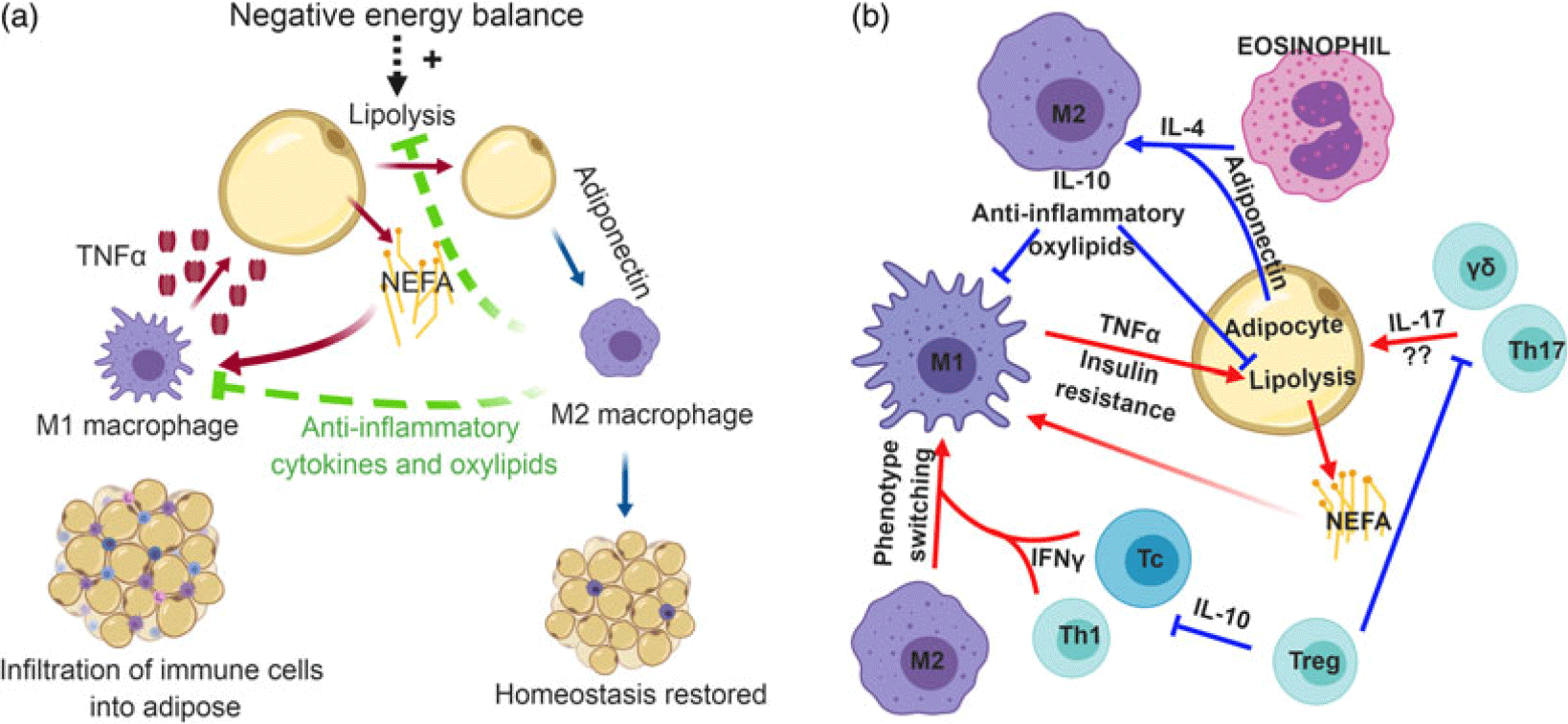
Figure 1 (Color online) Role of immune cells in mammalian adipose tissue. (a) Adipose tissue-resident macrophages exhibit a pro-inflammatory (M1) phenotype that is stimulated by the release of non-esterified fatty acids (NEFA) from adipocytes. In response to NEFA, M1 macrophages secrete the pro-inflammatory cytokine, tumor necrosis factor-α (TNF-α), which can further induce lipolysis. To maintain inflammatory tone, anti-inflammatory (M2) macrophages may be recruited into adipose tissue during negative energy balance. Adiponectin polarizes recruited macrophages toward an M2 phenotype, which produce anti-inflammatory cytokines and oxylipids to temper lipolysis and inflammation and restore homeostasis. (b) Immense cross-talk exists between a variety of immune cells to regulate inflammatory tone in adipose tissue. In obesity, cytotoxic T cells (Tc) and T helper 1 (Th1) cells in adipose tissue secrete interferon-γ (IFN-γ). This cytokine promotes phenotype switching of macrophages from an M2 phenotype to M1. Pro-inflammatory macrophages produce TNF-α, which induces insulin resistance. On the other hand, eosinophils produce interleukin-4 (IL-4) which synergizes with the anti-inflammatory adipose-derived cytokine, adiponectin, to promote M2 polarization of macrophages. This anti-inflammatory macrophage is thought to temper inflammatory responses in adipose tissue and slow lipolysis. Lastly, the role of IL-17-producing γδ T cells and Th17 cells are relatively unknown, but the production of IL-17 likely contributes to the chronic, low-grade inflammatory tone seen in metabolic inflammation. Regulatory T cells (Treg) can inhibit the polarization of pro-inflammatory immune cells, thereby promoting tissue homeostasis and self-tolerance. Panel B inspired by Chatzigeorgiou et al. (Reference Chatzigeorgiou, Karalis, Bornstein and Chavakis2012). Most studies underlying these relationships have been conducted with mice, but mechanisms are proposed to be conserved across mammalian species.
Monocytes are recruited to adipose tissue via chemoattractants, such as CC-chemokine ligand 2 (CCL2) and CCL5 (Mukesh et al., Reference Mukesh, Bionaz, Graugnard, Drackley and Loor2010), which are produced by adipocytes and M1 macrophages, and additional recruitment and invasion of M1 macrophages should aid in amplifying the inflammatory response (Chawla et al., Reference Chawla, Nguyen and Goh2011; Odegaard and Chawla, Reference Odegaard and Chawla2013). Considering these findings, one could expect a cascade of immune activation events snowballing into a pronounced inflammatory response. Yet, metabolic inflammation appears to have ‘one foot on the gas’ and simultaneously ‘one foot on the brake’, resulting in a smoldering, low-grade inflammatory tone. Thus, the question of how this inflammatory tone is maintained remains elusive to many researchers. It seems plausible that other immune cells are playing a critical role in maintaining a low-grade inflammatory tone in diseased cattle, preventing an aggressive immune response, and that these cells could underpin an anti-inflammatory adaptation that may occur in healthy early-lactation dairy cattle.
Eosinophils are granulocytes that are typically associated with allergy and asthma. In adipose tissue, eosinophils produce the anti-inflammatory cytokine, interleukin-4 (IL-4), that promotes differentiation of monocytes toward the IL-10 producing, M2 macrophage phenotype in mice (Wu et al., Reference Wu, Molofsky, Liang, Ricardo-Gonzalez, Jouihan, Bando, Chawla and Locksley2011). Interleukin-4 synergizes with adiponectin, an adipocyte-derived anti-inflammatory cytokine (Kabara et al., Reference Kabara, Sordillo, Holcombe and Contreras2014), to improve insulin sensitivity (Chawla et al., Reference Chawla, Nguyen and Goh2011), and IL-10 protects against TNF-α-induced insulin resistance (Lumeng et al., Reference Lumeng, Bodzin and Saltiel2007). As previously mentioned, CCL2 recruits monocytes, and knocking out the CCL2 receptor in obese mice increased the number of eosinophils in adipose tissue (Bolus et al., Reference Bolus, Gutierrez, Kennedy, Anderson-Baucum and Hasty2015). Taken together, these data suggest that during metabolic inflammation, CCL2 may play a critical role in tilting the balance toward a pro-inflammatory tone by not only recruiting M1 macrophages but also reducing the migration of anti-inflammatory eosinophils into adipose tissue. In dairy cattle, the role of eosinophils in adipose tissue has yet to be explored, but blood eosinophils are reduced in early-lactation cows (Holtenius et al., Reference Holtenius, Waller, Essen-Gustavsson, Holtenius and Sandgren2004), suggesting a need for additional research.
T lymphocytes are also key players in regulating inflammatory tone in adipose tissue. T cells can be classified into different subsets such as regulatory T cells (Treg), helper T cells (Th), cytotoxic T cells (Tc) and γδ T cells. Depending on the stimuli, T cells can be polarized into different phenotypes that are defined by the cytokines produced, and these cytokines exert either pro- or anti-inflammatory effects. For instance, pro-inflammatory interferon-γ (IFN-γ) drives immune responses that are particularly effective against intracellular microbes (type 1 response, Th1), whereas cytokines such as IL-4, IL-5 and IL-13 support antibody production from B cells, M2 polarization of macrophages and elimination of parasitic infections (type 2 response, Th2). Moreover, pro-inflammatory IL-17 induces neutrophil generation and migration (Th17), while IL-10 promotes tissue homeostasis and self-tolerance by suppressing the immune function (Treg). These cells serve to counterbalance each other. For instance, a pro-inflammatory Th1 cytokine is counterbalanced with the anti-inflammatory Th2 cytokine; and similarly, Th17 offsets Treg cells. Thus, the balance of these cells is critical for determining inflammatory tone.
In lean mice, immunosuppressive Treg cells, which produce IL-10, were highly enriched in adipose tissue, but not so in obese mice (Feuerer et al., Reference Feuerer, Herrero, Cipolletta, Naaz, Wong, Nayer, Lee, Goldfine, Benoist, Shoelson and Mathis2009). Indeed, obesity is characterized by the production of pro-inflammatory cytokines from the major T cell subsets, including cytotoxic T cells, T helper cells and γδ T cells (Feuerer et al., Reference Feuerer, Herrero, Cipolletta, Naaz, Wong, Nayer, Lee, Goldfine, Benoist, Shoelson and Mathis2009; Winer et al., Reference Winer, Paltser, Chan, Tsui, Engleman, Winer and Dosch2009; Mehta et al., Reference Mehta, Nuotio-Antar and Smith2015). In mice fed a high-fat diet, cytotoxic T cells promoted monocyte infiltration and inflammation in adipose tissue, and depletion of these T cells ameliorated insulin resistance (Nishimura et al., Reference Nishimura, Manabe, Nagasaki, Eto, Yamashita, Ohsugi, Otsu, Hara, Ueki, Sugiura, Yoshimura, Kadowaki and Nagai2009). More specifically, IFN-γ produced by Th1 and cytotoxic T cells promotes M1 macrophage differentiation, and more recent evidence has shown that this cytokine can also induce insulin resistance directly (Rocha et al., Reference Rocha, Folco, Sukhova, Shimizu, Gotsman, Vernon and Libby2008; McGillicuddy et al., Reference McGillicuddy, Chiquoine, Hinkle, Kim, Shah, Roche, Smyth and Reilly2009; O’Rourke et al., Reference O’Rourke, White, Metcalf, Winters, Diggs, Zhu and Marks2012; Khan et al., Reference Khan, Dai Perrard, Perrard, Mansoori, Smith, Wu and Ballantyne2014). While it is unclear what role IL-17 plays in adipose tissue (Ahmed and Gaffen, Reference Ahmed and Gaffen2010), it should be noted that obesity is associated with an increase in IL-17 production (Winer et al., Reference Winer, Paltser, Chan, Tsui, Engleman, Winer and Dosch2009). Th17 cells exacerbate hepatic steatosis and liver inflammation (Tang et al., Reference Tang, Bian, Zhao, Liu, Liang, Wang, Han, Peng, Chen, Shen, Qiu, Li and Ma2011), and γδ T cells are increased in adipose tissue in obese mice (Mehta et al., Reference Mehta, Nuotio-Antar and Smith2015); both of these cell types are major sources of IL-17. Moreover, when stimulated peripheral blood mononuclear cells are treated with pro-inflammatory palmitate, there is a dramatic increase in IL-17 production (McCambridge et al., Reference McCambridge, Agrawal, Keady, Kern, Hasturk, Nikolajczyk and Bharath2019). On the anti-inflammatory side, the abundance of Th2 cells in adipose tissue was positively associated with insulin sensitivity in humans (McLaughlin et al., Reference McLaughlin, Liu, Lamendola, Shen, Morton, Rivas, Winer, Tolentino, Choi, Zhang, Hui Yen Chng and Engleman2014). Moreover, Th2-associated cytokines are needed for infiltration of eosinophils in adipose tissue, which in turn promotes macrophage phenotype switching from M1 to M2 (Molofsky et al., Reference Molofsky, Nussbaum, Liang, Van Dyken, Cheng, Mohapatra, Chawla and Locksley2013). Piecing it altogether, a tilting of the scale toward Th1 and Th17 and away from Th2 and Treg responses could be another underlying mechanism behind the low-grade chronic inflammatory state found in metabolic inflammation (Figure 1b).
Effector memory T cells, which are antigen-specific T cells that secrete cytokines, have been identified in adipose tissue of dairy cattle (Oliveira et al., Reference Oliveira, Rasteiro, Correia, Pinto, Meireles, Ferreira, Vilanova and Teixeira2019); however, cytokines produced by these cells in metabolically stressed or healthy cattle have yet to be elucidated. Extrapolation from studies conducted in mice should be done with caution, as species differences between mouse, non-human primate and humans have already been identified in the frequency of Treg cells in adipose tissue (Laparra et al., Reference Laparra, Tricot, Le Van, Damouche, Gorwood, Vaslin, Favier, Benoist, Ho Tsong Fang, Bosquet, Le Grand, Chapon, Lambotte and Bourgeois2019). Supporting this concern, postpartum dairy cattle are characterized as having an anti-inflammatory T cell phenotype compared to late-lactation cows (Shafer-Weaver and Sordillo, Reference Shafer-Weaver and Sordillo1997; Shafer-Weaver et al., Reference Shafer-Weaver, Corl and Sordillo1999), and the production of IFN-γ from stimulated peripheral blood mononuclear cells isolated from peripartum dairy cattle was negatively correlated with non-esterified fatty acid (NEFA) concentrations (Lacetera et al., Reference Lacetera, Scalia, Bernabucci, Ronchi, Pirazzi and Nardone2005). Taken together, there seems to be a conflict between research conducted in obesity-induced mouse models, suggestive of a pro-inflammatory T cell polarization in adipose, and peripartum dairy cattle, suggestive of an anti-inflammatory T cell polarization from blood samples. However, similar to observations in mouse models (Feuerer et al., Reference Feuerer, Herrero, Cipolletta, Naaz, Wong, Nayer, Lee, Goldfine, Benoist, Shoelson and Mathis2009), Treg cells were abundant in mesenteric adipose tissue from healthy, lean dairy cattle (Aylward et al., Reference Aylward, Clark, Galileo, Baernard, Wilson, Brannick, Gressley, Fecteau, Davis and Dyer2019), although a comparison between over-fed, feed-restricted or diseased cattle was not conducted. Potentially more informative, Kosteli et al. (Reference Kosteli, Sugaru, Haemmerle, Martin, Lei, Zechner and Ferrante2010) identified a recruitment of M2 macrophages from circulation into adipose tissue in fasted obese mice, and that these cells played an integral role on tempering lipolysis. This model may better reflect what occurs in transition dairy cattle, especially considering that over-conditioned dairy cows experience more extreme negative energy balance during early lactation and are at a greater risk of disease. Could it be that the anti-inflammatory T cell bias found in postpartum cows is a consequence of anti-inflammatory cytokines generated by a larger population of M2 macrophages? Moreover, could these anti-inflammatory T cells exist to decelerate lipolysis? Speculation aside, much research is needed to illuminate T cell phenotypes and their role in adipose tissue in peripartum cows. γδ T cells, which possess both innate and adaptive immune cell properties, are of great interest due to their abundance in adipose tissue in dairy cattle (∼40% of all T cells; Oliveira et al., Reference Oliveira, Rasteiro, Correia, Pinto, Meireles, Ferreira, Vilanova and Teixeira2019) as well as their apparent role in the recognition of lipid antigens (Champagne, Reference Champagne2011).
Metabolites and lipid mediators influence inflammatory tone
Negative energy balance in early-lactation dairy cows leads to the mobilization of fat reserves, increasing serum NEFA. These fatty acids can be metabolized in the liver for the purpose of energy production, ketone production (e.g. β-hydroxybutyrate, BHB); or in cases of excessive fat mobilization, fat can accumulate in the liver. Moreover, metabolic demands in early lactation augment the production of reactive oxygen species (ROS), leading to oxidative stress. Metabolites, including saturated fatty acids, as well as damage-associated molecular patterns, can influence inflammatory responses (Bradford et al., Reference Bradford, Yuan, Farney, Mamedova and Carpenter2015). Immune cells can be activated through a variety of mechanisms, including toll-like receptors (TLR), nuclear receptors such as peroxisome proliferator-activated receptors (PPAR), and intracellular sensors such as inflammasomes (e.g. nucleotide-binding domain, leucine-rich containing family, pyrin domain-containing-3, also known as NLRP3). While some of these receptors, such as TLR, are more typically thought of as a tool to identify pathogens via pathogen-associated molecular patterns, some metabolites can also activate these pathways. For example, saturated fatty acids activate TLR4 signaling in numerous immune cells, including macrophages (Lee et al., Reference Lee, Sohn, Rhee and Hwang2001), as well as non-immune cells such as hepatocytes (Mamedova et al., Reference Mamedova, Yuan, Laudick, Fleming, Mashek and Bradford2013), though perhaps not through direct receptor–ligand interaction (Lancaster et al., Reference Lancaster, Langley, Berglund, Kammoun, Reibe, Estevez, Weir, Mellett, Pernes, Conway, Lee, Timpson, Murphy, Masters, Gerondakis, Bartonicek, Kaczorowski, Dinger, Meikle, Bond and Febbraio2018). The activation of TLR4 leads to increased production of pro-inflammatory cytokines, including TNF-α, which in turn induces insulin resistance in parenchymal tissue to spare glucose for immune cells to use in driving oxidative burst. It is telling that inflammatory M1 macrophages obtain energy through glycolysis, whereas M2 macrophages obtain energy from oxidative phosphorylation (Galván-Peña and O’Neill, Reference Galván-Peña and O’Neill2014). Fatty acids can also activate PPAR, which are nuclear receptors that function as transcription factors to regulate gene expression. For example, the activation of PPARγ via n-3 polyunsaturated fatty acids attenuates inflammatory responses in mouse monocytes (Lee et al., Reference Lee, Plakidas, Lee, Heikkinen, Chanmugam, Bray and Hwang2003), and some similar evidence exists in periparturient dairy cattle (Silvestre et al., Reference Silvestre, Carvalho, Crawford, Santos, Staples, Jenkins and Thatcher2011; Trevisi et al., Reference Trevisi, Grossi, Cappelli, Cogrossi and Bertoni2011).
Lastly, inflammasomes are cytoplasmic sensors, such as NLRP3, that are triggered by microbial molecules in addition to damage signals related to metabolic stress, including saturated fatty acids and ROS and inhibited by ketone bodies such as BHB. NLRP3 controls the expression of caspase-1, which is used for the maturation of pro-inflammatory cytokines IL-1β and IL-18. More specifically, caspase-1 cleaves pro-IL-1β and pro-IL-18, resulting in the activation of these cytokines. While little is known about inflammasomes in dairy cattle, a relationship has been established between excessive activation of NLRP3 and human diseases such as cardiovascular disease, type 2 diabetes and obesity (Haneklaus and O’Neill, Reference Haneklaus and O’Neill2015). Saturated fatty acids, such as palmitate, activate NLRP3 and induce insulin resistance, likely through IL-1β (Wen et al., Reference Wen, Gris, Lei, Jha, Zhang, Huang, Brickey and Ting2011). Similarly, knocking out NLRP3 improved insulin signaling, reduced the amount of IFN-γ mRNA and protein and reduced the number of effector memory T cells in adipose tissue (Vandanmagsar et al., Reference Vandanmagsar, Youm, Ravussin, Galgani, Stadler, Mynatt, Ravussin, Stephens and Dixit2011). In contrast to the activation by saturated fatty acids, BHB attenuates inflammatory responses associated with NLRP3 (Youm et al., Reference Youm, Nguyen, Grant, Goldberg, Bodogai, Kim, D’Agostino, Planavsky, Lupfer, Kanneganti, Kang, Horvath, Fahmy, Crawford, Biragyn, Alnemri and Dixit2015; Goldberg et al., Reference Goldberg, Asher, Molony, Shaw, Zeiss, Wang, Morozova-Roche, Herzog, Iwasaki and Dixit2017). Moreover, in dairy cattle, a continuous intravenous infusion of BHB decreased glucose concentrations in the blood of postpartum dairy cattle, but had no effect on insulin concentrations, which could be due to enhanced insulin sensitivity (Zarrin et al., Reference Zarrin, Grossen-Rösti, Bruckmaier and Gross2017). In human disease models, BHB suppressed the synthesis of TNF-α (Yamanashi et al., Reference Yamanashi, Iwata, Kamiya, Tsunetomi, Kajitani, Wada, Litsuka, Yamauchi, Miura, Pu, Shirayama, Watanabe, Duman and Kaneko2017) and IL-1β (Youm et al., Reference Youm, Nguyen, Grant, Goldberg, Bodogai, Kim, D’Agostino, Planavsky, Lupfer, Kanneganti, Kang, Horvath, Fahmy, Crawford, Biragyn, Alnemri and Dixit2015; Goldberg et al., Reference Goldberg, Asher, Molony, Shaw, Zeiss, Wang, Morozova-Roche, Herzog, Iwasaki and Dixit2017). Taken together, these data suggest a potential anti-inflammatory mechanism via BHB suppression of inflammasome activation, subsequently improving insulin sensitivity.
Another class of potent lipid mediators are the oxylipids, which have been reviewed in much greater detail elsewhere (Sordillo, Reference Sordillo2018). Oxylipids (also referred to as eicosanoids) are rapidly synthesized from polyunsaturated fatty acids (PUFA) during inflammatory events. Polyunsaturated fatty acids can be oxygenated either enzymatically (cyclooxygenase, lipoxygenase or cytochrome P450 epoxygenase pathways) or through non-enzymatic pathways. Moreover, oxylipids can exert either pro- or anti-inflammatory responses, and this effect can be dependent upon the substrate. Simply put, n-3 PUFA typically yield more anti-inflammatory or resolving oxylipids, whereas n-6 PUFA yield more pro-inflammatory oxylipids (Contreras et al., Reference Contreras, Mattmiller, Raphael, Gandy and Sordillo2012; Raphael and Sordillo, Reference Raphael and Sordillo2013). To add to the complexity of these pathways, pro-inflammatory oxylipids can be further metabolized into anti-inflammatory oxylipids, highlighting their role in both the onset and resolution of inflammation (Sordillo, Reference Sordillo2018). As previously mentioned, metabolic inflammation is characterized as a chronic, low-grade inflammatory state. In early-lactation dairy cows, an increase in plasma pro-inflammatory oxylipids was associated with elevated concentrations of pro-inflammatory cytokines (Raphael et al., Reference Raphael, Halbert, Contreras and Sordillo2014). Moreover, the ratio of pro- to anti-inflammatory oxylipids (such as 9-HODE to 9-oxoODE and 13-HODE to 13-oxoODE) was increased in early lactation, but this ratio decreased as cows approached peak lactation (Raphael et al., Reference Raphael, Halbert, Contreras and Sordillo2014). The administration of exogenous TNF-α during the first week of lactation prevented an increase in plasma anti-inflammatory oxylipids during that week (Yuan et al., Reference Yuan, Farney, Mamedova, Sordillo and Bradford2013), demonstrating a cross-talk between cytokine and oxylipid signaling in transition cows. It should be noted that oxylipid profiles can vary between tissue, milk or plasma samples (Mavangira et al., Reference Mavangira, Gandy, Zhang, Ryman, Jones and Sordillo2015; Contreras et al., Reference Contreras, Strieder-Barboza, De Souza, Gandy, Mavangira, Lock and Sordillo2017), and thus immune responses in one organ could be affected differently than in another organ. This is particularly true for adipose tissue, as the ratio of pro- to anti-inflammatory oxylipids (i.e. 9-HODE:9-oxoODE and 13-HODE:13-oxoODE) is considerably smaller than in plasma (Contreras et al., Reference Contreras, Strieder-Barboza, De Souza, Gandy, Mavangira, Lock and Sordillo2017). Considering all of these findings, changes in lipid metabolism in early-lactation dairy cows likely play a key role in determining the inflammatory tone, although this effect may vary depending on the organ.
Inflammatory tone is maintained in the face of exogenous agents
The evidence above points to an immune–metabolic cross-talk underlying inflammatory tone in early-lactation dairy cattle. However, if inflammatory tone is truly controlled in a homeostatic manner, then it should be possible to observe counter-regulatory responses to exogenous ‘nudges’ in one direction or another. In fact, we observed signs of such counter-regulatory mechanisms in three studies, described below.
In one study, we used oral administration of a non-steroidal anti-inflammatory drug (NSAID), sodium salicylate, to alter inflammatory signaling in early-lactation dairy cattle (Farney et al., Reference Farney, Mamedova, Coetzee, KuKanich, Sordillo, Stoakes, Minton, Hollis and Bradford2013). Although this treatment generally did not cause measurable changes in circulating inflammatory mediators during the 7-day treatment period, we observed a marked rise in pro-inflammatory oxylipids in blood plasma from salicylate-treated cows a week after the treatment ended. This surprising finding led us to suggest that a homeostatic mechanism maintained inflammatory tone in the face of exogenous salicylate by upregulating pro-inflammatory pathways, potentially explaining the limited effects during the treatment window. When the exogenous agent was removed, this shift in endogenous tone was observed as an inflammatory rebound (Farney et al., Reference Farney, Mamedova, Coetzee, KuKanich, Sordillo, Stoakes, Minton, Hollis and Bradford2013). Similar findings have been reported in humans (Pijak, Reference Pijak2006).
Another observation consistent with the results described above was an increase in immune signaling proteins in adipose tissue of cows treated with salicylate for the first 5 days of lactation (Takiya et al., Reference Takiya, Montgomery, Mamedova, Kra, Nemes-Navon, Levin, Fleming, Bradford and Zachut2019). Altered proteins clearly pointed to enhanced complement activation as well as a likely increase in macrophage populations in the tissue in response to oral salicylate. Although other ‘off-target’ mechanisms cannot be ruled out, this enhancement of adipose tissue inflammatory mechanisms may provide the underpinning for homeostatic maintenance of inflammatory signals in the bloodstream of cows treated with this NSAID.
A third study, this time employing pro-inflammatory cytokine administration, provided further evidence of a regulated inflammatory tone. In this experiment, we utilized miniature osmotic pumps to continuously deliver a small amount of recombinant bovine TNF-α into a subcutaneous adipose tissue depot of lactating dairy cows (Martel et al., Reference Martel, Mamedova, Minton, Jones, Carroll and Bradford2014). Unlike studies where a daily bolus administration of an equal amount of TNF-α altered the metabolism of cows (Bradford et al., 2009; Yuan et al., Reference Yuan, Farney, Mamedova, Sordillo and Bradford2013), this continuous administration had no measurable impact on metabolism. Furthermore, transcriptional responses in both liver and contralateral adipose tissue pointed to decreased inflammatory signaling, a surprising finding given the pro-inflammatory nature of the cytokine infused. The most convincing evidence of a counter-regulatory anti-inflammatory response to TNF-α administration was a highly significant increase in IL-10 protein in the contralateral adipose depot (Martel et al., Reference Martel, Mamedova, Minton, Jones, Carroll and Bradford2014), potentially due to a cross-talk between the immune system and the sympathetic nervous system (Bowers et al., Reference Bowers, Festuccia, Song, Shi, Migliorini and Bartness2004) to inhibit TNF-α production (Elenkov et al., Reference Elenkov, Haskó, Kovács and Vizi1995). Here again, a subtle and chronic exogenous ‘nudge’ was counteracted by an apparent homeostatic response in the opposite direction.
Altered set-points for an inflammatory tone: adaptive or pathological?
A hallmark of homeostasis is the use of negative feedback mechanisms to keep a regulated variable near its set-point. Inflammatory responses do not seem to align with this paradigm, because the cytokine storm (along with changes in other mediators) that accompanies an acute inflammatory event causes numerous deviations from typical set-points. The inflammatory response, though, is normally followed by a pre-programming resolution wave that does, in time, bring systems back into homeostasis (Serhan and Savill, Reference Serhan and Savill2005; Sugimoto et al., Reference Sugimoto, Vago, Perretti and Teixeira2019). From the vantage point of days, rather than minutes, perhaps the inflammatory network does not differ from endocrine regulatory systems so much.
In many homeostatic control systems, regulatory targets can be altered, either through pathology or homeorhesis. Considering the inflammatory tone as a regulated system, therefore, is not inconsistent with either acute inflammatory and anti-inflammatory cytokine waves in response to disease or with chronic elevation of inflammatory mediators in obesity. Temporary or chronic changes in inflammatory set-points allow for the activation of various components of the immune system and for alterations in metabolism; whether these changes are considered homeorhetic or pathological ultimately comes down to whether we view them as adaptive or not.
A well-described example of homeorhetic regulation of inflammatory tone is endotoxin tolerance. In this phenomenon, the primary exposure to lipopolysaccharide (LPS) induces an acute inflammatory response, whereas chronic or repeated exposures lead to diminished responses in many animals (Elsasser et al., Reference Elsasser, Blum and Kahl2005; Petzl et al., Reference Petzl, Günther, Pfister, Sauter-Louis, Goetze, von Aulock, Hafner-Marx, Schuberth, Seyfert and Zerbe2012). The concept that a low dose of a potential toxin can protect against subsequent exposures that should be harmful is called hormesis (Mattson, Reference Mattson2008). Although endotoxin tolerance is still being studied, several contributing mechanisms have been described. Endotoxin exposure increases the shedding of TNF-α receptors on monocytes and increases the concentration of soluble ‘decoy’ TNF-α receptors in circulation (van der Poll et al., Reference van der Poll, Calvano, Kumar, Braxton, Coyle, Barbosa, Moldawer and Lowry1995). Moreover, LPS signaling via the receptor CD14 results in TLR4 endocytosis, diminishing its ability to generate intracellular responses to LPS (Zanoni et al., Reference Zanoni, Ostuni, Marek Lorri, Barresi, Barbalat, Barton Gregory, Granucci and Kagan Jonathan2011). Similarly, the release of the acute-phase protein, lipopolysaccharide-binding protein (LBP), facilitates an inflammatory response to endotoxin at low concentrations, but at high concentrations, LBP neutralizes endotoxin in lipid membranes, resulting in the reduction of TNF-α synthesis (Gutsmann et al., Reference Gutsmann, Müller, Carroll, MacKenzie, Wiese and Seydel2001). Each of these mechanisms enables a more tolerant response to LPS, noted by a reduction of pro-inflammatory cytokine synthesis in addition to mechanisms negating the effects of a potential cytokine storm, thus allowing for a more stable inflammatory tone in the face of chronic mild endotoxin exposure.
While the ability of the immune system to develop tolerance toward microbial stimuli has been well established, mechanisms underlying homeostasis in metabolic inflammation have not been clearly described. However, it is noteworthy that the anti-inflammatory effect of adiponectin (an adipocyte-derived cytokine) on macrophages is through the induction of immunological tolerance to pro-inflammatory stimuli (Tsatsanis et al., Reference Tsatsanis, Zacharioudaki, Androulidaki, Dermitzaki, Charalampopoulos, Minas, Gravanis and Margioris2005), in addition to stimulating the production of anti-inflammatory cytokines (Wolf et al., Reference Wolf, Wolf, Rumpold, Enrich and Tilg2004; Wulster-Radcliffe et al., Reference Wulster-Radcliffe, Ajuwon, Wang, Christian and Spurlock2004). It seems probable that metabolic inflammation is enhanced in periparturient dairy cattle due, in part, to reductions in adiponectin (Kabara et al., Reference Kabara, Sordillo, Holcombe and Contreras2014).
Alterations in metabolic flux by inflammatory signaling in adipose tissue
Inflammatory mediators work on a variety of target organs to influence nutrient influx and partitioning. Although most postpartum dairy cows are in a catabolic state requiring the release of stored nutrient reserves, the magnitude of this catabolism varies greatly among cows. There is evidence suggesting that inflammatory mediators, working in part through altered insulin sensitivity, may underlie some of the variation in the rate of tissue catabolism in early lactation (Zachut et al., Reference Zachut, Honig, Striem, Zick, Boura-Halfon and Moallem2013; Zachut, Reference Zachut2015). This is consistent with the emerging roles of immune cells and signals in regulating adipose tissue metabolism.
Lipolysis is characterized by an orchestrated infiltration of a variety of immune cells, including monocytes, granulocytes and lymphocytes (Odegaard and Chawla, Reference Odegaard and Chawla2013). Pro-inflammatory activation of these cells results in the release of cytokines such as TNF-α. In turn, TNF-α promotes lipolysis through a variety of mechanisms; the injection of recombinant TNF-α in cattle reduced feed intake (Kushibiki et al., Reference Kushibiki, Hodate, Shingu, Obara, Touno, Shinoda and Yokomizo2003) and insulin sensitivity (Kushibiki et al., Reference Kushibiki, Hodate, Shingu, Ueda, Shinoda, Mori, Itoh and Yokomizo2001), and directly stimulated lipolysis (Kushibiki et al., Reference Kushibiki, Hodate, Shingu, Hayashi, Touno, Shinoda and Yokomizo2002). The pro-lipolytic effects of inflammatory mediators such as TNF-α are consistent with the typical catabolic response to disease.
In contrast, an elegant series of experiments illuminated an opposing immune mechanism in mice (Kosteli et al., Reference Kosteli, Sugaru, Haemmerle, Martin, Lei, Zechner and Ferrante2010). Caloric restriction in obese mice resulted in a greater number of M2 macrophages in adipose at the onset of negative energy balance (Kosteli et al., Reference Kosteli, Sugaru, Haemmerle, Martin, Lei, Zechner and Ferrante2010), which was also found in both diseased early-lactation dairy cattle and feed-restricted cows compared to cows in an anabolic state (Contreras et al., Reference Contreras, Kabara, Brester, Neuder and Kiupel2015; Reference Contreras, Thelen, Schmidt, Strieder-Barboza, Preseault, Raphael, Kiupel, Caron and Lock2016). Anti-inflammatory macrophages attenuated lipolysis in obese mice during fasting, despite the fact that TNF-α was greater in obese mice compared to lean mice fed a normal chow diet (Kosteli et al., Reference Kosteli, Sugaru, Haemmerle, Martin, Lei, Zechner and Ferrante2010). These data suggest that immunometabolic control of lipolysis is driven by the balance of M1 and M2 macrophages. The simultaneous activity of both M1 and M2 macrophages is consistent with the model of inflammatory homeostasis discussed previously.
One key shortcoming in much of the research conducted with inflammatory mediators has been the short-term nature of studies. We know a tremendous amount about cytokine impacts on metabolic flux via transcriptional or post-translational impacts on nutrient transporters and enzymes, but impacts on tissue remodeling have been largely ignored. This is not a trivial oversight; aside from driving immune responses, inflammatory signaling is probably used most widely in controlling proliferation and differentiation of cells (Cao et al., Reference Cao, Bonizzi, Seagroves, Greten, Johnson, Schmidt and Karin2001; Langen et al., Reference Langen, Schols, Kelders, Wouters and Janssen-Heininger2001; Baldassarre et al., Reference Baldassarre, Nicoloso, Schiappacassi, Chimienti and Belletti2004). For an example of how this can lead to misinterpretation, consider a series of studies with mice engineered to limit inflammatory signaling in adipose tissue (Wernstedt Asterholm et al., Reference Wernstedt Asterholm, Tao, Morley, Wang, Delgado-Lopez, Wang and Scherer2014). Inflammatory mediators generally have lipolytic effects, and these studies of two mouse models were well designed to determine whether eliminating cytokine signaling would enhance adipose triglyceride accretion. In fact, the opposite occurred. Suppressing cytokine signaling inhibited adipogenesis, constraining adipose tissue accretion in growing mice and ultimately resulting in more severe fatty liver disease (Wernstedt Asterholm et al., Reference Wernstedt Asterholm, Tao, Morley, Wang, Delgado-Lopez, Wang and Scherer2014). In this case, removing cytokine stimulation of adipocyte differentiation had a greater impact on tissue mass than did the removal of the lipolytic stimulus. It is important to keep in mind the potential effects of inflammatory mediators on cellular dynamics in addition to metabolic enzymes and transporters.
Implications of non-steroidal anti-inflammatory therapy on dairy cow metabolism and productivity
A growing number of studies have evaluated the use of NSAID to modulate the transition to lactation in dairy cattle, and several have demonstrated interesting impacts on metabolism.
Treatment of dairy cows with oral sodium salicylate for the first 7 days of lactation caused a substantial decrease in blood glucose concentration, particularly among older cows (Farney et al., Reference Farney, Mamedova, Coetzee, KuKanich, Sordillo, Stoakes, Minton, Hollis and Bradford2013). This occurred in the absence of any measurable differences in energy balance compared to control cows during treatment, suggesting that a fuel-specific mechanism was responsible for this effect. We subsequently conducted a follow-up study where multiparous cows were treated with oral sodium salicylate, and plasma glucose turnover was determined with a stable isotope dilution method on day 7 of lactation. We found that glucose turner rate was decreased by 25%, despite a significant decrease in insulin–glucagon ratio (Montgomery et al., Reference Montgomery, Mamedova, Zachut, Kra, Häussler, Vaughn, Gonzalez and Bradford2019). Given the steady-state assumptions used in metabolic flux studies, the decline in glucose turnover reflected both a decreased use of glucose and a decreased supply. Therefore, somewhat surprisingly, the evidence pointed to an inhibition of gluconeogenesis as the primary factor underlying salicylate-induced hypoglycemia in transition dairy cows, rather than enhanced peripheral utilization of glucose. It remains unclear exactly how sodium salicylate inhibited gluconeogenesis, but one plausible mechanism may be that it prevented inflammatory induction of gluconeogenesis (Mamedova et al., Reference Mamedova, Yuan, Laudick, Fleming, Mashek and Bradford2013).
Similar peripartum NSAID treatment strategies have also resulted in substantial increases in milk secretion (Trevisi and Bertoni, Reference Trevisi, Bertoni and Quinn2008; Carpenter et al., Reference Carpenter, Ylioja, Vargas, Mamedova, Mendonca, Coetzee, Hollis, Gehring and Bradford2016; Swartz et al., Reference Swartz, Schramm, Bewley, Wood, Leslie and Petersson-Wolfe2018). Treatment protocols that provided meaningful NSAID to the system for only a few days had rather astounding effects, with milk yield increases of 10% to 15% lasting 10 months. As in the discussion of potential impacts on adipose tissue metabolic flux, the net increase in milk yield could plausibly be due to an increase in either secretory epithelial cell abundance in the mammary gland or to a greater metabolic flux in each secretory cell. Further complicating the matter is the 6-week lag prior to milk responses (Carpenter et al., Reference Carpenter, Ylioja, Vargas, Mamedova, Mendonca, Coetzee, Hollis, Gehring and Bradford2016). Extra-mammary mechanisms may be the primary impact of treatment; for example, alterations in feeding behavior could possibly result in greater nutrient supply, allowing the mammary gland to better meet its potential to synthesize milk (Carpenter et al., Reference Carpenter, Ylioja, Mamedova, Olagaray and Bradford2018). On the other hand, more direct effects are also possible. We and others have observed a reduction in early-lactation somatic cells in milk of cows treated with NSAID (Carpenter et al., Reference Carpenter, Ylioja, Vargas, Mamedova, Mendonca, Coetzee, Hollis, Gehring and Bradford2016; Shock et al., Reference Shock, Renaud, Roche, Poliquin and Olson2018), and inflammatory signals from neutrophils can directly inhibit milk component synthesis both immediately (Connelly et al., Reference Connelly, Barham, Pigg, Saint-Jean, Sherrill, Cheng, Chodosh, Blackwell and Yull2010) and over the longer term, through epigenetic mechanisms (Vanselow et al., Reference Vanselow, Yang, Herrmann, Zerbe, Schuberth, Petzl, Tomek and Seyfert2006). Although the mode of action remains to be clarified, the dramatic and sustained increase in milk secretion following early-lactation NSAID treatment provides a potent example of just how powerful the immunometabolic complex is at the onset of lactation.
Burning questions
While numerous advances have clarified our view on homeostatic mechanisms related to metabolic inflammation, many questions are left unanswered, particularly in reference to periparturient dairy cattle. Elucidating these control mechanisms may better inform researchers searching for potential solutions to improve dairy cattle productivity and immunity. A few of these outstanding questions are outlined below.
What is the biological purpose for maintaining inflammatory tone during NSAID therapy in periparturient dairy cattle?
How does immune cell cross-talk between monocytes, lymphocytes and granulocytes impact inflammatory tone in bovine adipose tissue, and what are the subsequent effects on metabolic adaptations to lactation?
Do monocytes develop immunological tolerance toward metabolite signals, and is this a reason for the low-grade inflammatory tone?
Are there beneficial or deleterious effects from the rebound inflammatory response that occurs following NSAID administration? Does this effect contribute to long-term increases in milk yield, potentially through enhanced mammary gland development?
Conclusions
Resident immune cells are found in all metabolically important tissues, and they have a significant impact on nutrient flux in adipose tissue and perhaps other organs. These impacts are not unidirectional; in fact, adipocytes can alter immune cell phenotypes through both cytokine and nutrient release, whereas resident immune cells serve to clear out residual lipids and also modulate lipolysis. The cross-talk between cells in adipose tissue may have an outsized influence on whole-body inflammatory tone, and may provide for homeostatic regulation of the balance between immune protection and tolerance. Altering the inflammatory tone, even in subtle ways, can have surprisingly large impacts on whole-body nutrient use, including rates of lipolysis, gluconeogenesis and galactopoiesis. Peripartum NSAID administration has drawn attention not only for potential health benefits but also because of these metabolic responses. Although much remains to be understood about site and mode of action of these NSAID, recent findings nonetheless demonstrate the powerful impact of inflammatory signals in the transition period.
Acknowledgements
Contribution no. 19-277-J from the Kansas Agricultural Experiment Station. A brief preliminary version of this work has been published in abstract form (Bradford and Swartz, 2019). The work at Kansas State University described in this review was supported by the USDA National Institute of Food and Agriculture, Hatch project 1018048, and Agriculture and Food Research Initiative Competitive Grant no. 2013-01976, as well as by the National Science Foundation (grant #1456794).
B. J. Bradford 0000-0002-6775-4961
Declaration of interest
Turner Swartz declares no conflict of interest. Barry Bradford has received speaking and/or consulting fees from Bayer AG, Merck Animal Health, Zoetis and Elanco.
Ethics statement
No primary research requiring ethics approvals are reported in this review paper.
Software and data repository resources
None of the findings were deposited in an official repository.