Book contents
- Pediatric Nephropathology & Childhood Kidney Tumors
- Diagnostic Pediatric Pathology
- Pediatric Nephropathology & Childhood Kidney Tumors
- Copyright page
- Dedication
- Contents
- Contributors
- Preface
- Section 1 Normal and Abnormal Human Kidney Development
- Section 2 Glomerular Diseases
- Section 3 Tubulointerstitial Diseases
- Section 4 Vascular Diseases
- Section 5 Infectious Diseases
- Section 6 Cystic Diseases
- Section 7 Solid Tumors of the Kidney
- Section 8 Transplant Pathology of the Kidney
- Index
- References
Section 6 - Cystic Diseases
Published online by Cambridge University Press: 10 August 2023
- Pediatric Nephropathology & Childhood Kidney Tumors
- Diagnostic Pediatric Pathology
- Pediatric Nephropathology & Childhood Kidney Tumors
- Copyright page
- Dedication
- Contents
- Contributors
- Preface
- Section 1 Normal and Abnormal Human Kidney Development
- Section 2 Glomerular Diseases
- Section 3 Tubulointerstitial Diseases
- Section 4 Vascular Diseases
- Section 5 Infectious Diseases
- Section 6 Cystic Diseases
- Section 7 Solid Tumors of the Kidney
- Section 8 Transplant Pathology of the Kidney
- Index
- References
Summary
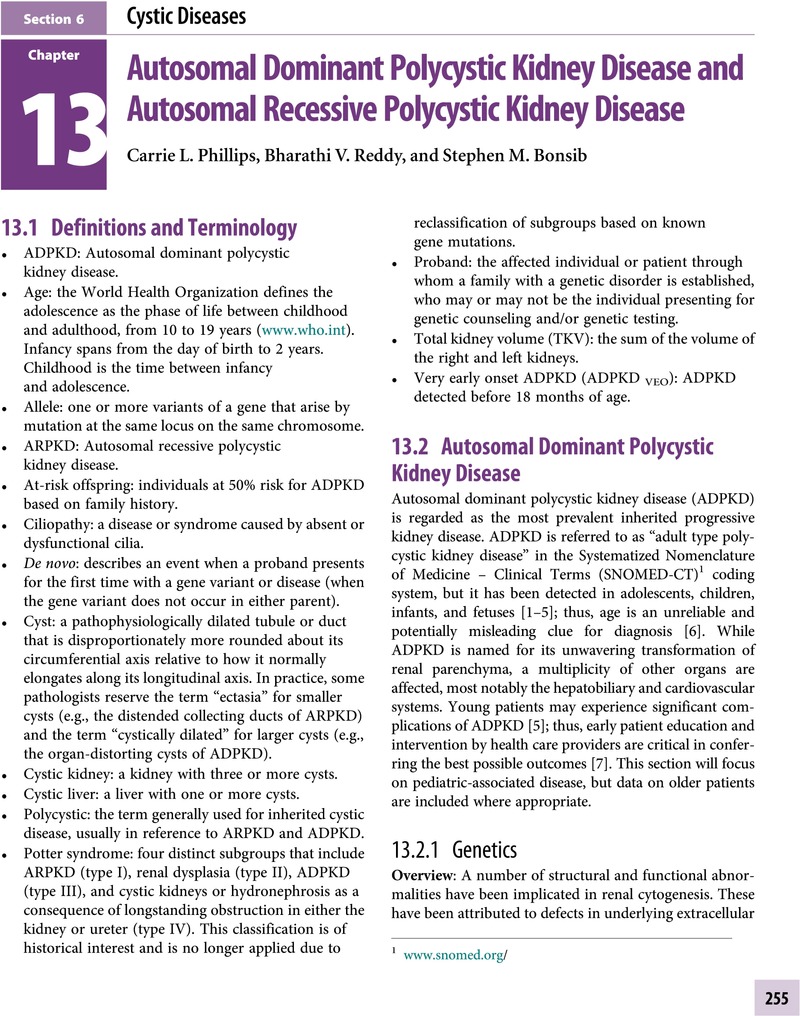
- Type
- Chapter
- Information
- Pediatric Nephropathology & Childhood Kidney Tumors , pp. 255 - 298Publisher: Cambridge University PressPrint publication year: 2023