Book contents
- Source-to-Sink Fluxes in Undisturbed Cold Environments
- Source-to-Sink Fluxes in Undisturbed Cold Environments
- Copyright page
- Contents
- Contributors
- Preface
- Part I Solute and sedimentary fluxes and budgets in changing cold climate environments
- Part II Climate change in cold environments and general implications for contemporary solute and sedimentary fluxes
- Part III Solute and sedimentary fluxes in subarctic and Arctic environments
- Part IV Solute and sedimentary fluxes in sub-Antarctic and Antarctic environments
- Part V Solute and sedimentary fluxes in alpine/mountain environments
- Part VI Quantitative analysis of solute and sedimentary fluxes in cold climate environments
- Index
- References
Part V - Solute and sedimentary fluxes in alpine/mountain environments
Published online by Cambridge University Press: 05 July 2016
- Source-to-Sink Fluxes in Undisturbed Cold Environments
- Source-to-Sink Fluxes in Undisturbed Cold Environments
- Copyright page
- Contents
- Contributors
- Preface
- Part I Solute and sedimentary fluxes and budgets in changing cold climate environments
- Part II Climate change in cold environments and general implications for contemporary solute and sedimentary fluxes
- Part III Solute and sedimentary fluxes in subarctic and Arctic environments
- Part IV Solute and sedimentary fluxes in sub-Antarctic and Antarctic environments
- Part V Solute and sedimentary fluxes in alpine/mountain environments
- Part VI Quantitative analysis of solute and sedimentary fluxes in cold climate environments
- Index
- References
Summary
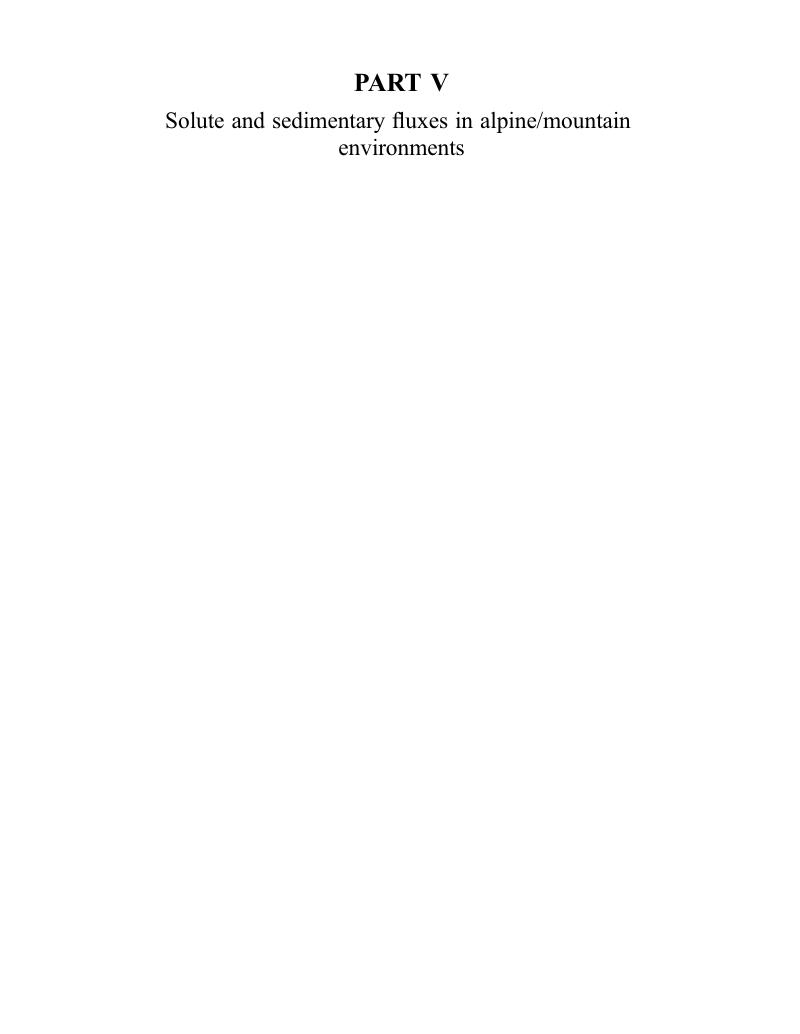
- Type
- Chapter
- Information
- Source-to-Sink Fluxes in Undisturbed Cold Environments , pp. 273 - 382Publisher: Cambridge University PressPrint publication year: 2016