Book contents
- Frontmatter
- Contents
- List of contributors
- Preface
- Part I Introduction to stem cells and regenerative medicine
- Part II Porous scaffolds for regenerative medicine
- Part III Hydrogel scaffolds for regenerative medicine
- Part IV Biological factor delivery
- 20 Growth factor delivery on scaffolds
- 21 Gene delivery for periodontal regeneration
- 22 Endogenous stem/progenitor cell recruitment for tissue regeneration
- 23 Affinity-based delivery systems
- 24 DNA delivery for regeneration
- Part V Animal models and clinical applications
- Index
- References
23 - Affinity-based delivery systems
from Part IV - Biological factor delivery
Published online by Cambridge University Press: 05 February 2015
- Frontmatter
- Contents
- List of contributors
- Preface
- Part I Introduction to stem cells and regenerative medicine
- Part II Porous scaffolds for regenerative medicine
- Part III Hydrogel scaffolds for regenerative medicine
- Part IV Biological factor delivery
- 20 Growth factor delivery on scaffolds
- 21 Gene delivery for periodontal regeneration
- 22 Endogenous stem/progenitor cell recruitment for tissue regeneration
- 23 Affinity-based delivery systems
- 24 DNA delivery for regeneration
- Part V Animal models and clinical applications
- Index
- References
Summary
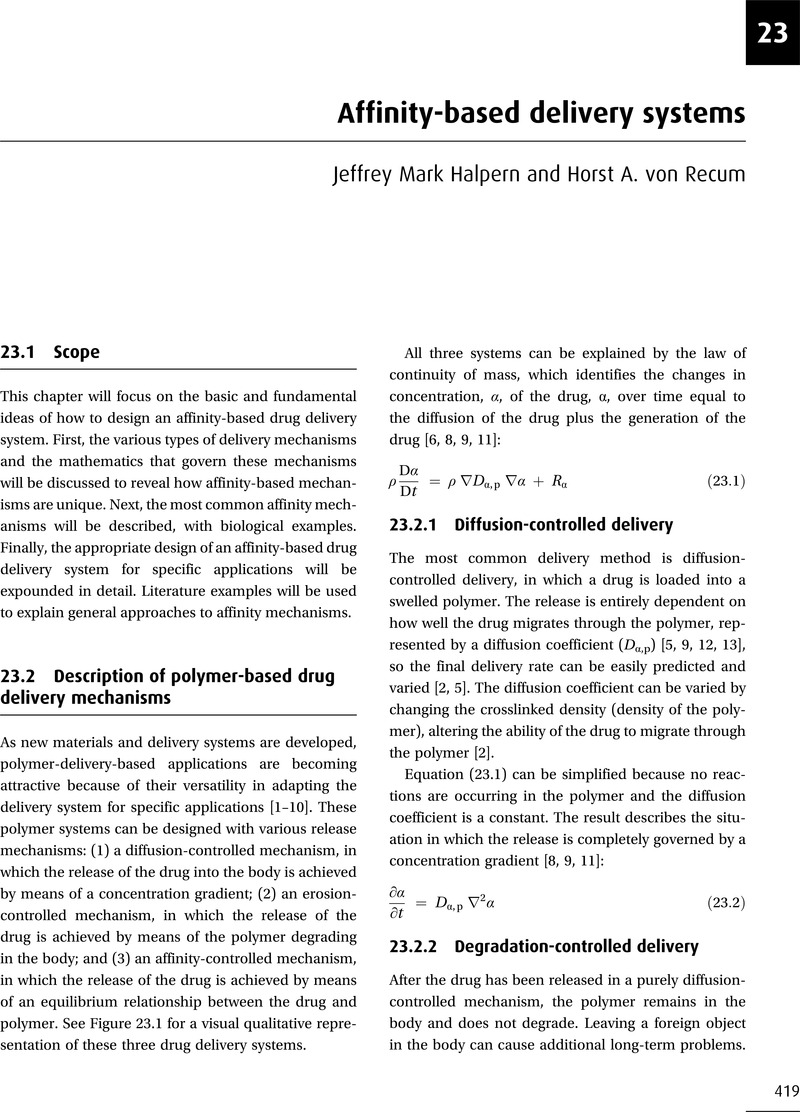
- Type
- Chapter
- Information
- Biomaterials and Regenerative Medicine , pp. 419 - 430Publisher: Cambridge University PressPrint publication year: 2014
References
- 1
- Cited by