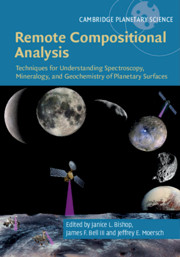
Book contents
- Remote Compositional Analysis
- Cambridge Planetary Science
- Remote Compositional Analysis
- Copyright page
- Contents
- Contributors
- Foreword
- Preface
- Acknowledgments
- Part I Theory of Remote Compositional Analysis Techniques and Laboratory Measurements
- Part II Terrestrial Field and Airborne Applications
- Part III Analysis Methods
- Part IV Applications to Planetary Surfaces
- 17 Spectral Analyses of Mercury
- 18 Compositional Analysis of the Moon in the Visible and Near-Infrared Regions
- 19 Spectral Analyses of Asteroids
- 20 Visible and Near-Infrared Spectral Analyses of Asteroids and Comets from Dawn and Rosetta
- 21 Spectral Analyses of Saturn’s Moons Using the Cassini Visual Infrared Mapping Spectrometer
- 22 Spectroscopy of Pluto and Its Satellites
- 23 Visible to Short-Wave Infrared Spectral Analyses of Mars from Orbit Using CRISM and OMEGA
- 24 Thermal Infrared Spectral Analyses of Mars from Orbit Using the Thermal Emission Spectrometer and Thermal Emission Imaging System
- 25 Thermal Infrared Remote Sensing of Mars from Rovers Using the Miniature Thermal Emission Spectrometer
- 26 Compositional and Mineralogic Analyses of Mars Using Multispectral Imaging on the Mars Exploration Rover, Phoenix, and Mars Science Laboratory Missions
- 27 Mössbauer Spectroscopy at Gusev Crater and Meridiani Planum
- 28 Elemental Analyses of Mars from Rovers Using the Alpha-Particle X-Ray Spectrometer
- 29 Elemental Analyses of Mars from Rovers with Laser-Induced Breakdown Spectroscopy by ChemCam and SuperCam
- 30 Neutron, Gamma-Ray, and X-Ray Spectroscopy of Planetary Bodies
- 31 Radar Remote Sensing of Planetary Bodies
- Index
- References
19 - Spectral Analyses of Asteroids
from Part IV - Applications to Planetary Surfaces
Published online by Cambridge University Press: 15 November 2019
- Remote Compositional Analysis
- Cambridge Planetary Science
- Remote Compositional Analysis
- Copyright page
- Contents
- Contributors
- Foreword
- Preface
- Acknowledgments
- Part I Theory of Remote Compositional Analysis Techniques and Laboratory Measurements
- Part II Terrestrial Field and Airborne Applications
- Part III Analysis Methods
- Part IV Applications to Planetary Surfaces
- 17 Spectral Analyses of Mercury
- 18 Compositional Analysis of the Moon in the Visible and Near-Infrared Regions
- 19 Spectral Analyses of Asteroids
- 20 Visible and Near-Infrared Spectral Analyses of Asteroids and Comets from Dawn and Rosetta
- 21 Spectral Analyses of Saturn’s Moons Using the Cassini Visual Infrared Mapping Spectrometer
- 22 Spectroscopy of Pluto and Its Satellites
- 23 Visible to Short-Wave Infrared Spectral Analyses of Mars from Orbit Using CRISM and OMEGA
- 24 Thermal Infrared Spectral Analyses of Mars from Orbit Using the Thermal Emission Spectrometer and Thermal Emission Imaging System
- 25 Thermal Infrared Remote Sensing of Mars from Rovers Using the Miniature Thermal Emission Spectrometer
- 26 Compositional and Mineralogic Analyses of Mars Using Multispectral Imaging on the Mars Exploration Rover, Phoenix, and Mars Science Laboratory Missions
- 27 Mössbauer Spectroscopy at Gusev Crater and Meridiani Planum
- 28 Elemental Analyses of Mars from Rovers Using the Alpha-Particle X-Ray Spectrometer
- 29 Elemental Analyses of Mars from Rovers with Laser-Induced Breakdown Spectroscopy by ChemCam and SuperCam
- 30 Neutron, Gamma-Ray, and X-Ray Spectroscopy of Planetary Bodies
- 31 Radar Remote Sensing of Planetary Bodies
- Index
- References
Summary
Asteroids represent a time capsule, storing information about the composition of and conditions in the solar nebula as well as processes that have affected the Solar System. The asteroid population includes primitive bodies, partially melted material, and the result of full melting and differentiation of planetesimals. Asteroidal minerals, organic molecules, and ices that are relevant to uncovering the history of the Solar System are accessible spectroscopically. Reflection and thermal emission spectroscopy from ground-based telescopes, space telescopes, and spacecraft provide a rich view of asteroid surfaces. Analysis techniques, including taxonomic classification, direct comparisons to meteorites and pure materials, band parameter analysis, and physical models of light scattering, are customized to the specific science question under study. In recent years, spacecraft missions to asteroids have provided ground-truth to more remote spectral analyses, corroborating many inferences from ground-based observations, while enabling new discoveries and a richer, deeper view of asteroid surfaces. These compositional studies provide an important complement to and constraint on dynamical investigations of Solar System evolution. The future of asteroid science is bright, with advances expected in the areas of sample return, additional reconnaissance missions, improved wavelength coverage spectroscopy, and significant increases in the size of the database of asteroid spectra.
Keywords
- Type
- Chapter
- Information
- Remote Compositional AnalysisTechniques for Understanding Spectroscopy, Mineralogy, and Geochemistry of Planetary Surfaces, pp. 393 - 412Publisher: Cambridge University PressPrint publication year: 2019
References
- 1
- Cited by