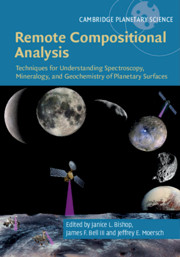
Book contents
- Remote Compositional Analysis
- Cambridge Planetary Science
- Remote Compositional Analysis
- Copyright page
- Contents
- Contributors
- Foreword
- Preface
- Acknowledgments
- Part I Theory of Remote Compositional Analysis Techniques and Laboratory Measurements
- 1 Electronic Spectra of Minerals in the Visible and Near-Infrared Regions
- 2 Theory of Reflectance and Emittance Spectroscopy of Geologic Materials in the Visible and Infrared Regions
- 3 Mid-infrared (Thermal) Emission and Reflectance Spectroscopy
- 4 Visible and Near-Infrared Reflectance Spectroscopy
- 5 Spectroscopy of Ices, Volatiles, and Organics in the Visible and Infrared Regions
- 6 Raman Spectroscopy
- 7 Mössbauer Spectroscopy
- 8 Laser-Induced Breakdown Spectroscopy
- 9 Neutron, Gamma-Ray, and X-Ray Spectroscopy
- 10 Radar Remote Sensing
- Part II Terrestrial Field and Airborne Applications
- Part III Analysis Methods
- Part IV Applications to Planetary Surfaces
- Index
- References
3 - Mid-infrared (Thermal) Emission and Reflectance Spectroscopy
Laboratory Spectra of Geologic Materials
from Part I - Theory of Remote Compositional Analysis Techniques and Laboratory Measurements
Published online by Cambridge University Press: 15 November 2019
- Remote Compositional Analysis
- Cambridge Planetary Science
- Remote Compositional Analysis
- Copyright page
- Contents
- Contributors
- Foreword
- Preface
- Acknowledgments
- Part I Theory of Remote Compositional Analysis Techniques and Laboratory Measurements
- 1 Electronic Spectra of Minerals in the Visible and Near-Infrared Regions
- 2 Theory of Reflectance and Emittance Spectroscopy of Geologic Materials in the Visible and Infrared Regions
- 3 Mid-infrared (Thermal) Emission and Reflectance Spectroscopy
- 4 Visible and Near-Infrared Reflectance Spectroscopy
- 5 Spectroscopy of Ices, Volatiles, and Organics in the Visible and Infrared Regions
- 6 Raman Spectroscopy
- 7 Mössbauer Spectroscopy
- 8 Laser-Induced Breakdown Spectroscopy
- 9 Neutron, Gamma-Ray, and X-Ray Spectroscopy
- 10 Radar Remote Sensing
- Part II Terrestrial Field and Airborne Applications
- Part III Analysis Methods
- Part IV Applications to Planetary Surfaces
- Index
- References
Summary
Middle infrared (~2000 to 200 cm–1 or 5 to 50 μm) data are extremely useful for compositional determination of geologic materials because this wavelength region hosts the fundamental (“Reststrahlen”) vibrational bands of most minerals. Analysis of remotely sensed data requires comparison to well-developed spectral libraries populated with a wide variety of mid-IR mineral spectra (and additional rock or meteorite spectra). Here we present the theory behind molecular vibrations of mineral structures and the simple harmonic oscillators that define them mathematically. We present dispersion theory that describes how energy travels through a crystal and how propagating energy is affected by the crystal lattice structure, specifically along the various crystal axes. The equipment required for these types of laboratory measurements (both emissivity and reflectivity) is presented as well as a discussion about how mid-IR data are affected by particle size and how related volume scattering affects spectral data. Finally, mid-IR emissivity spectra acquired in a dry, 1-atm environment are provided for 93 different minerals and meteorites. These spectra are available as ancillary data files.
Keywords
- Type
- Chapter
- Information
- Remote Compositional AnalysisTechniques for Understanding Spectroscopy, Mineralogy, and Geochemistry of Planetary Surfaces, pp. 42 - 67Publisher: Cambridge University PressPrint publication year: 2019
References
- 10
- Cited by