Implications
Exposure of eggs to different wavelengths of light can influence embryonic development and have positive effects on production and welfare of broilers. Physiological processes underlying these effects are not understood. The present study demonstrates that exposure of eggs to light/dark regimen 12 : 12 with white, red, green and blue light selectively affects the rhythmic biosynthesis of melatonin. High amplitude was found in embryos incubated under red and white light while blue light resulted in the lowest melatonin levels. The rhythmic melatonin biosynthesis is suggested as a possible mechanism, which transfers information about the quality of light to the developing embryo.
Introduction
The incubation environment determines the hatching success and embryonic development of avian species. Environmental conditions during incubation can affect physiological processes and behaviour in birds, not only during their embryonic life but also after hatching. In hatchery practices, the temperature, humidity and CO2 content are of major importance and have been extensively studied (Noiva et al., Reference Noiva, Menezes and Peleteiro2014). However, the importance of lighting during incubation for hatching efficiency, chick quality and post-embryonic development has only recently been evaluated (Archer, Reference Archer2017 and Reference Archer2018; Tong et al., Reference Tong, McGonnell, Demmers, Roulston, Bergoug, Romanini, Verhelst, Guinebretière, Eterradossi, Berckmans and Exadaktylos2017).
In commercial hatcheries, eggs are incubated under constant environmental conditions (temperature, humidity, complete darkness) while in natural conditions, avian eggs are exposed to periodic changes in these parameters (Gwinner et al., Reference Gwinner, Zeman and Klaassen1997), as the mother leaves the nest for foraging. Therefore, it is possible that periodic changes in environmental variables during embryonic development may positively influence embryonic development and hatchability and even ‘program’ the postembryonic development of chicks with positive consequences on fitness in the wild and efficiency of production and welfare in poultry industry.
Previous studies have demonstrated that light during incubation can affect embryonic development, hatchability and the post-hatch development of chicks (Shafey, Reference Shafey2004; Archer and Mench, Reference Archer and Mench2014). Moreover, Ozkan et al. (Reference Ozkan, Yalçin, Babacanoglu, Uysal, Karadas and Kozanoglu2012) demonstrated that light during incubation better prepared chicks to deal with novel environments shortly after hatching. Recent data suggest that not only the duration and intensity of light, but also light wavelength, can significantly affect embryonic development, hatching success, post-embryonic growth and behaviour, although published results are limited. Recently, Archer (Reference Archer2017) demonstrated that white and red light during incubation improved hatching success in broiler eggs in comparison with green light or dark incubated eggs. Moreover, red and polychromatic white light reduced stress susceptibility, whereas green had no effect on this parameter.
The mechanisms, how light during incubation can influence embryonic development and hatchability are not known, but the hormone melatonin is one of candidates. Melatonin is produced in a rhythmic manner, with high concentrations during the night-time and low concentrations during the day-time, in both diurnal and nocturnal vertebrates (Cassone et al., Reference Cassone, Paulose, Whitfield-Rucker and Peters2009). It is generally accepted that the rhythmic production of melatonin in the pineal gland can transfer information about the ambient light/dark (LD) cycle to the internal environment and endocrine system.
Our previous studies demonstrated that the pineal gland already synthesises melatonin in a rhythmic manner during embryonic development under both in vivo (Zeman and Gwinner, Reference Zeman and Gwinner1993) and in vitro (Lamosova et al., Reference Lamosova, Zeman, Mackova and Gwinner1995) conditions. Circadian oscillations of pineal melatonin concentrations reflect the LD cycle during incubation and therefore can serve as a signal transferring information about ambient photoperiod, not only in hatched birds but also in avian embryos (Zeman and Herichova, Reference Zeman and Herichova2011).
Until now, there have been no data about the effects of different wavelengths of light on rhythmic melatonin production in the avian embryo. Therefore, the aim of the present study was: (1) to determine effects of monochromatic blue, green and red light as well as polychromatic white light on rhythmic melatonin biosynthesis in the pineal gland of 20-day-old chick embryos and (2) to evaluate if the incubation of embryos in different colours of light affects rhythmic melatonin production after hatching.
Material and methods
The study was conducted using Ross 308 broiler breeder (age 42 to 50 weeks) eggs (n=450; weight 64±2 g), which have light-brown eggshell (Mach Hydina, Budmerice, Slovakia). Eggs were incubated in two incubators MIDI F500 (PL Maschine KFT, Hungary) at standard temperature 37.5±0.2°C and humidity levels of 50% to 60% with automatic egg turning. In our experiment, eggs were incubated under constant darkness for the first 10 days of incubation since our previous studies showed that this period is not critical for melatonin rhythm development in chick embryos (Zeman et al., Reference Zeman, Gwinner, Herichova, Lamosova and Kostal1999a; Zeman and Herichova, Reference Zeman and Herichova2011). Afterwards, eggs were exposed to the LD regime of 12 : 12 h until hatching, with monochromatic red light in one and blue light in the second incubator. In the subsequent trial, monochromatic green and polychromatic white light were used in the respective incubators. Incubators were illuminated by light-emitting diode (LED) strips (Slov-Led Plus, Zvolen, Slovakia) emitting light with an average intensity of 0.04 W/m2. The strips were mounted on the top of incubators and emitted monochromatic red light at 632 nm, blue at 463 nm, green at 517 nm and polychromatic white light with maximum of 448 nm. Light intensity and chromatic temperature were measured using an illuminance spectrophotometer (CL-500A; Konica Minolta, Japan).
In experiment one, 20-day-old embryos were sampled over 24 h at times indicated in the Results section (4 to 6 per sampling time). Embryos were taken from the eggshell and decapitated under dim red light during the dark-time. Pineal glands were quickly excised, immediately frozen on dry ice (–70°C) and stored at –20°C until melatonin measurement.
In experiment two, eggs were incubated under identical conditions to experiment 1 and were exposed to either monochromatic green or polychromatic white light during the L phase of the cycle. The pineal glands were collected from 20-day-old embryos during the light phase at ZT10 (Zeitgeber time 0 is defined as light onset) and during the dark phase (ZT16 and ZT19), when high melatonin biosynthesis is expected. A subgroup of eggs (34 and 44 for the white and green light, respectively) was allowed to hatch under respective light wavelength. Afterwards, chicks were kept in the incubators under the same lighting conditions as experienced during incubation and pineal glands of 2-day-old chicks were collected during the light (ZT10) – and dark-time (ZT16 and ZT19).
Melatonin from pineal glands was extracted with methanol and melatonin levels were measured by radioimmunoassay according to the methods validated for pineal extracts (Zeman et al., Reference Zeman, Gwinner, Herichova, Lamosova and Kostal1999a). Samples were measured in three assays with intra- and inter-assay variation coefficients of 4.3% and 14.1%, respectively. The sensitivity of the assay was 9.0 pg/pineal gland. The experiment was approved by the Ethical Committee at the Institute of Animal Biochemistry and Genetics, Slovak Academy of Sciences and State Veterinary Authority for the Slovak Republic.
Statistical analysis
Variations of pineal melatonin concentrations over 24 h were evaluated by cosinor analysis performed in R (R Development Core Team, 2017) using the cosinor package (Sachs, Reference Sachs2014). Mesor (the 24-h time series mean), amplitude (one-half the peak-to-trough difference) and acrophase (the peak time of the fitted curve) were derived from the cosinor analysis. A significant 24-h rhythm was detected with an F-test by rejection of the zero-amplitude hypothesis. The acrophase and amplitude of significant melatonin rhythms were compared between light treatments by Wald tests. Differences in mesors were considered significant if the corresponding confidence intervals did not overlap. A comparison of embryonic and posthatching pineal melatonin concentrations in white- and green-light treatments during the incubation was performed with a three-way ANOVA followed by Fisher’s LSD posthoc tests. Developmental stage, light treatment, ZT and respective interactions were included as fixed factors. Pineal melatonin levels were logarithmically transformed to approach the normal distribution required for ANOVA.
Results
On embryonic day 20 (ED20), pineal melatonin levels of chick embryos incubated under LD 12 : 12 h exhibited a significant 24-h rhythm with acrophase during the dark phase (between ZT18 and ZT20) in all treatment groups (Figure 1, Table 1). The amplitude of pineal melatonin rhythm was the lowest in the blue-light incubated embryos compared with the green-, red- and white-light treatments. Moreover, the amplitude of melatonin rhythm in the green-light group was higher than in the blue- and lower than in both the red- and white-light groups. No differences were found between the red- and white-light treatments. Mesor of the circadian pineal melatonin rhythm was also higher in white- and red-light incubated embryos as compared to the green and blue light treatments (Table 1).
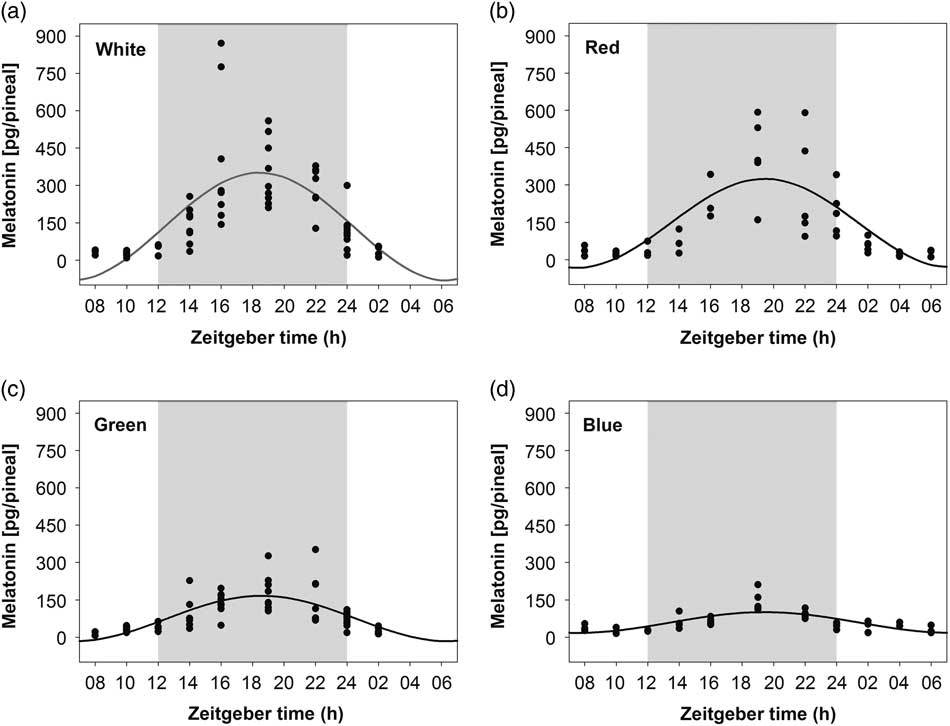
Figure 1 Daily profile of pineal melatonin concentrations in 20-day-old chick embryos. Eggs were incubated under the light–dark cycle 12 : 12 h using white (a), red (b), green (c) or blue (d) light during the light phase. Time is expressed as Zeitgeber time with n=3 to 11 per time point. Significant 24-h rhythms are depicted with the best fitting cosine curve. Shaded area indicates the dark phase.
Table 1 Cosinor analysis of 24-h melatonin rhythms in the pineal gland of 20-day-old chick embryos incubated under white, red, green or blue light during the light phase of the light-dark cycle 12 : 12 h

Upper and lower confidence intervals for mesor, amplitude and acrophase are given in brackets.
R 2, F-tests and P-values describe significance of 24-h rhythms.
a,b,cDifferent superscript letters within column represent significant differences between light treatments (P<0.001).
Pineal melatonin concentrations significantly increased on post-hatching day two (PD2) compared with pre-hatching levels (F (1,90)=184.18; P<0.001). This increase was substantial especially in night-time melatonin levels at ZT16 and ZT19 (Figure 2). The ANOVA revealed a significant interaction between developmental stage, light treatment and ZT (F (1,90)=7.38; P<0.01) showing that the green light resulted in lower pineal melatonin concentrations than the white-light group in embryos (ED20) at ZT16 and ZT19, while no differences between light treatments were found in hatched chicks on PD2 (Figure 2).
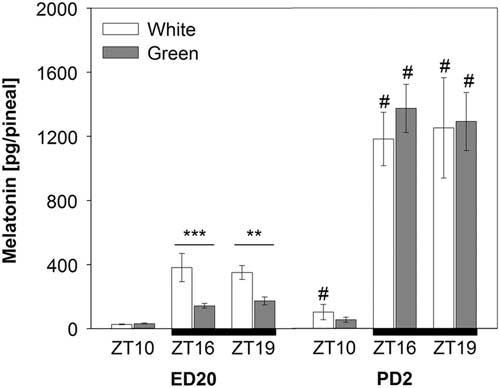
Figure 2 Pineal melatonin concentrations in 20-day-old chick embryos (ED20) and 2-day-old chicks (PD2). Eggs were incubated under the light–dark cycle 12 : 12 h using white or green light during the light phase. Time is expressed as Zeitgeber time (ZT; ZT0=light on) with n=4 to 11 per time point. Black bars at the bottom line represent the dark phase. Data are given as mean±SEM. **P<0.01 and ***P<0.001 for differences between white- and green-light groups. # P<0.001 for differences between ED20 and PD2 within respective ZT and light treatment.
Discussion
Our results demonstrated that the melatonin biosynthesis in the pineal gland of 20-day-old chick embryos is rhythmic and can selectively respond to different wavelengths of light during incubation. Results are in line with our previous data, demonstrating that chick embryos incubated under polychromatic white light develop circadian melatonin production during the final stages of embryonic life (Zeman and Gwinner, Reference Zeman and Gwinner1993; Zeman et al., Reference Zeman, Gwinner, Herichova, Lamosova and Kostal1999a). This is probably an adaptation of avian embryos to extrauterine development without humoral links to the maternal organism (Zeman and Herichova, Reference Zeman and Herichova2011). In contrast, the mammalian embryo benefits from the maternal melatonin rhythm, which can synchronise its physiological processes and therefore, circadian melatonin production begins after birth in mammals (Reppert, Reference Reppert1995).
We found the expected daily rhythm of pineal melatonin concentrations with higher dark-time than light-time levels under all lighting conditions. The maximum amplitude of pineal melatonin rhythm was found in embryos incubated under red and white light and the amplitude was lower in embryos incubated under green light. The incubation under blue light induced the lowest amplitude of melatonin rhythm. Different wavelengths of light during incubation may selectively influence embryonic and postembryonic development of avian embryos although published results are unequivocal. Originally, in ovo intermittent photostimulation with monochromatic green light, from day 5 of incubation until hatching, was reported to augment embryonic and post-hatch development, as well as muscle growth in broilers (Rozenboim et al., Reference Rozenboim, Biran, Chaiseha, Yahav, Rosenstrauch, Sklan and Halevy2004). However, it was recently reported that incubation under a 12L : 12D schedule of green light did not produce significant effects in comparison with incubation in constant darkness, while incubation of broiler eggs under white and red light improved not only hatchability and chick quality but also the post-hatch welfare of broiler chickens by decreasing their fear responses and stress susceptibility (Archer, Reference Archer2017). Embryos incubated during the first 18 days under continuous green light with an intensity of 1340 to 1730 lx exhibited increased growth and hatchability compared with dark-incubated eggs (Shafey and Al-Mohsen, Reference Shafey and Al-Mohsen2002). In another study, a 12L : 12D regime of green light during 18 days of incubation decreased hatchability and accelerated embryonic development in comparison with control dark incubation (Tong et al., Reference Tong, McGonnell, Demmers, Roulston, Bergoug, Romanini, Verhelst, Guinebretière, Eterradossi, Berckmans and Exadaktylos2017). The authors explain their finding by effects of light treatment on alterations of hormonal profiles during the hatching process. They measured melatonin concentrations in plasma and found the rhythmic profile of plasma melatonin concentrations, which peaked at the end of the dark period. In comparison with the pineal concentrations measured in the present study, those authors report a lower amplitude of plasma melatonin levels. The results demonstrate that in addition to wavelengths of light, additional variables, such as colour of egg shell and LD regimen can be important and must be considered in future studies.
Although the wavelength of light during incubation can potentially influence embryonic development in birds, underlying physiological mechanisms are completely unknown. One of potential mechanisms could involve rhythmic melatonin production. Interestingly, red and polychromatic white light resulted in the most pronounced melatonin rhythm in our study and maximal effects on post-hatch performance in the study of Archer (Reference Archer2017). The mechanisms of how melatonin can influence reported parameters are not understood but melatonin was found to influence growth hormone production in birds (Zeman et al., Reference Zeman, Buyse, Lamosova, Herichova and Decuypere1999b). In mammalian species, melatonin is known for its pleiotropic effects (Hardeland et al., Reference Hardeland, Cardinali, Srinivasan, Spence, Brown and Pandi-Perumal2011) and has the potential to modulate the development and metabolism of the skeletal system (Dimitri and Rosen, Reference Dimitri and Rosen2017; van der Pol et al., Reference van der Pol, van Roovert-Reijrink, Aalbers, Kemp and van den Brand2017). Moreover, melatonin was suggested to have epigenetic effects (Korkmaz et al., Reference Korkmaz, Rosales-Corral and Reiter2012) and in this way can influence the manifestation of a broad spectrum of genes.
The spectral sensitivity of the melatonin biosynthesis we found in embryos differs from that found in post-hatched chicks. Pineal melatonin levels increased substantially during 2 post-embryonic days in comparison with embryos. However, in contrast to embryos, 2-day-old chicks incubated and kept either under green or white light did not differ in pineal melatonin concentrations. Higher circadian amplitude of plasma melatonin rhythm was reported in 7- and 14-day-old chickens kept in green light than in both blue and white light (Jin et al., Reference Jin, Jia, Li, Yang, Wang, Cao and Chen2011). The mechanisms behind this different colour sensitivity of embryonic and post-embryonic circadian system are not clear yet. It was found that green light exposure during incubation down-regulated expression of green and red opsin while red light worked in the opposite direction (Rozenboim et al., Reference Rozenboim, El Halawani, Kashash, Piestun and Halevy2013). These changes can be important for subsequent sensitivity to light during post-embryonic development but can hardly explain observed effects of embryo exposure to different light colours on postnatal development and behaviour. Moreover, different components of the avian circadian system (the pineal and retina) can have different spectral sensitivity or eggshell can selectively filter the light spectrum. Indeed, Archer (Reference Archer2017) proved that the white LED spectrum is filtered by broiler eggshell while red and green LED lights are not changed when transferred through broiler eggshell.
Different transparency of eggshell to different wavelengths of light can be environmentally important since different shell colours can selectively filter the polychromatic light to which embryos are exposed. Pigments of eggshell either admit penetration or block different wavelengths of light spectra (Maurer et al., Reference Maurer, Portugal and Cassey2011). The blue-green eggshell colour is caused by deposition of pigment biliverdin while red-brown eggshell maculation is generated by protoporphyrin (Miksik et al., Reference Miksik, Holan and Deyl1996). Deposition of both pigments is in a reciprocal relationship. The blue-red biliverdin-based coloration could be related to better female conditions (Moreno et al., Reference Moreno, Lobato, Morales, Merino, Tomas, Martinez-de la Puente, Sanz, Mateo and Soler2006) and ensure better egg quality. Conversely, food restricted canary females laid eggs with higher eggshell chroma and protoporphyrin concentrations, which can modify the association between female body condition and intensity of blue-green coloration (Hargitai et al., Reference Hargitai, Boross, Nyiri and Eke2018). However, in our study we used light-brown shell eggs to avoid complications with filtering of different wavelengths of light.
Modulation of melatonin production is not the only possible way that light during incubation could influence embryonic development. Avian molecular clockworks in the pineal gland and the suprachiasmatic nucleus start to develop during embryonic life (Zeman and Herichova, Reference Zeman and Herichova2011). Expression of the clock gene per2 is rhythmic in the pineal gland of 19-day-old chick embryos incubated in the LD cycle (Okabayashi et al., Reference Okabayashi, Yasuo, Watanabe, Namikawa, Ebihara and Yoshimura2003) and expression of this gene is directly inducible by light during the subjective day in the embryonic pineal gland (Herichova et al., Reference Herichova, Monosikova and Zeman2007). Peripheral oscillators develop postnatally and a robust per2 and bmal1 expression was observed in the heart and liver of 4-day-old chicks (Zeman et al., Reference Zeman, Szantoova and Herichova2009) and selectively respond to different monochromatic lights (Jiang et al., Reference Jiang, Wang, Cao, Dong and Chen2017).
In summary, pineal glands of chick embryos are selectively sensitive to different wavelengths of light. Exposure to red and white light during incubation resulted in the maximum amplitude of pineal melatonin rhythm, while embryos incubated under green and blue light exhibited a low amplitude of melatonin rhythm. Postembryonic exposure to green light induced a similar increase of melatonin biosynthesis as white light. Our results suggest the rhythmic melatonin biosynthesis as a mechanism, which transfers information about the quality of ambient light to the developing avian embryo.
Acknowledgements
This study was supported by a grant APVV-17-0371 and VEGA 1/0501/19. The authors thank the company Mach Hydina, Budmerice, Slovakia for hatching eggs and Slov-Led Plus, Zvolen, Slovakia for LED lights.
Declaration of interest
Authors declare no conflicts of interest.
Ethics statement
All procedures were carried out in accordance with the laws and regulations of the Slovak Republic, and approved by the Ethical Committee of the Institute of Animal Biochemistry and Genetics, Centre of Biosciences, Slovak Academy of Sciences, Bratislava and Slovak Veterinary Authority.
Software and data repository resources
None of the data were deposited in an official repository.