Implications
Environmental pollutants include a wide range of chemicals, mostly man-made, which are present in products that are used on a daily basis, such as detergents, pesticides and adhesives or derived from the combustion of hydrocarbon fuels. Although concentrations of these chemicals in the environment are generally low and, as such, may not induce adverse effects when acting in isolation, combinations of these chemicals can interfere, subtly, with physiological systems and therefore the capacity of ruminants and other animals to reproduce, rear offspring or fight disease. Thus, both the viability of populations and the health and welfare of individuals could be compromised by exposure to environmental levels of pollutants.
Introduction
Historically, ruminant animals production systems were of relatively low intensity; the inputs of energy, food and fertiliser were small and outputs of meat, milk and by-products were low. Accordingly, fertiliser inputs comprised, primarily, animal and human manure with more unusual products such as seaweed being used only where available. Significant accumulation of pollutants in soils, and potential exposure of animals to elevated concentrations of pollutants was rare and occurred only in certain highly specialised circumstances, for example, where soils were repeatedly fertilised, with ash and bird carcases (Meharg et al., Reference Meharg, Deacon, Edwards, Donaldson, Davidson, Spring, Scrimgeour, Feldmann and Rabb2006). On the other hand, the potential for pollutant exposure in modern production systems is greatly increased, for several reasons.
First, modern animal production systems involve the widespread use of pesticides and herbicides and traditional organic fertiliser has been replaced to a significant extent with synthetic nitrate fertilisers. However, the economical and environmental costs of inorganic fertiliser production, together with anthropogenic waste generation, have led, in many countries, to a return to the use of processed sewage sludge instead (Commission of the European Communities, 1994; Swanson et al., Reference Swanson, Bortman, O’Connor and Stanford2004). The chemical profile of sludge (Smith, Reference Smith1995) reflects the mix of thousands of different environmental chemicals to which we are exposed, and its use gives rise to potentially increased exposure to, and bioaccumulation of, chemicals. Other potentially polluted materials such as composted green waste, derived from domestic and municipal sources are also being applied to land. Thus, domestic ruminants may be exposed to concentrations of pollutants that are higher than those occurring ‘naturally’ in the environment, either through the application of chemicals or because they are exposed to modern waste through recycling of waste to land. However, at this time, it is logistically impossible to characterise the magnitude of potential exposure owing to the complexity of the mixture and the high cost of analysis.
Second, while aerial input of pollutants to the environment as a result of human actions would originally have been relatively trivial, comprising small amounts of potentially toxic metals (PTMs) derived from the smelting of ores (Hong et al., Reference Hong, Candelone, Patterson and Boutron1996) and polycyclic aromatic hydrocarbons (PAHs) as a result of natural and man-made fires (Bostrom et al., Reference Bostrom, Gerde, Hanberg, Jernstrom, Johansson, Kyrklund, Rannug, Törnqvist, Victorin and Westerholm2002; Yunker et al., Reference Yunker, Macdonald, Vingarzan, Mitchell, Goyette and Sylvestre2002), in recent decades aerial inputs have increased enormously. Thousands of new, partially volatile, organic chemicals manufactured for a multitude of industrial, agricultural and domestic purposes are being released into the environment. Occasionally, air pollution has been sufficiently severe to cause damage to plants (e.g. acid rain) but effects on animals, and ruminants in particular, have seldom been proven, although often suspected (Kelly, Reference Kelly1995).
Environmental pollutants – what are they and from where do they originate?
Most, but not all, pollutant types are derived from human activities. From around the time of the Second World War, the nature of the pollutant burden within the environment, its distribution and effects have changed significantly, largely as a result of human activities and inventions. As indicated above, while PTMs have been used for thousands of years, their use has increased enormously and so they have become ubiquitous in the environment and now appear at low concentrations in soils and at higher concentrations in products such as sewage sludge (Smith, Reference Smith1995). The potential for low-environmental concentrations of metals to perturb animal physiology is now being recognised (Spurgeon et al., Reference Spurgeon, Hopkin and Jones1994).
Nitrates are another category of inorganic pollutant that can interfere with animal physiology and in particular with reproductive function; specifically, they have been implicated in altered thyroid function and the disruption of gonadal steroidogenesis (Guillette and Edwards, Reference Guillette and Edwards2005; Edwards et al., Reference Edwards, Miller and Guillette2006). Thus, their presence in drinking water is of concern to animal (and human) health and productivity.
Human activity and industrialisation have resulted in an increase in the manufacture, use and release into the environment, of a wide variety of organic substances (Table 1). Although not designed to be physiologically active, many of these can bind to steroidal and other cellular receptors or otherwise interfere with endocrine signalling or enzyme systems and thus affect physiological processes in species as diverse as bacteria (Fox, Reference Fox2004) and mammals (Toppari et al., Reference Toppari, Larsen, Christiansen, Giwercman, Grandjean, Guillette, Jegou, Jensen, Jouannet, Keiding, Leffers, McLachlan, Meyer, Muller, Meyts, Scheike, Sharpe, Sumpter and Skakkebaek1996; Sweeney et al., Reference Sweeney, Nicol, Roche and Brooks2000). Environmental concentrations of these pollutants are seldom high enough to exert toxic effects, in the conventional sense, being similar to background levels (Rhind, Reference Rhind2009), but through subtle effects on physiological systems they can interfere with normal function.
Table 1 Some classes of organic pollutant and their sources or route of entry to the environment

PAH = polycyclic aromatic hydrocarbons; PCB = polychlorinated biphenyls; PBDE = polybrominated diphenyl ethers.
Chemicals that induce effects by perturbing endocrine systems or mimicking endocrine mediators are collectively described as endocrine disrupting compounds (EDCs). Although pollutants can be very different, chemically and mechanistically, for the purpose of this review, it is appropriate to consider all of these organic and inorganic pollutant classes together and to loosely define them as EDCs because all are known to have disruptive capabilities and they have the potential to interact, additively (Bemis and Seegal, Reference Bemis and Seegal1999).
How do these pollutants affect ruminants?
Investigations of the effects of EDC exposure on animals have frequently involved the application of pharmacological doses of individual pollutants to laboratory rodents (to elucidate effects and mechanisms of action and to identify risks associated with exposure to individual chemicals) and less controlled studies of wild animals in which effects had been observed following known pollution incidents (Colborn et al., Reference Colborn, Vom Saal and Soto1993; Institute for Environment and Health (IEH), 1999). Such studies have identified several characteristics of EDCs that make them biologically significant in relation to all animals, including domestic ruminants. They (i) are highly persistent – for example, many EDCs, including PAHs, polychlorinated biphenyls (PCBs) and polybrominated diphenyl ethers (PBDEs), have half lives of about 10 years or more (Smith, Reference Smith1995) or in the case of heavy metals are never degraded, (ii) are ubiquitous, that is, although production and use can be localised, EDCs are distributed throughout the environment, (iii) frequently accumulate in animal tissue because they are hydrophobic and lipophilic (Nimrod and Benson, Reference Nimrod and Benson1996), (iv) exert effects on physiological systems at very low concentrations, orders of magnitude lower than those known to have acute toxic effects (Brevini et al., Reference Brevini, Cillo, Antonini and Gandolfi2005; Fowler et al., Reference Fowler, Abramovich, Haites, Cash, Groome, Al-Qahtani, Murray and Lea2007a), (v) have unpredictable effects as they can act additively or synergistically (Payne et al., Reference Payne, Rajapakse, Wilkins and Kortenkamp2000; Rajapakse et al., Reference Rajapakse, Silva and Kortenkamp2002; Crofton et al., Reference Crofton, Craft, Hedge, Gennings, Simmons, Carchman, Carter and deVito2005; Hauser et al., Reference Hauser, Williams, Altshul and Calafat2005), depending on circumstances; mixtures of compounds can induce biological responses, even when each chemical is present at concentrations too low to induce a biological response by itself (Rajapakse et al., Reference Rajapakse, Silva and Kortenkamp2002; Kortenkamp, Reference Kortenkamp2007) and (vi) can induce changes in organ structure or function in subsequent, unexposed, generations (Bøgh et al., Reference Bøgh, Christensen, Dantzer, Groot, Thøfner, Rasmussen, Schmidt and Greve2001; Anway and Skinner, Reference Anway and Skinner2006; Edwards and Myers, Reference Edwards and Myers2007; Steinberg et al., Reference Steinberg, Walker, Juenger, Woller and Gore2008).
Collectively, these properties mean that low-level exposure to pollutants has the potential to affect ruminant productivity and, for example, through changes in the immune system, health and welfare. However, the magnitude of the responses, which is likely to depend on many factors, including the rate, timing and duration of exposure, is ill defined and poorly understood for all animal species.
Patterns of exposure and tissue concentrations
Ruminants, like other terrestrial species, can be exposed to pollutants, at least theoretically, through ingestion of food and water, through inhalation and by absorption through the skin. It is widely accepted that the primary route of exposure in such species is via the diet (Fries, Reference Fries1995; Norstrom, Reference Norstrom2002) but the significance of other routes of exposure has not been extensively investigated and they may yet prove to be important. Exposure of target organs also depends on the chemical class and associated properties, the age and stage of development of the exposed animal (i.e. foetal, neonatal and adult), the rate of pollutant uptake and rates of subsequent degradation, excretion and/or metabolism (Meador et al., Reference Meador, McCaarty, Escher and Adams2008; Rhind, Reference Rhind2008). None of these determinants has been well characterised in ruminants, or indeed for any species, or for any of the classes of EDCs. However, as exposure rates are normally very low and as the health and productivity of the majority of ruminant populations, like that of humans, appears to be unaltered by such levels of exposure, at least superficially, it might be concluded that the environment is almost always entirely benign.
In certain production systems, ruminants can be exposed to slightly higher levels of thousands of different pollutants, relative to those seen in the wider environment, for example when animals are grazed on pastures fertilised with sewage sludge (Rhind, Reference Rhind2005) or drink water contaminated with sewage (Meijer et al., Reference Meijer, de Bree, Wagenaar and Spoelstra1999). The practice of recycling human waste is ancient and ‘night soil’ was collected and returned to land both before and after industrialisation. Processed sewage sludge, as generated in the 21st century, however, is a very different product to that, which has been used as fertiliser in the past as it contains variable combinations of anthropogenic pollutants including organic pollutants and PTMs from domestic, agricultural and industrial sources, at much higher concentrations than those found in the rest of the natural environment (Smith, Reference Smith1995). However, the actual pattern of exposure to individual pollutants is unknown because it would require analysis of thousands of different chemicals. Rates of tissue accumulation of selected chemicals, and associated effects on the physiology of grazing animals on pastures fertilised with sewage sludge have been addressed both theoretically and in practice. Theoretical estimation of tissue accumulation of individual pollutants would suggest that increases in tissue levels would be small and of no physiological consequence (Wild and Jones, Reference Wild and Jones1992; Duarte-Davidson and Jones, Reference Duarte-Davidson and Jones1996). These conclusions have been largely supported by empirical studies that involved exposure of animals to sewage sludge or to specific compounds (Fries and Marrow, Reference Fries and Marrow1977; Fries et al., Reference Fries, Cook and Prewitt1978; Fries, Reference Fries1996; Rhind et al., Reference Rhind, Kyle, Telfer, Duff and Smith2005a, Reference Rhind, Kyle and Owen2005b, Reference Rhind, Kyle, Mackie and Telfer2007 and Reference Rhind, Kyle, Mackie and Telfer2009). None of these studies reported the patterns of reproductive performance associated with exposure to pollutants but related studies, discussed below, have shown that even such small increases in tissue concentrations of the pollutants measured, following sludge exposure, are associated with physiological changes. It should be noted that a relatively limited range of chemical types has been measured in tissue and it cannot be assumed that those measured are the ones responsible for inducing the observed effects; at best, the reported values represent an index of the total pollutant ‘insult’, which is likely to include several thousand chemicals.
Effects on animal physiology
The fundamental mechanisms of action of pollutants have been investigated and reviewed previously (Sikka and Naz, Reference Sikka and Naz1999; Rhind, Reference Rhind2002). The majority of experiments have been based on laboratory rodents and studies in ruminants are relatively rare; a small number of studies, mostly in sheep, have addressed the physiological effects of EDC exposure, using the classical model whereby relatively high concentrations of selected pollutants are administered for short periods of time (Beard et al., Reference Beard, Bartelewski, Chandolia, Honaramooz and Rawlings1999; Sweeney et al., Reference Sweeney, Nicol, Roche and Brooks2000; Wright et al., Reference Wright, Evans, Evans, Duffy, Fox, Boland, Roche and Sweeney2002). However, additional results are now emerging from investigation of the effects of prolonged, low-level exposure to EDC mixtures in sheep that have been maintained on sewage sludge-treated pastures (Erhard and Rhind, Reference Erhard and Rhind2004; Paul et al., Reference Paul, Rhind, Kyle, Scott, McKinnell and Sharpe2005; Fowler et al., Reference Fowler, Dorà, McFerran, Amezaga, Miller, Lea, Cash, McNeilly, Evans, Cotinot, Sharpe and Rhind2008; Bellingham et al., Reference Bellingham, Fowler, Amezaga, Rhind, Cotinot, Mandon-Pepin, Sharpe, Kyle and Evans2009; Lind et al., Reference Lind, Gustavsson, Hermsen, Larsson, Kyle, Örberg and Rhind2009). In these studies, the concentrations of chemicals to which animals were exposed, and the specific mixture of chemicals involved, were not comprehensively defined because this would have been logistically impossible. However, effects of exposure were demonstrated by comparing animals reared on sludge-treated pastures with others reared on comparable pastures treated with inorganic fertiliser, which contains minimal amounts of pollutants.
The majority of reported ruminant trials have concentrated on the effects of EDC exposure on the reproductive axis and the results can be classed according to organ/function.
Hypothalamic-pituitary axis
The endogenous activity of the gonads is driven by the actions of the hypothalamus and pituitary gland, both of which are steroid-sensitive and, thus, potential targets for EDCs. Studies in sheep and goats have reported significant effects of exposure to chemicals with known endocrine disrupting effects, including octylphenol (Sweeney et al., Reference Sweeney, Nicol, Roche and Brooks2000; Wright et al., Reference Wright, Evans, Evans, Duffy, Fox, Boland, Roche and Sweeney2002), bisphenol A (Evans et al., Reference Evans, North, Dye and Sweeney2004; Savabieasfahani et al., Reference Savabieasfahani, Kannan, Astapova, Evans and Padmanabhan2006), methoxyclor (Savabieasfahani et al., Reference Savabieasfahani, Kannan, Astapova, Evans and Padmanabhan2006), PCB153 (Lyche et al., Reference Lyche, Oskam, Skaare, Reksen, Sweeney, Dahl, Farstad and Ropstad2004; Oskam et al., Reference Oskam, Lyche, Krogenaes, Thomassen, Skaare, Wiger, Dahl, Sweeney, Stien and Ropstad2005) and valporate (Krogenaes et al., Reference Krogenaes, Tauboll, Stien, Oskam, Lyche, Dahl, Thomassen, Sweeney and Ropstad2008), on the hypothalmic-pituitary (HP) gland axis. The majority of these studies have investigated the effects of EDCs on the HP axis during development, that is, during gestation and/or lactation, the most sensitive ‘windows’, when the endocrine system can be permanently altered (IPCS, 2002). Comparison of the results obtained in these studies show that although the chemicals applied and/or the purported mechanisms of action were similar, the observed effects were often chemical and species specific. For example, the timing of puberty, which reflects activation of the HP axis, was advanced in female lambs exposed to octylphenol (Wright et al., Reference Wright, Evans, Evans, Duffy, Fox, Boland, Roche and Sweeney2002), delayed in both male (Oskam et al., Reference Oskam, Lyche, Krogenaes, Thomassen, Skaare, Wiger, Dahl, Sweeney, Stien and Ropstad2005) and female (Lyche et al., Reference Lyche, Oskam, Skaare, Reksen, Sweeney, Dahl, Farstad and Ropstad2004) goats exposed to PCB153, and remained unchanged in male (Oskam et al., Reference Oskam, Lyche, Krogenaes, Thomassen, Skaare, Wiger, Dahl, Sweeney, Stien and Ropstad2005) and female (Lyche et al., Reference Lyche, Oskam, Skaare, Reksen, Sweeney, Dahl, Farstad and Ropstad2004) goats exposed to PCB126, during development. These results illustrate the complexity of the effects of EDCs on the HP axis and the difficulty of extrapolating between, or even within, species.
In line with the hypothesis that exposure, during critical windows of development can be more disruptive to physiological systems, the results of studies that exposed sheep to the same dose of octylphenol, at different development stages, indicated different physiological consequences. Exposure to pharmacological doses during development (in utero and early post natal period), the period during which HP axis differentiation and sexual dimorphism occurs (Rhind et al., Reference Rhind, Rae and Brooks2001; Robinson, Reference Robinson2006), was found to be potentially more detrimental than later exposure and was seen to induce effects on reproductive function/physiology which were manifested only in later life. For example, gestational exposure to octylphenol at 1 mg/kg per day for 2 weeks resulted in reduced foetal FSH secretion, which compromised testis development (Sweeney et al., Reference Sweeney, Nicol, Roche and Brooks2000) and lactational exposure was associated with altered semen quality (Sweeney et al., Reference Sweeney, Fox, Robertson, Kelly, Duffy, Lonergan, O’Doherty, Roche and Evans2007). Similarly, octylphenol exposure of female lambs, in utero, altered FSH secretion during the late follicular phase, and changed the timing of puberty (Wright et al., Reference Wright, Evans, Evans, Duffy, Fox, Boland, Roche and Sweeney2002). However, exposure to similar doses of octylphenol during the pre-pubertal period had no significant effect on either LH or FSH secretion in female lambs (Evans et al., Reference Evans, North, Dye and Sweeney2004).
Bisphenol A exposure, also at pharmacological doses (5 mg/kg per day for 2 months) has also been shown to suppress LH secretion in female sheep either when exposure occurs during development (Savabieasfahani et al., Reference Savabieasfahani, Kannan, Astapova, Evans and Padmanabhan2006) or during the pre-pubertal period (Evans et al., Reference Evans, North, Dye and Sweeney2004). Although it was not possible to determine the exact nature and location of action in these studies, Katoh et al. (Reference Katoh, Matsuda, Ishigami, Yonekura, Ishiwata, Chen and Obara2004) showed that bisphenol exposure affected growth hormone through an effect exerted at the level of the pituitary gonadotrophes, whereas other studies such as those by Wright et al. (Reference Wright, Evans, Evans, Duffy, Fox, Boland, Roche and Sweeney2002) with octylphenol, and Lyche et al. (Reference Lyche, Oskam, Skaare, Reksen, Sweeney, Dahl, Farstad and Ropstad2004) with PCB153, have indicated that EDC exposure can have effects at the level of the hypothalamus as puberty is driven by maturational changes in the hypothalamus and the timing of puberty was affected in both studies.
A criticism of the above studies that have investigated the effects of EDCs on the HP axis is that they addressed effects of exposure to pharmacological doses of single chemicals, at concentrations hundreds or thousands of times higher than the levels present in the environment, where effects are likely to be exerted through the actions of many chemicals, at low concentrations, in combination. Studies using the sewage sludge model described earlier provide a means to address this real world exposure. Preliminary results indicate that sludge exposure alters the population of gonadotrophes in the pituitary glands of adult ewes that had been maintained on these pastures and changes the phenotype of pituitary cell populations. Changes in the activity of a number of neurotransmitter systems within the hypothalamus have been reported (Bellingham et al., Reference Bellingham, Fowler, Amezaga, Rhind, Cotinot, Mandon-Pepin, Sharpe, Kyle and Evans2009). Given the fundamental importance of the HP axis in the regulation of normal gonadal function, alterations to this system by EDCs, may have deleterious consequences for ovarian or testicular function and thus reproductive function and fertility.
Testis
As for the HP axis, most studies of EDC effects have involved laboratory animals and employed levels of chemical exposure that are probably not environmentally relevant (Hotchkiss et al., Reference Hotchkiss, Rider, Blystone, Wilson, Hartig, Ankley, Foster, Gray and Gray2008). More recently, several well-designed studies have investigated the effect of mixtures of EDCs on the developing rodent testis and its functions, and have shown that combinations of, for example, anti-androgenic EDCs, exert major effects at doses at which the individual EDCs have no significant effect (Christiansen et al., Reference Christiansen, Scholze, Axelstad, Boberg, Kortenkamp and Hass2008; Rider et al., Reference Rider, Wilson, Howdeshell, Hotchkiss, Furr, Lambright and Gray2009). Such observations indicate that it is likely that in domestic animals, exposure to the thousands of EDCs in the environment will exert effects on the developing testis, although the large numbers of chemicals involved and the potential complexities of their interactions make it difficult to predict the incidence or severity of such effects. Nevertheless, the high incidence of reproductive abnormalities in human males at birth (cryptorchidism and hypospadias) and in adulthood (e.g. low-sperm counts), together with the evidence of temporal changes in incidence of these disorders, especially of falling/low-sperm counts (Swan et al., Reference Swan, Elkin and Fenster2000), is at least consistent with environmental impacts (Skakkebaek et al., Reference Skakkebaek, Rajpert-De Meyts and Main2001; Sharpe and Skakkebaek, Reference Sharpe and Skakkebaek2003).
The issue of falling sperm counts in human males remains controversial, largely because of difficulties in proving/disproving that it is happening and of identifying potential causes (Swan et al., Reference Swan, Elkin and Fenster2000). One argument against sperm counts having fallen is the absence of evidence of any similar decline in domestic ruminants, over the same time period; it is assumed that their exposures should be broadly similar to that of humans (Setchell, Reference Setchell1997). However, this comparison is invalid for two reasons. First, and most important, domestic ruminants store sperm and are thus able to maintain a high and uniform sperm count over many frequent ejaculations, whereas humans do not store sperm and therefore sperm counts are greatly affected by ejaculatory frequency (Sharpe, Reference Sharpe1994). Consequently, sperm counts in the ejaculates of domestic ruminants do not provide an accurate insight into the level of sperm production, whereas in humans it does (Sharpe, Reference Sharpe1994; Sharpe and Skakkebaek, Reference Sharpe and Skakkebaek2003). Second, domestic animals are constantly selected for high fertility/sperm production and normal husbandry practice would have resulted in the culling of less fertile animals within the timescale that sperm counts have ‘fallen’, whereas reproductive technologies act to perpetuate and potentially exacerbate defects in human sperm production.
The rodent EDC mixture studies referred to above have not so far addressed effects on sperm counts/sperm production, although the reported adverse effects on foetal testis development including suppression of androgen production/action would be expected to reduce Sertoli cell proliferation in foetal life (Scott et al., Reference Scott, Hutchison, Mahood, Hallmark, Welsh, de Gendt, Verhoeven, O’Shaughnessy and Sharpe2007 and Reference Scott, Hutchison, Jobling, McKinnell, Drake and Sharpe2008). Similar results have also been found in the foetal sheep after pregnant ewes were reared on pasture fertilised with sewage sludge (Paul et al., Reference Paul, Rhind, Kyle, Scott, McKinnell and Sharpe2005); specifically, foetal blood testosterone levels were reduced alongside of reductions in Leydig, Sertoli and germ cell numbers. Although these effects were considerable, they did not allow dissection of mechanisms and of cause and effect relationships; on the basis of rodent studies, it would be expected that reduced intra-testicular testosterone concentrations during development would result in reduced Sertoli cell number in the adult (Scott et al., Reference Scott, Hutchison, Mahood, Hallmark, Welsh, de Gendt, Verhoeven, O’Shaughnessy and Sharpe2007). However, studies in the rat have also shown that even when Sertoli cell number is reduced at birth by 40% to 50%, compensation occurs rapidly after birth so that normal Sertoli cell numbers are restored by puberty and maintained into adulthood (Hutchison et al., Reference Hutchison, Scott, Walker, McKinnell, Mahood, Ferrara and Sharpe2008; Scott et al., Reference Scott, Hutchison, Jobling, McKinnell, Drake and Sharpe2008). However, this recovery occurred following cessation of the causal treatment (in this instance, dibutyl phthalate) and so it could be argued that continued EDC exposure through foetal and postnatal life, which is more akin to ‘real world’ exposures, might interfere with compensatory Sertoli cell proliferation; this possibility remains to be tested.
Rat studies have led to the identification of ‘a male programming window’ (Welsh et al., Reference Welsh, Saunders, Fisken, Scott, Hutchison, Smith and Sharpe2008), during which androgens (produced by the foetal testis) act to programme later development of the reproductive tract and genitalia. Deficient androgen action during this time window leads to permanent reductions in size of the penis, prostate and testis and increased risk of malformations such as hypospadias and cryptorchidism (Welsh et al., Reference Welsh, Saunders, Fisken, Scott, Hutchison, Smith and Sharpe2008). Exactly, the same time window applies to programming of the male–female difference in anogenital distance and so the latter, at any age after birth, can provide an index of overall androgen exposure/action within the male programming window (Scott et al., Reference Scott, Hutchison, Jobling, McKinnell, Drake and Sharpe2008; Welsh et al., Reference Welsh, Saunders, Fisken, Scott, Hutchison, Smith and Sharpe2008). A similar time window applies to domestic ruminants, the best characterised being in the sheep (Wood and Foster, Reference Wood and Foster1998), and is thought, by analogy to the rat and human, to start when testosterone production by the foetal testis first commences (Welsh et al., Reference Welsh, Saunders, Fisken, Scott, Hutchison, Smith and Sharpe2008). With regard to the effects of EDC exposure, the most important implication of these findings is that only exposure within the male programming window is likely to affect androgen-dependent reproductive development. However, development of the normally formed penis (Welsh et al., Reference Welsh, Saunders, Fisken, Scott, Hutchison, Smith and Sharpe2008) and the increase in Sertoli cell number/determination of adult testis size (Scott et al., Reference Scott, Hutchison, Jobling, McKinnell, Drake and Sharpe2008) also depend upon androgen action after the male programming window and therefore may be susceptible to EDC effects for a greater period.
Ovary
Ovarian follicle formation in ruminants occurs during foetal life (Rüsse, Reference Rüsse1983) and involves the assembly of meiotically arrested oocytes and somatic pre-granulosa cells into primordial follicles (Hirshfield, Reference Hirshfield1991). EDCs could, potentially, perturb the function of each of the cell types involved. Female germ cells begin meiosis during early development, and EDC-exposure at this time can potentially reduce a female’s lifetime reserve of oocytes, which cannot be renewed, unlike males in which continuous spermatogenesis may quench transient EDC effects. On the other hand oogonia may be less sensitive than male germ cells to some gonotoxic insults (e.g. Guerquin et al., Reference Guerquin, Duquenne, Coffigny, Rouiller-Fabre, Lambrot, Bakalska, Frydman, Habert and Livera2009).
The developmental stage at which damage occurs determines the impact that exposure to chemicals will have on reproduction (Hoyer, Reference Hoyer2005; Uzumcu and Zachow, Reference Uzumcu and Zachow2007). Chemicals selectively damaging large growing or antral follicles only temporarily interrupt reproductive function, unlike when damage to the primordial follicle population occurs, because these are replaced by recruitment from the primordial follicle pool. Two key developmental processes occur subsequent to oocyte meiotic arrest: (i) primordial follicle assembly (at 75-day gestation in sheep: Sawyer et al., Reference Sawyer, Smith, Heath, Juengel, Wakefield and McNatty2002) and (ii) primordial follicle recruitment. Both are coordinated by paracrine and autocrine growth factors and occur in the later stages of ruminant gestation (McNatty et al., Reference McNatty, Heath, Lundy, Fidler, Quirke, O’Connell, Smith, Groome and Tisdall1999). Primordial follicles are then activated and recruited into the growing cohort of primary follicles, a vital determinant of reproductive life span (Skinner, Reference Skinner2005). Thus it is possible to investigate the effects of EDC exposure in relation to the known developmental changes that occur during maturation of the oocycte, its release and fertilisation.
As multiple ovarian systems are sensitive to EDCs, associated effects are diverse and dependent on the specific chemical involved (Table 2). As for other organs, exposure to environmental concentrations of pollutant mixtures has been shown to perturb ovarian development in the sheep (Fowler et al., Reference Fowler, Dorà, McFerran, Amezaga, Miller, Lea, Cash, McNeilly, Evans, Cotinot, Sharpe and Rhind2008; Mandon-Pepin et al., Reference Mandon-Pepin, Loup, Poumerol, Fowler, Amezaga, Sharpe, Evans, Bellingham, Rhind and Cotinot2009) and follicle health in the adult offspring (Figure 1; Amezaga et al., Reference Amezaga, Speers, Bellingham, Evans, Mandon-Pepin, Cotinot, Sharpe, Rhind and Fowler2009). Disruption of the first stages of gametogenesis and gonadal differentiation, in utero, ultimately control reproductive viability in mature offspring and can have transgenerational consequences (Anway and Skinner, Reference Anway and Skinner2006).
Table 2 Ovarian follicle and oocyte disruption by EDC – mechanisms and effects

EDC = endocrine disrupting compound; BPA = bisphenolA; DES = diethylstilbestrol; EE2 = ethinyl estradiol; PCB = polychlorinated biphenyls; MEHP = mono ethylhexyl phthalate.
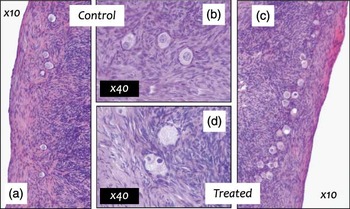
Figure 1 Ovaries (hematoxylin-stained sections) of 18-month-old ewes exposed from conception to control inorganic fertiliser (a, b) or sewage sludge fertiliser (c, d); panels a and c ×10, b and d ×40 objective magnification. Treated ovaries have a higher proportion of unhealthy primordial and activating primordial follicles. Unhealthy follicles include follicles with intense nuclear staining (c, d), vacuoles (c) and clusters of two or more oocytes, which often share a common layer of granulosa cells (c, d).
With regard to future investigations of these effects, it should be noted that much of the literature concerning reproductive effects of EDCs is based on rodent models but ruminants exhibit significant differences in physiology compared with, say, laboratory rodents such as mice; for example, steroidogenesis occurs in ruminants during foetal, not postnatal, ovarian development. Thus, the use of the rodent model alone to understand risks posed to domestic ruminants is unwise.
Oocyte maturation and early embryo development
During maturation, the oocyte and early embryo are particularly susceptible to pollutants, even at the background doses to which they might be exposed, in vivo, when their mothers are not exposed to elevated environmental levels of pollutants (Pocar et al., Reference Pocar, Brevini, Perazzoli, Cillo, Modina and Gandolfi2001a and Reference Pocar, Perazzoli, Luciano and Gandolfi2001b). For example, incubation with PCBs (0.0001 to 1 μg/ml of a mixture of PCBs (Aroclor); exposed for 24 to 48 h) significantly reduced the percentage of bovine oocytes reaching metaphase II, and lower doses that do not impair meiotic processes reduced fertilisation rates and embryonic development (Pocar et al., Reference Pocar, Brevini, Perazzoli, Cillo, Modina and Gandolfi2001a and Reference Pocar, Perazzoli, Luciano and Gandolfi2001b). Some of the underlying mechanisms responsible for this, and similar effects, have been identified (Table 2) but many potential mechanisms remain to be investigated.
The processes of endometrial transformation, and development and implantation of the embryo depend on an exchange of hormonal signals between the embryo and mother. During early embryo development and the implantation window, specific amounts of oestradiol (E2) and progesterone (P) are required for endometrial maintenance and chorionic gonadotropin for maintenance of hormone production by the corpus luteum (Makrigiannakis et al., Reference Makrigiannakis, Minas, Kalantaridou and Chrousos2006). These vital endocrine dialogues can be disturbed by EDCs. In addition to indirect effects of EDCs on embryo development, exerted via changes in hormone profiles, direct embryotoxic effects are possible through actions of EDCs on hormone receptors (Agras et al., Reference Agras, Shiroyanagi and Baskin2007; Davey et al., Reference Davey, Bodwell, Gosse and Hamilton2007). It has been shown that the hormone receptor expression in blastocysts differs between embryoblast and trophoblast, which represent different cell lineages, and this results in different developmental impacts (Navarrete Santos et al., Reference Navarrete Santos, Tonack, Kirstein, Kietz and Fischer2004a, Reference Navarrete Santos, Tonack, Kirstein, Pantaleon, Kaye and Fischer2004b and Reference Navarrete Santos, Ramin, Tonack and Fischer2008). Along with steroid hormone receptors, many of the effects of EDCs on pre-implantation embryos, and on implantation, are mediated through the aryl hydrocarbon receptor (AhR) and peroxisome proliferator-activated receptor (PPAR) signalling pathways. The AhR, a binding partner of dioxins and coplanar PCBs (McMillan and Bradfield, Reference McMillan and Bradfield2007), is essential for fertility, being involved in folliculogenesis, oestrogen biosynthesis and signalling, progesterone biosynthesis and corpus luteum function (e.g. Pocar et al., Reference Pocar, Augustin and Fischer2004, Reference Pocar, Fischer, Klonisch and Hombach-Klonisch2005a and Reference Pocar, Nestler, Risch and Fischer2005b, Li et al., Reference Li, Liu, Dai, Lu, Yang and Huang2006; Barnett et al., Reference Barnett, Tomic, Gupta, Babus, Roby and Terranova2007, Ohtake et al., Reference Ohtake, Fujii-Kuriyama and Shigeaki2009). It may be necessary, also, for normal development of the pre-implantation embryo (Clausen et al., Reference Clausen, Kietz and Fischer2005) and embryo-maternal signalling during implantation. Both indirect and direct EDC effects were observed at environmentally relevant concentrations with exposure durations between 4 h and 7 days (short-term) or 6 to 12 weeks (long-term).
Pre-implantation exposure to EDCs of a variety of classes, at environmentally relevant concentrations, can perturb the reproductive and implantation systems of early embryos through some of the above mechanisms (Table 3). Effects include perturbation of energy metabolism (Tonack et al., Reference Tonack, Kind, Thompson, Wobus, Fischer and Navarrete Santos2007) and downregulation of relevant genes (Hanlon et al., Reference Hanlon, Cimafranca, Liu, Cho and Jefcoate2005). As PPAR signalling is disrupted by EDCs (Huang, Reference Huang2008; Nakanishi, Reference Nakanishi2008) and affects AhR expression (Hanlon et al., Reference Hanlon, Ganem, Cho, Yamamoto and Jefcoate2003; Lovekamp-Swan and Davis, Reference Lovekamp-Swan and Davis2003; Villard et al., Reference Villard, Caverni, Baanannou, Khalil, Martin, Penel, Pineau, Seree and Barra2007), it directly links metabolic and EDC pathways with pollutants.
Table 3 Early embryo disruption by EDC – mechanisms and effects

EDC = endocrine disrupting compound; TCDD = 2,3,7,8-Tetrachlorodibenzo-p-dioxin; PAH = polycyclic aromatic hydrocarbons; PCB = polychlorinated biphenyls.
In summary, embryo implantation is highly vulnerable to endocrine disruption, as EDCs, (plasticiser, PAHs, PCBs, PDBEs, dioxins, pesticides, organotins and heavy metals) at concentrations as low as 10 nM or 2 ng/kg and for an exposure period as short as 4 h, can interfere with the actions of many hormones and receptors essential for pre- and peri-implantation development of the embryo and endometrium.
Effects on other aspects of animal health and welfare
Although direct effects on components of the reproductive system are often among the most noticeable adverse effects of pollutants, animal performance and welfare can also be compromised by sub-optimal function in other physiological systems. Milk production and associated success in rearing offspring are critical to healthy populations. Rodent studies have shown that mammary development, differentiation and gene expression can be perturbed by exposure to organic pollutants before or around puberty (Fenton, Reference Fenton2006; Moral et al., Reference Moral, Wang, Russo, Lamartiniere, Pereira and Russo2008) and during pregnancy (Fenton, Reference Fenton2006), potentially compromising neonatal nutrition and survival. Similarly, studies of the mammary tissue of sheep exposed to sewage sludge (Fowler et al., Reference Fowler, Gordon, Thow, Cash, Miller, Lea and Rhind2007b) demonstrated changes in tissue structure and associated protein expression.
The capacity of environmental pollutants to adversely affect the immune system and the importance of exposure during foetal development, are well known from studies of species exposed to heavy pollutant burdens (Martineau et al., Reference Martineau, Lagace, Beland, Higgins, Armstrong and Shugart1988; De Swart et al., Reference De Swart, Ross, Timmerman, Vos, Reijnders, Vos and Osterhaus1995; Dietert and Piepenbrink, Reference Dietert and Piepenbrink2006). The complexity and individual variability of the immune response makes it difficult to detect when the suppression is modest, as is likely to be the case in ruminants, but it remains highly likely that EDC exposure is having adverse effects on immune function in this taxon as well.
EDC exposure can affect thyroid function and therefore metabolism; for example, EDCs have been shown to inhibit the expression of nuclear thyroid hormone receptors or perturb the hypothalamic-pituitary–thyroid axis (Jekat et al., Reference Jekat, Meisel, Eckard and Winterhoff1994; Hansen, Reference Hansen1998; Sugiyama et al., Reference Sugiyama, Shimada, Miyoshi and Yamauchi2005). In addition, PCBs acting via AhRs, can induce multiple histological and physiological changes within the gland, affecting thyroid hormone production (Hansen, Reference Hansen1998). As the thyroid gland is involved in the regulation of many fundamental physiological processes, particularly in the developing animal (Erenberg et al., Reference Erenberg, Omori, Menkes, Oh and Fisher1974), and in seasonal reproductive transitions (Shi and Barrell, Reference Shi and Barrell1992), its disruption could adversely affect animal health, welfare, reproduction and productivity.
Effects of exposure to environmental pollutants on bone structure have been identified previously in both wildlife (Lind et al., Reference Lind, Milnes, Lundberg, Bermudez, Orberg and Guillette2004a; Lundberg et al., Reference Lundberg, Fox, Wejheden, Lind, Larsson, Örberg and Lind2008) and domestic species (Lundberg et al., Reference Lundberg, Lyche, Ropstad, Aleksandersen, Ronn, Skaare, Larsson, Örberg and Lind2006) and recent work involving the sewage sludge paradigm has shown that exposure to a mixture of pollutants at low concentrations can increase mineral content and reduce bone strength, at least in females, (Lind et al., Reference Lind, Gustavsson, Hermsen, Larsson, Kyle, Örberg and Rhind2009).
Studies of humans also indicate that environmental pollutants can affect adipogenesis (Stahlhut et al., Reference Stahlhut, van Wijngaarden, Dye, Cook and Swan2007). Experiments involving various species indicate that phthalate exposure can induce insulin resistance and alter receptor activity, glucose transporters and transcription factors (Alonso-Magdalena et al., Reference Alonso-Magdalena, Laribi, Ropero, Fuentes, Ripoli, Soria and Nadal2005; Fujiyoshi et al., Reference Fujiyoshi, Michalek and Matsumura2006; Grun et al., Reference Grun, Watanabe, Zamanian, Maeda, Arima, Cubacha, Gardiner, Kanno, Iguchi and Blumberg2006). Thus, it seems likely that subtle changes in nutrient partitioning and in feed efficiency will occur in EDC-exposed ruminants although they are, as yet, undetected.
Exposure of rats to PCBs increased serum cholesterol concentrations and blood pressure, risk factors for heart disease (Lind et al., Reference Lind, Orberg, Edlund, Sjoblom and Lind2004b), and studies of humans suggest a relationship between dioxin exposure and risk of cardiovascular disease (Humblet et al., Reference Humblet, Birnbaum, Rimm, Mittleman and Hauser2008). Theoretically, such sub-clinical disorders have the potential to compromise ruminant health and welfare, causing small reductions in productivity.
One measure of neuroendocrine development is offspring behaviour. Altered patterns of behaviour have been reported in children exposed to various pollutants during pre-natal and early post-natal development (Vreugdenhil et al., Reference Vreugdenhil, Slijper, Mulder and Weisglas-Kuperus2002; Lanphear et al., Reference Lanphear, Hornung, Khoury, Yolton, Baghurst, Bellinger, Canfield, Dietrich, Bornschein, Greene, Rothenberg, Needleman, Schnaas, Wasserman, Graziano and Roberts2005; Korrick and Bellinger, Reference Korrick and Bellinger2007) and in lambs exposed to sewage sludge, via their dams (Erhard and Rhind, Reference Erhard and Rhind2004). Altered social or sexual behaviour, learning ability or fearfulness all have the potential to reduce animals’ capacity to obtain food, breed and compete successfully with others in the flock or herd.
In summary, environmental pollutants can adversely affect diverse physiological systems and processes in many species, including ruminants. These effects are not generally reflected in visible reductions in animal performance but sub-clinical effects may result in subtle reductions in animal performance, with associated economic consequences. Furthermore, such underlying physiological changes may become increasingly important as new chemicals are manufactured or as concentrations of others in the environment are increased. These add to the animal burden but readily observable effects may only become apparent if, or when, a critical level of the ‘insult’ is reached. For example, human sperm quality and fertility are reportedly declining over time (Nordstrom Joensen et al., Reference Nordstrom Joensen, Skakkebaek and Jorgensen2009) but effects on conception rates may become obvious only when the number of fertile sperm declines to a critical level. The fact that some effects are known to be exerted on the developing foetus and are expressed in the adult animal and, critically, also in subsequent generations (Bøgh et al., Reference Bøgh, Christensen, Dantzer, Groot, Thøfner, Rasmussen, Schmidt and Greve2001), means that exposures now may lead to animal production problems in the future.
Future research needs
In order to understand risks and effects, the input of pollutants into biological systems has to be defined. However, at present, measures of environmental concentrations are costly, often technically difficult, and generally limited to relatively few of the thousands of chemicals that may be important. Such measurements rarely take account of factors that can affect biological availability such as substrate binding or conversion into different forms with different chemical characteristics and/or biological effects.
Similarly, the processes regulating transfer of pollutants between the environment and the target organs, in animals at each stage of development, must be better characterised. The overall efficiency of this process depends on several components including the rate of ingestion or inhalation and pollutant availability, as determined by the strength of bonds between it and the substrate (food, soil, water and air). Transfer depends, also, on the efficiency of physiological processes such as uptake from the maternal gastrointestinal tract, lungs or skin and, following uptake by the dam, uptake from the maternal circulation via the placenta, rates of foetal metabolism, excretion and absorption into lipid stores (Rhind, Reference Rhind2008). These processes have been quantified for very few species or pollutant classes.
Understanding the effects of pollutants requires knowledge of actions at the cellular and molecular level. Different sets of genes are expressed while others are silenced, in a developmental stage and tissue-specific manner, by the co-ordinated action of a number of epigenetic mechanisms that involve chemical modifications to both DNA and chromatin (Li, Reference Li2002; Morgan et al., Reference Morgan, Santos, Green, Dean and Reik2005). The precedent that EDCs can epigenetically modify at least one of these modifications (i.e. DNA methylation) in the germ line, thereby promoting transgenerational abnormalities including impaired male fertility, has been established in rats exposed to the agricultural chemicals vinclozolin and methoxychlor (Anway et al., Reference Anway, Cupp, Uzumcu and Skinner2005). Although it remains to be determined if the effects of EDCs in ruminants operate by similarly dysregulating the normal pattern of epigenetic-mediated gene expression, a different ‘insult’, in the form of clinically relevant reductions in specific micronutrients during the peri-conceptional period, in sheep has been shown to lead to widespread epigenetic alterations to DNA methylation in offspring. Such alterations are associated with obesity, insulin resistance and high blood pressure (Sinclair et al., Reference Sinclair, Allegrucci, Singh, Gardner, Sebastian, Bispham, Thurston, Huntley, Rees, Maloney, Lea, Craigon, McEvoy and Young2007). Consequently, the focus of future studies on the effects of EDC exposure in ruminants will need to consider such modes of action.
The process of identifying causal relationships between different classes of pollutant and their effects has to be extended using numerous approaches already proven in ruminants. These include measurements of changes in organ structure and/or function (Evans et al., Reference Evans, North, Dye and Sweeney2004; Paul et al., Reference Paul, Rhind, Kyle, Scott, McKinnell and Sharpe2005) and in gene or protein expression (Edwards and Myers, Reference Edwards and Myers2007; Fowler et al., Reference Fowler, Dorà, McFerran, Amezaga, Miller, Lea, Cash, McNeilly, Evans, Cotinot, Sharpe and Rhind2008). However, current understanding of additive and synergistic effects of EDCs, especially in complex mixtures, is, at best, very limited and so elucidation of these effects will be a critical area of future research. As it is logistically impossible to address every possible combination, it will be appropriate to study combinations with different mechanisms of action in order to better comprehend the effects of mixtures (Kortenkamp, Reference Kortenkamp2007). There is also likely to be a significant role for bioinformatics and computer modelling approaches (Suk et al., Reference Suk, Olden and Yang2002). However, such studies may be partially constrained by lack of understanding of mechanisms of action and of data pertaining to the effects of each EDC, individually, let alone when part of a mixture.
The variability associated with each of the multiple processes regulating tissue concentrations of EDCs results in very large individual animal variation in tissue concentrations (Rhind, Reference Rhind2008). Investigation of the relationships between genotype and phenotypic responses might be expected to lead to improved predictability of effects but, owing to the complexity of mixtures and multiple genomic factors it is almost impossible for a single DNA variant site to be consistently associated with a particular trait (Nebert, Reference Nebert2005). This area of work is likely to be of major significance in the future, although frequently unpopular with funding agencies.
Although a number of physiological systems have already been shown to be affected by a wide range of chemicals, it is important to recognise not only that there may be chemicals, which are not yet widely recognised as endocrine disruptors, but also that there may be physiological responses to exposure that are not currently recognised as effects of EDC (Guillette, Reference Guillette2006). Thus, it is important that research into these phenomena is approached with an open mind and receptiveness to previously unidentified risks and mechanisms. The work of the European REACH (Registration, Evaluation and Authorisation of Chemicals) programme is designed to address the issue of chemical use and, by implication, environmental pollutants. Although it will undoubtedly help to identify and control the use of the most persistent, bioaccumulative and toxic chemicals, concerns remain that the legislation may fail to take account of effects of mixtures and of chemicals present at levels deemed to be below the ‘no effect level’ (Santillo and Johnston, Reference Santillo and Johnston2006).
Conclusions
Environmental pollutants can adversely affect animal health and reproductive function, through either direct or indirect effects on numerous organs and systems. However, empirical evidence of the relationships between exposure and physiological effects is scarce, particularly for ruminants, reflecting the fact that levels of exposure to each individual chemical are generally very low and they do not act individually. At this time, effects of environmentally relevant levels of exposure to EDCs are not yet reflected in visibly reduced animal performance. Nevertheless, concerns remain that there may be subtle perturbations of reproductive function and since some of the observed changes in physiological function may be expressed in subsequent generations, even without further exposure to pollutants, there may be even greater cause for concern. Like ruminant productivity, human health is generally considered to be good/improving but, at the same time, the incidence of breast cancer in women in the United Kingdom, a disease considered to be related to EDCs, is increasing at a rate of 2% every year (Office for National Statistics, 2008) indicating that some trends in health/performance may only be observed on a population basis rather than an individual basis. It is postulated that comparable insidious effects on ruminants may also be present but until appropriate end points are recognised and measured, such potential threats may remain hidden.
Acknowledgements
Much of the work reported in this paper, and the preparation of the paper, was funded by the Scottish Government Research and Analysis Directorate, the Welcome Trust (Contact no. 080388/Z/06/Z) and the European Commission Framework 7 Programme (Contract no. 212885).