Book contents
- Planetesimals
- Cambridge Planetary Science
- Planetesimals
- Copyright page
- Contents
- Contributors
- 1 Planetesimals
- Part One Dynamical Evolution
- Part Two Chemical and Mineralogical Diversity
- 4 Differentiation Under Highly Reducing Conditions: New Insights from Enstatite Meteorites and Mercury
- 5 Origin and Evolution of Volatile-rich Asteroids
- 6 Silicate Melting and Volatile Loss During Differentiation in Planetesimals
- 7 Iron and Stony-iron Meteorites: Evidence for the Formation, Crystallization, and Early Impact Histories of Differentiated Planetesimals
- 8 Arguments for the Non-existence of Magma Oceans in Asteroids
- 9 Magnetic Fields on Asteroids and Planetesimals
- 10 Magnetic Mineralogy of Meteoritic Metal: Paleomagnetic Evidence for Dynamo Activity on Differentiated Planetesimals
- 11 Chronology of Planetesimal Differentiation
- 12 Stable Isotope Evidence for the Differentiation and Evolution of Planetesimals
- Part Three Asteroids as Records of Formation and Differentiation
- Part Four Early Differentiation and Consequences for Planet Formation
- Index
- References
11 - Chronology of Planetesimal Differentiation
from Part Two - Chemical and Mineralogical Diversity
Published online by Cambridge University Press: 25 February 2017
- Planetesimals
- Cambridge Planetary Science
- Planetesimals
- Copyright page
- Contents
- Contributors
- 1 Planetesimals
- Part One Dynamical Evolution
- Part Two Chemical and Mineralogical Diversity
- 4 Differentiation Under Highly Reducing Conditions: New Insights from Enstatite Meteorites and Mercury
- 5 Origin and Evolution of Volatile-rich Asteroids
- 6 Silicate Melting and Volatile Loss During Differentiation in Planetesimals
- 7 Iron and Stony-iron Meteorites: Evidence for the Formation, Crystallization, and Early Impact Histories of Differentiated Planetesimals
- 8 Arguments for the Non-existence of Magma Oceans in Asteroids
- 9 Magnetic Fields on Asteroids and Planetesimals
- 10 Magnetic Mineralogy of Meteoritic Metal: Paleomagnetic Evidence for Dynamo Activity on Differentiated Planetesimals
- 11 Chronology of Planetesimal Differentiation
- 12 Stable Isotope Evidence for the Differentiation and Evolution of Planetesimals
- Part Three Asteroids as Records of Formation and Differentiation
- Part Four Early Differentiation and Consequences for Planet Formation
- Index
- References
Summary
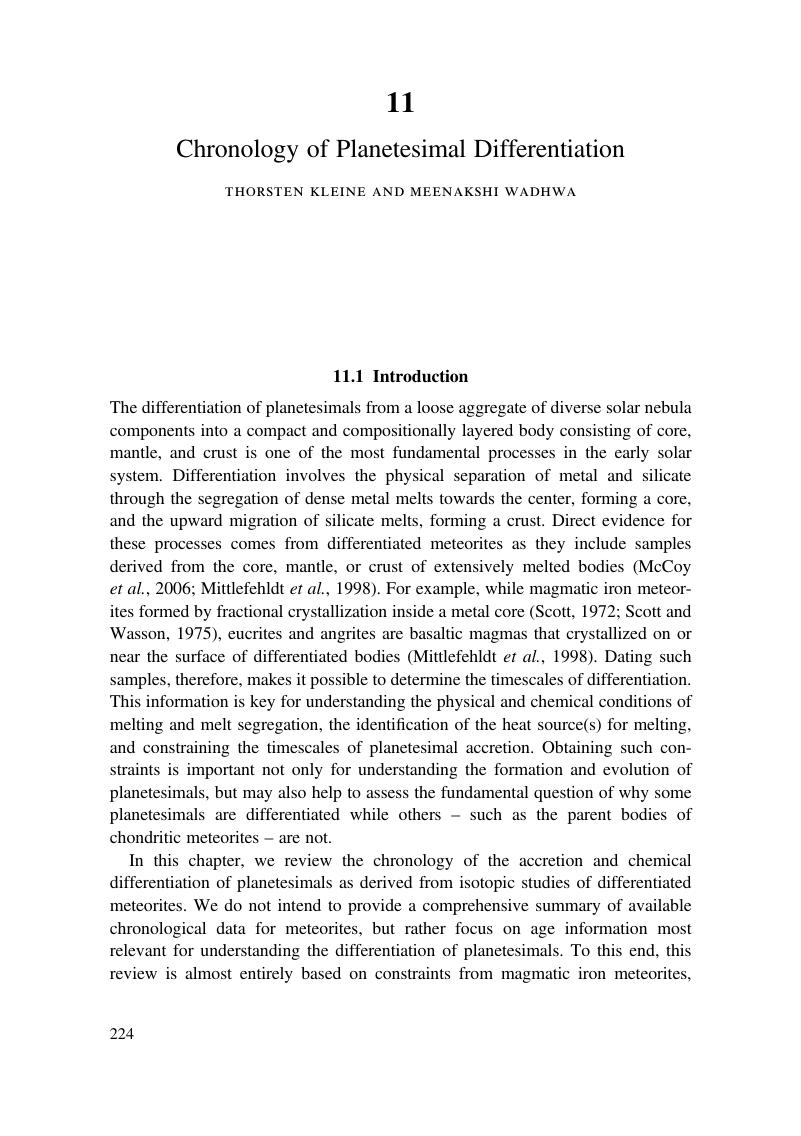
- Type
- Chapter
- Information
- PlanetesimalsEarly Differentiation and Consequences for Planets, pp. 224 - 245Publisher: Cambridge University PressPrint publication year: 2017
References
- 9
- Cited by