Introduction
Severe acute respiratory syndrome coronavirus 2 (SARS-CoV-2) causes coronavirus disease 2019 (COVID-19), an infection which may affect the respiratory tract. The World Health Organization recognised COVID-19 as a pandemic in early 2020 owing to the rapid dissemination of the virus and the high virulence(Reference Cucinotta and Vanelli1).
Most patients affected with COVID-19 are oligosymptomatic, having almost no symptoms or presenting them discreetly. However, damage caused by this virus may intensify due to an inadequate immune response(Reference Böhmer, Buchholz and Corman2). SARS-CoV-2 often causes respiratory tract infection symptoms, but it is not limited to impairing lung function; it can systematically affect the organism, undermining gastrointestinal(Reference Wong, Lui and Sung3), cardiovascular and renal functions(Reference Zheng, Ma and Zhang4), and even the nervous system(Reference Berger5).
Reports suggest that SARS-CoV-2 RNA may be detected in the stool of some COVID-19 patients(Reference Amirian6,Reference Dhar and Mohanty7) , while another study has already reported that there is dysbiosis, meaning there are changes in the diversity and population of beneficial bacteria and that these are associated with the severity of COVID-19(Reference Tang, Gu and Gong8). These findings, associated with the gastrointestinal symptoms of this disease, point to an involvement of the gut–lung axis and an influence of the gut microbiota(Reference Amirian6,Reference Dhar and Mohanty7) .
The ‘gut–lung axis’ refers to mutual interaction or a bidirectional effect between gut microbiota and lungs. Previous studies have shown that viral respiratory infections alter the commensal microbiota in both the gastrointestinal and the airway tracts of the host, probably through the blood, which transports endotoxins and microbial metabolites(Reference Enaud, Prevel and Ciarlo9–Reference Zuo, Zhang and Lui11).
The gut microbiota varies depending on diet, lifestyle, antibiotics, genetic background and nutritional status(Reference Danneskiold-Samsøe, Barros and Santos12). In this sense, obesity, hypertension and diabetes have been identified as risk factors for serious complications of COVID-19, although the underlying pathophysiology surrounding the manifestation of symptoms and clinical outcomes of the disease are not yet clear(Reference Maffetone and Laursen13). There is evidence that dietetic modulation of the gut microbiota can influence viral transmission and disease progression(Reference Walton, Gibson and Hunter14).
Maintaining a healthy diet associated with the consumption of probiotics and prebiotics may be an important nutritional strategy as a prevention or as an adjuvant treatment for COVID-19(Reference Walton, Gibson and Hunter14), even in individuals with inadequate nutritional status and unbalanced gut microbiota(Reference Dhar and Mohanty7,Reference Vernocchi, Del Chierico and Putignani15) . Probiotics and prebiotics play an important role in regulating the immune system through intestinal microbiota modulation, with increased proliferation of micro-organisms beneficial to health and reduced pathogenic micro-organisms, as well as by the systemic actions of the products of microbial metabolism such as short-chain fatty acids (SCFA)(Reference Vernocchi, Del Chierico and Putignani15).
The immunomodulatory effect of probiotics and prebiotics on development and maturation of innate and adaptive immunity occurs through the secretion of cytokines such as interleukin 10 (IL-10) and transforming growth factor-β (TGF-β; control the regulatory T (Treg) cells development and the response of helper T cells (Th1/Th2)) for the control for releasing tumour necrosis factor-α (TNF-α), interferons and chemokines of immune cells. Treg cells are an important subpopulation of T cells responsible for suppressing the inflammation response and maintenance of immune system homeostasis(Reference Azad, Sarker and Wan16–Reference Peters, van de Steeg and van Bilsen19).
Although vaccination is progressing in many countries, studies show that SARS-CoV-2 has mutated, with increased transmissibility and increased severity of COVID-19 cases(Reference Dao, Hoang and Gautret20). Considering that diet and nutritional status have been identified as important variables for the outcome of this infection(Reference Morais, Aquino and da Silva-Maia21), this narrative review analyses the main aspects of using probiotics and prebiotics as a nutritional strategy to improve the immune response against respiratory viral infections, considering prospects for COVID-19. We also considered the relationship between inflammatory responses and the clinical spectrum of COVID-19, ranging from asymptomatic to severe clinical presentations, and the influence of chronic diseases and nutritional status of individuals on disease prognosis.
In this sense, we sought to gather evidence and new insights into the role of consuming probiotic strains and prebiotic components in a single study, considering: the diversity of strains and compounds, doses, and administration time; the potential action mechanisms against COVID-19 related to the gut–lung axis; and the impact of nutritional status, the life cycle of subjects and the pros and cons of consuming probiotics and prebiotics in the progression of COVID-19. To date, no previous work has gathered this information.
Therefore, we conducted searches in research databases (PubMed, Cochrane Library, Scopus and Web of Science) using the keywords (prebiotic OR probiotic OR synbiotic OR dietary fibre OR polysaccharide OR oligosaccharide OR polyphenol OR phenolic OR Bifidobacterium OR Lactobacillus) AND (SARS OR SARS-CoV infection OR MERS OR MERS-CoV infection OR respiratory viral infection OR viral infection OR viral disease OR lung injury OR acute respiratory distress syndrome OR pneumonia OR ventilator-associated pneumonia) AND (immune system OR T cell OR Treg cell OR gut-lung axis OR dysbiosis OR intestinal dysbiosis OR gut dysbiosis OR symptoms OR abdominal pain OR cough OR diarrhoea OR fever OR headache OR nausea OR sore throat OR vomiting). Our search resulted in 260 studies published up to July 2021, and after removing duplicates and assessing eligibility, a total of 69 clinical studies were included in this narrative review.
Nutritional status and its relationship with the gut–lung axis as a risk factor for COVID-19 complications
The immune response among individuals generally varies according to genetic polymorphisms, which regulate the expression of cytokines, cytokine receptors, human leucocyte antigen and adhesion molecules. In addition, factors such as age, sex, stress, nutrient status and history of infections and vaccinations are important contributors to this response(Reference Calder and Kew22–Reference Fakhouri, Peterson and Kothari24).
Individuals with chronic diseases such as obesity, hypertension and diabetes have undermined nutritional status, due to pre-existing chronic inflammation with high levels of inflammatory markers (C-reactive protein (CRP), IL-6 and TNF-α), a high degree of oxidative stress, and endothelial dysfunction caused by an imbalanced production of vasodilator and vasoconstrictor agents. These conditions combined with the COVID-19 pathophysiology overload the immune system and hinder the development of more effective responses(Reference Kwaifa, Bahari and Yong25,Reference Butler and Barrientos26) , in turn increasing the risk of COVID-19 complications such as pneumonia and acute respiratory distress syndrome(Reference Sharma, Agarwal, Gupta and Saxena27,Reference Yang, Yu and Xu28) .
Experimental and clinical observations have suggested that the gut microbiota plays a key role in the pathogenesis of sepsis and acute respiratory distress syndrome(Reference Dickson29), which further highlights the relevance of nutrition in the clinical outcome and remission of the symptoms of this disease. In addition, COVID-19, when combined with poor nutrition, is associated with requiring mechanical ventilation, longer length of stay in intensive care, and increased mortality rate(Reference Sharma, Agarwal, Gupta and Saxena27).
Antibiotic therapy has been used to treat viral respiratory infections, including COVID-19. However, the use of antibiotics also causes dysbiosis(Reference Kolodziejczyk, Zheng and Elinav30), and this condition increases gut permeability, undermines healing and phagocytosis, reduces lymphocyte count (lymphopenia) and increases the production of pro-inflammatory cytokines(Reference Calder, Carr and Gombart31).
There is evidence that the gut–lung axis plays a key role in the pathophysiology of COVID-19(Reference Amirian6,Reference Dhar and Mohanty7) . Bacterial translocation was initially described to explain how a gut injury could lead to clinical manifestations of injuries at a distance in other organs, including in the lungs. The gut has extensive lymphoid tissue, and mesenteric lymph carries cytokines produced in the gut to the thoracic duct and then the lungs(Reference Iyer and Bansal32).
Previous studies have shown that respiratory infections such as influenza are associated with an alteration in the composition of the gut microbiota, which raises the question of whether SARS-CoV-2 could also harm the gut microbiota(Reference Groves, Higham and Moffatt33). SARS-CoV-2 uses angiotensin-converting enzyme 2 (ACE2) to infect alveolar and epithelial cells of the oesophagus and host enterocytes and cause local and/or systemic hyper-inflammation(Reference Lamers, Beumer and van der Vaart34).
A recent study investigated the interaction between gut and SARS-CoV-2 through single-cell RNA sequencing, showing that the genes of the virus receptor binding domains and the modes of binding with the spike protein of this virus are probably constantly changing in the enterocytes(Reference Muus, Luecken and Eraslan35). In addition, the SARS-CoV-2 genome data show specific mutations and genetic diversity according to the variability of individuals in different demographic regions, which could predict better-designed prophylactic measures among populations(Reference Bajaj and Purohit36).
Due to the high degree of viral replication in the gut epithelium, the action of SARS-CoV-2 in the gastrointestinal system causes an increase in the occurrence of nausea, vomiting, diarrhoea and abdominal pain(Reference Lamers, Beumer and van der Vaart34,Reference Henry, de Oliveira and Benoit37) , which is further aggravated by dysbiosis(Reference Levy, Kolodziejczyk and Thaiss38). Other systemic symptoms such as fever, coughing, fatigue, myalgia, dyspnoea, head and throat pain, smell and taste loss, and anxiety generated by fear of the disease and social isolation lead to a marked reduction in appetite, which further impairs the patient’s nutritional status(Reference Lechien, Chiesa-Estomba and de Siati39).
Inadequate nutritional status and high consumption of pro-inflammatory foods commonly present in a Westernised diet (namely a high content of saturated and trans fats, sodium, and sugars, and low in fibres, vitamins and minerals) are related to greater susceptibility to respiratory infections and a worse prognosis(Reference Danneskiold-Samsøe, Barros and Santos12,Reference Morais, Aquino and da Silva-Maia21) . This relationship may be due to the impact of consuming nutritionally inadequate diets on the individual’s nutritional status and gut health, as well as its interaction with the respiratory tract and the immune system(Reference Dhar and Mohanty7,Reference Kolodziejczyk, Zheng and Elinav30) .
On the other hand, adequate nutrition is a strong ally in promoting health, preventing and treating diseases(Reference Rautiainen, Manson and Lichtenstein40). Several food components present in a Mediterranean diet (fibres, polyunsaturated fatty acids and phenolic compounds) have been demonstrated to reduce inflammation and improve immune response against respiratory infections(Reference Messina, Polito and Monda41).
Adherence to healthy eating habits may be considered a strategy to prevent severe cases of viral infections such as COVID-19. In this context, the inclusion of foods with prebiotic and probiotic properties in the diet may become an important tool for preventing mild to moderate COVID-19 cases, as these components can modulate the immune system through interaction with the gut microbiota and act directly or indirectly at the systemic level, including the respiratory(Reference Sanders, Merenstein and Reid42), gastrointestinal(Reference Hill, Guarner and Reid43), cardiovascular(Reference Delgado and Tamashiro44) and nervous system(Reference Ahmadi, Nagpal and Wang45).
Probiotics
Action mechanisms and health benefits of probiotics
Probiotics are living micro-organisms which provide benefits to the host’s health when administered in adequate doses(Reference Hill, Guarner and Reid43). These micro-organisms belong to different genera and species, both bacteria and yeast. Some lactic acid bacteria, in particular the Lactobacillus and Bifidobacterium genera, make up the vast majority of probiotics marketed either in pharmaceutical formulas or in foods, such as fermented milk, yoghurts, cheeses, kefir and kombucha(Reference Gallego and Salminen46).
The probiotics most frequently found in food products are Bifidobacterium (adolescentis, animalis, bifidum, breve and longum) and Lactobacillus (acidophilus, casei, fermentum, gasseri, johnsonii, paracasei, plantarum, rhamnosus and salivarius)(Reference Zielińska and Kolożyn-Krajewska47). Some yeast strains are also believed to be probiotic, such as Saccharomyces boulardii (Reference Kerry, Patra and Gouda48). Despite the different metabolic characteristics of probiotic strains, which have not yet been fully elucidated, the ability to produce SCFA is a feature shared by many different probiotics and certainly plays a significant role in the host’s health(Reference Sanders, Benson and Lebeer49).
SCFA are final products of the fermentation carried out by probiotics, either from endogenous compounds such as mucus and/or from non-digested carbohydrates in the diet, such as prebiotics(Reference Kerry, Patra and Gouda48). SCFA are organic acids which have one to six carbon atoms such as butyric, acetic and propionic acids, which are quantitatively and qualitatively produced depending on the production site in the large intestine, the gut microbiota composition, the substrates supplied by the diet and the presence of other metabolites(Reference Ríos-Covián, Ruas-Madiedo and Margolles50).
These organic acids are energy sources for colonocytes and promote lower pH in the colon, which inhibits formation of high levels of secondary bile acids(Reference Molska and Reguła51,Reference Davison and Wischmeyer52) , increases the availability of calcium and magnesium(Reference Graf, Di Cagno and Fåk53), modulates the intestinal microbiota through selectively stimulating the growth of beneficial bacterial genera, induces proliferation of epithelial cells in the colon to maintain the integrity of the intestinal barrier, and decreases the translocation of inflammatory bacterial lipopolysaccharide(Reference Brosseau, Selle and Palmer54).
SCFA act as anti-inflammatory mediators, thus stimulating the synthesis of anti-inflammatory cytokine IL-10 by macrophages, neutrophils and T cells, in addition to inhibiting the synthesis of inflammatory cytokines such as TNF-α and IL-6(Reference Sanders, Benson and Lebeer49,Reference Rooks and Garrett55) .
The main probiotic actions on the host’s health occur by inhibiting the proliferation of pathogens in the gut via the production of antimicrobial substances, mainly by Bifidobacterium and Lactobacillus genera, with consequent protection of the intestinal barrier, in addition to modulating the immune system through regulation of innate and adaptive immunity proteins(Reference Zeng, Shen and Bo18,Reference Kalantar-Zadeh, Ward and Kalantar-Zadeh56,Reference Qian, Lu and Huang57) . The specific probiotics can also act at the systemic level, in the central(Reference Wang, Lee and Braun58), cardiovascular(Reference Hsu, Lin and Hou59), endocrine(Reference Farzi, Fröhlich and Holzer60) and respiratory systems(Reference Zhang, Yeh and Jin61).
In this context, the consumption of probiotics has a potential preventive effect on COVID-19 complications, as they contribute to maintaining a healthy microbiota and reinforcing the intestinal barrier, increasing intestinal motility, and reducing pro-inflammatory states(Reference Kalantar-Zadeh, Ward and Kalantar-Zadeh56,Reference Harper, Vijayakumar and Ouwehand62) . Moreover, probiotic activity in different systems and axes of the organism which interact with the gut microbiota may be an effective strategy to alleviate the harmful effects of COVID-19.
The role of probiotics in the immune system and respiratory infections
Probiotics have been studied extensively in recent years and related to as an auxiliary tool in modulating humoral, cellular and non-specific immunity. The relationship between probiotics and the immune system is complex. Accordingly, it becomes even more important to understand the relationship between the consumption of probiotics, the modulation of immunity and its role in the respiratory system amid the COVID-19 pandemic.
To date, few studies have been reported on the use of probiotics as an adjuvant in treating COVID-19(Reference d’Ettorre, Ceccarelli and Marazzato63–Reference Li, Cheng and Xu65). However, scientific evidence points to the immunomodulatory effect of probiotics in resolving viral infections which can be used as a model for COVID-19 cases (Fig. 1).
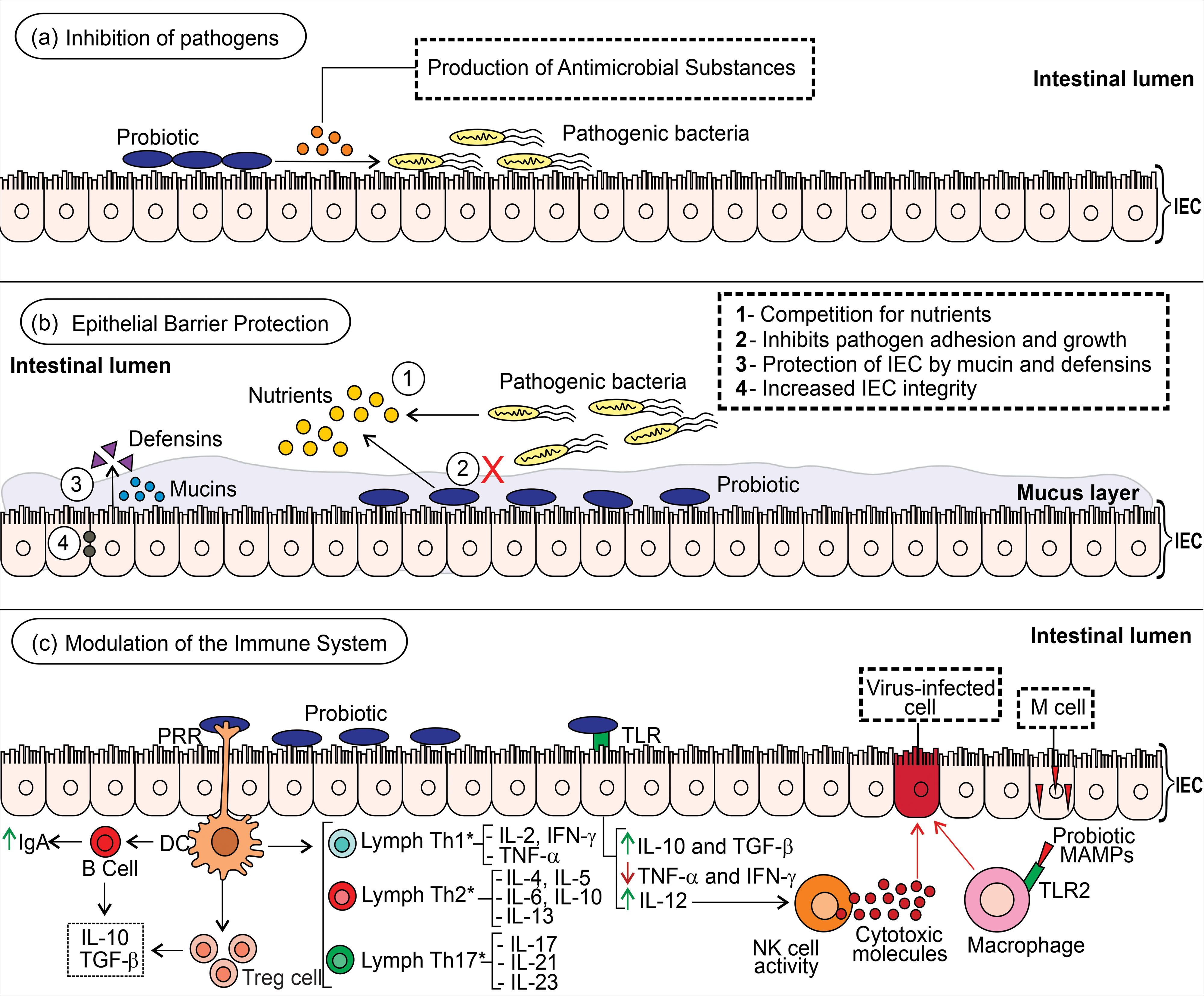
Fig. 1. Potential mechanisms of probiotics on the intestinal immune system. (a) Probiotics secrete antimicrobial substances (i.e. bacteriocin and defensins) capable of inhibiting the action of pathogens in the intestinal epithelium. (b) These beneficial micro-organisms compete with pathogenic bacteria for the use of nutrients and for adhesion sites, which inhibits their growth and insertion in the intestinal epithelium. Probiotics can stimulate the synthesis and release of antimicrobial substances (i.e. defensins) by intestinal epithelial cells (IEC) as well as promote the expression and secretion of mucins which maintain the integrity of the IEC. (c) Probiotics bind with pathogen recognition receptors (PRR, i.e. toll-like receptor (TLR)) in dendritic cells, which stimulates B cells to produce immunomodulatory factors such as IgA, IL-10 and TGF-β; promotes differentiation of T cells in the respective T helper (Th) cells; and stimulates the activity of T-regulatory (Treg) cells which secrete anti-inflammatory IL-10 and TGF-β, regulating the immune system and controlling inflammation(Reference Azad, Sarker and Wan16,Reference Yousefi, Eslami and Ghasemian67,Reference Campbell and Rudensky170,Reference Sharabi, Tsokos and Ding171) . The interaction of probiotics with IEC inhibits TNF-α and IFN-γ production, stimulates IL-10 and IL-12 production, and controls TGF-β function(Reference Zeng, Shen and Bo18,Reference Peters, van de Steeg and van Bilsen19) . The activation of NK cells by IL-12 activates the release of cytotoxic molecules capable of eliminating cells infected by viruses. Moreover, probiotic substances (i.e. microbe-associated molecular patterns (MAMPs)) cross the intestinal barrier mediated by M cells and promote phagocytic activity against virus-infected cells when recognised by innate receptors (i.e. TLR macrophage). It is worth highlighting that the effects differ among the probiotic strains. *The predominant or suppressed Th profile varies among probiotic strains.
Probiotics regulate systemic immune responses through antigen-presenting cells (i.e. macrophages and dendritic cells) present and activated in the mucosa. Mature antigen-presenting cells are activated by antigens (i.e. bacteria) and migrate to the mesenteric lymph node to differentiate lymphocytes with naive cluster of differentiation 4 (CD4) + Th0 into Treg or Th cells, but this will depend on the cytokine secretion pattern(Reference Fong, Shah and Kirjavainen66). Furthermore, the probiotic immunoregulatory and/or immunostimulatory effects are dependent on microbial strain, dose, administration time and nutritional status of the host(Reference Azad, Sarker and Wan16,Reference Peters, van de Steeg and van Bilsen19,Reference Yousefi, Eslami and Ghasemian67) .
Gut dysbiosis causes an innate response of the immune system through an increase in the expression of IL-15 and IL-12 cytokines and recruits natural killer (NK) cells responsible for the apoptosis of infected cells. However, the inflammatory immune response to COVID-19 cases is characterised by dysfunctional NK cells and increased macrophage activity which causes tissue damage. Microbe-associated molecular patterns derived from probiotic strains can be recognised by TLR2 present in macrophages and regulate their phagocytic activity to eliminate the pathogen(Reference Azad, Sarker and Wan16,Reference Merad and Martin68,Reference Market, Angka and Martel69) .
The cell wall lipoproteins and lipoteichoic acid are microbe-associated molecular patterns of Bifidobacterium and Lactobacillus species which can modulate the immune system through binding with TLR2/TLR6 of the host. Lipoteichoic acid stimulates nitric oxide synthase action on the death of the pathogen-infected cell(Reference Azad, Sarker and Wan16,Reference Jiang, Yang and Jin70,Reference Lee, van Swam and Boeren71) . The peptidoglycan hydrolase TgaA of Bifidobacterium spp. induces the production of IL-2 in the dendritic cell, which is the key cytokine in the development of Treg cells(Reference Zeng, Shen and Bo18,Reference Ye, Brand and Zheng72) .
Probiotics strains can produce some metabolites with antiviral activity, such as acetic acid, lactic acid, γ-aminobutyric acid, plantaricin, bacteriocins (i.e. labyrinthopeptin A1, enterocin AAR-71 and erwiniocin NA4) and exopolysaccharides(Reference Tiwari, Dicks and Popov73,Reference Anwar, Altayb and Al-Abbasi74) . Exopolysaccharides from Lactobacillus plantarum strain N4 (Lp) showed an inhibition effect on transmissible gastroenteritis coronavirus in vitro (Reference Yang, Song and Wang75). A computational study demonstrated that plantaricin W’s anti-SARS-CoV-2 activity may be due to its stable binding to the ACE2 receptor of human, RNA-dependent RNA polymerase and residual binding domain of spike protein of SARS-CoV-2(Reference Anwar, Altayb and Al-Abbasi74).
Human intestinal defensin 5 (HD5) is an α-defensin secreted by Paneth cells from the small intestine crypts, which has antimicrobial action. An in vitro study by Wang and colleagues(Reference Wang, Wang and Li76) pointed out that HD5 cloaked several sites in the ligand-binding domain of ACE2 on enterocytes, mainly Asp30 and Lys31 residues on α-helix 1, which is essential for binding the SARS-CoV spike. They hypothesised that oral supplementation of specific probiotic strains would increase the number of Paneth cells and HD5 secretion to reduce infection of intestinal cells by SARS-CoV-2 and possibly maintain the balance of the local and systemic immune responses. However, studies must be conducted to investigate this hypothesis.
Studies with human and animal models which report effects from the use of probiotics on the immune system, viral infections, and risk factors for serious complications in COVID-19 are detailed in Supplementary Table S1. A study with female BALB/c mice infected with influenza A virus showed that the use of L. paracasei CNCM I-1518 at a dose of 2 × 108 colony-forming units (CFU)/d, for 7 d before and 10 d after viral infection, was able to modulate pulmonary immunity associated with better control of this infection, thereby enabling early activation of pro-inflammatory cytokines (IL-1α and IL-1β) and massive recruitment of immune cells into the lungs before influenza infection. The immune system pre-activation may induce faster clearance of the influenza virus(Reference Belkacem, Serafini and Wheeler77).
A study of female BABL/c mice (6–8 weeks old) with influenza (H1N1 and H3N2) treated with Lactobacillus plantarum strain DK119 at a dose of 108–109 CFU/d, for 10 d before and 14 d after viral infection, is noteworthy, showing that Lactobacillus plantarum DK119 strain had a preventive and protective effect against infection by influenza viruses, probably increasing the innate immunity of dendritic cells and CD11c+ macrophages and antiviral cytokines (i.e. interferon-γ (IFN-γ) and IL-12), thus contributing to better control of pulmonary viral loads(Reference Park, Ngo and Kwon78).
A study involving 190 healthy adults showed that the consumption of yoghurts with Lactobacillus gasseri SBT2055 strain probiotics at a dose of 100 g/d, consumed for 16 weeks, could activate innate and adaptive human immune responses (natural killer cell activity, myxovirus resistance A gene expression, IgG and IgA levels) after trivalent influenza vaccination, indicating the possible potential to prevent infections by influenza virus(Reference Nishihira, Moriya and Sakai79).
A meta-analysis has shown the relationship between the use of administered probiotics with doses ranging from 1 × 108 to 2 × 1010 CFU and a reduction in the severity of symptoms in cases of respiratory tract infection in children aged 3 months to 7 years and adolescents aged 7–18 years. This protocol also brought a decrease in the infection duration, mainly found in the formula containing Lactobacillus rhamnosus GG strain(Reference Laursen and Hojsak80,Reference Wang, Li and Ge81) . Although COVID-19 cases are more prevalent in adults and older adults, children and adolescents are also susceptible to infection and death from SARS-CoV-2 and can transmit the virus(Reference Dong, Mo and Hu82–Reference Lee and Raszka84). The results of the meta-analysis(Reference Laursen and Hojsak80,Reference Wang, Li and Ge81) indicate that a study protocol for the use of the Lactobacillus rhamnosus GG strain could be expanded to adult and juvenile populations for the prevention of COVID-19.
A pilot randomised clinical trial (RCT)(Reference Wang, Lin and Xiang85) carried out in hospitals in Wuhan showed that oropharyngeal probiotic Streptococcus thermophilus ENT-K12 at a dose of 1 × 109 CFU administered daily for 1 month in ninety-eight healthy frontline medical staff (20–65 years of age) reduced the incidence of respiratory tract infections and time experiencing respiratory tract infections symptoms (sore and/or itchy throat, cough, oral ulcer and fever), eliminated the need for antibiotics or antivirals drugs, and shortened the days absent from work. However, this RCT has major limiting factors, including not being a blinded study, small number of participants, and few days of treatment, which were not sufficient to detect the preventive effect of infections by COVID-19, reducing its quality of evidence(Reference Wang, Lin and Xiang85).
Two retrospective cohort studies by d’Ettorre et al.(Reference d’Ettorre, Ceccarelli and Marazzato63) and Ceccarelli et al.(Reference Ceccarelli, Borrazzo and Pinacchio64) reported the concomitant use of oral probiotic therapy (Bifidobacterium lactis DSM 32246 and DSM 32247 strains, Lactobacillus acidophilus DSM 32241, L. helveticus DSM 32242, L. paracasei DSM 32243, L. plantarum DSM 32244, L. brevis DSM 27961, Streptococcus thermophilus DSM 32345) at a dose of 2·4 × 1010 CFU/d and drug therapy (hydroxychloroquine, azithromycin, lopinavir-ritonavir or darunavir-cobicistat, and/or tocilizumab) for ±14 d in 28 and 112 Italian adults, respectively, with severe COVID-19 pneumonia to modulate the gut–lung axis. Patients treated with oral probiotic therapy showed remission of diarrhoea, fever, asthenia, headache, myalgia and dyspnoea; and no deaths or use of invasive mechanical ventilation were reported(Reference d’Ettorre, Ceccarelli and Marazzato63). Oral probiotic therapy increased the production of the nuclear factor erythroid 2p45-related factor 2 (Nrf2) and haem oxygenase-1 (HO-1), molecules with recognised antiviral activity(Reference d’Ettorre, Ceccarelli and Marazzato63). Furthermore, Ceccarelli et al.(Reference Ceccarelli, Borrazzo and Pinacchio64) showed that oral bacteriotherapy is an independent variable associated with a reduced risk for death in patients hospitalised with COVID-19.
A retrospective study evaluated the administration of probiotics in 311 patients with severe COVID-19 hospitalised at Wuhan Union Hospital(Reference Li, Cheng and Xu65). In addition to drug treatment (chloroquine phosphate, Arbidol and ribavirin interferon α inhalation or lopinavir/ritonavir), 123 patients received a combined dose of probiotics for an average time of 12·94 d. Probiotics were administered in a combined oral dose with tablets containing Bifidobacterium infantis, Lactobacillus acidophilus, Dung enterococcus and Bacillus cereus, 1·5 g tid; live combined Bifidobacterium longum, Lactobacillus bulgaricus and Streptococcus thermophiles tablets, 2 g tid; and live combined Enterococcus faecium and Bacillus subtilis capsules, 0·5 g tid. The use of probiotics could be an effective strategy in the treatment of COVID-19 patients to reduce the secondary infection and to moderate immunity(Reference Li, Cheng and Xu65).
The previously described trials(Reference d’Ettorre, Ceccarelli and Marazzato63–Reference Li, Cheng and Xu65) are observational, which limits extrapolating the outcomes to a larger population. Limitations include that these studies are non-blind, single-centre and not prospective, have a small number of participants, and did not randomise participants. However, the studies provide preliminary evidence that may guide future randomised controlled trials on prevention and/or treatment of COVID-19.
Probiotic treatment has been a promising field of research in the health sciences since specific probiotics (alone or combined with prebiotics) have the potential to modulate gut microbiota and immune responses in the host organism. It is seen that several probiotics strains, with emphasis on the genera Lactobacillus and Bifidobacterium, have a positive influence on innate immunity, exerting several antiviral properties, as well as on the protection of the respiratory system through controlling cytokine production and recruiting defence cells. As the lungs constitute the main action site of COVID-19, the use of specific probiotics becomes a promising tool in supporting the defence mechanisms in these organs.
Prebiotics
Action mechanisms and health benefits of prebiotics
The interest in foods and supplements with prebiotic properties has grown over the years, and its concept has undergone changes considering the scientific and clinical updates. Prebiotics are defined as a substrate which is selectively utilised by host micro-organisms that confer a health benefit. These compounds must not be degraded by the target host enzymes, and so they are fermented by the colon bacteria(Reference Gibson, Hutkins and Sanders86).
Most prebiotics are synthesised or isolated from plant polysaccharides and are oligosaccharides, such as: fructo-oligosaccharides (FOS), found in beetroot, asparagus, garlic, onions, chicory, wheat and banana; galacto-oligosaccharides (GOS), found in human milk and cow milk; isomalto-oligosaccharides, found in sugar cane and honey; xylo-oligosaccharides, found in fruits, vegetables, wheat bran and honey(Reference Ashwinia, Ramya and Ramkumara87); inulin, found in wheat, tomatoes, garlic, barley and chicory roots; and resistant starch, found in raw potatoes, green bananas and grains. In addition to being natural sources, processed foods are often supplemented with prebiotics, such as dairy products, beverages, baby formulas, meats and bakery products(Reference Neri-Numa, Arruda and Geraldi88).
Although prebiotics and probiotics have common action mechanisms, especially regarding the modulation of the gut microbiota, they differ in their composition and metabolism. Depending on the structure and composition, prebiotics can be used by specific bacteria as a source of carbon and energy(Reference Singh, Chang and Yan89). Prebiotics may have direct effects on gut epithelial cells and immune cells or indirect effect on the host’s health, serving as substrates for probiotics that promote immunomodulation, as they inhibit the growth of pathogenic bacteria and also improve digestion and absorption of essential nutrients(Reference Ahmadi, Nagpal and Wang45,Reference Markowiak and Śliżewska90) .
Prebiotics intake is associated with benefits at the systemic level in the body, mediated by SCFA such as through regulation of various pathophysiological (i.e. inflammation) and metabolic processes (i.e. lipid and glucose metabolism), thus contributing to prevention or treatment of chronic diseases(Reference Xavier-Santos, Bedani and Lima91). Regular intake of prebiotics is also associated with beneficial effects on the renal(Reference McFarlane, Ramos and Johnson92), cardiovascular(Reference Delzenne, Olivares and Neyrinck93), nervous(Reference Paiva, Duarte-Silva and Peixoto94) and respiratory(Reference Groves, Higham and Moffatt33) systems.
Other non-carbohydrate substances such as phenolic compounds have also been accepted as potential prebiotics(Reference Gibson, Hutkins and Sanders86). Phenolics are secondary metabolites of plants and are present in various foods such as fruits, vegetables, teas, coffee, wines and chocolates(Reference Amiot, Riva and Vinet95). Phenolic compounds may also benefit the gut microbiota, exerting similar effects to prebiotics already well established in the pertinent literature(Reference Moorthy, Chaiyakunapruk and Jacob96). These compounds seem to interact with transcription factors, including nuclear factor κ-light-chain-enhancer of activated B cells (NF-kB) and Nrf2, exerting anti-inflammatory and antioxidant effects and immunomodulatory properties(Reference Delgado and Tamashiro44).
The role of prebiotics in the immune system and respiratory infections
There is evidence to support the effectiveness of using prebiotics in viral diseases, including respiratory diseases, although most studies have been conducted with individuals in their infancy(Reference Luoto, Ruuskanen and Waris97–Reference Ranucci, Buccigrossi and Borgia99). In this sense, associations between the effects of prebiotic consumption on the gut microbiota and immunity related to respiratory infections, especially on COVID-19 symptoms, constitute a challenge to be investigated(Reference Kalantar-Zadeh, Ward and Kalantar-Zadeh56).
Prebiotics produce beneficial alterations in the immune system and the host’s health through direct and indirect mechanisms(Reference Ranucci, Buccigrossi and Borgia99) (Fig. 2). Some non-clinical and clinical studies about the effects of prebiotics on the immune system, viral infections, and risk factors for serious complications in COVID-19 are detailed in Supplementary Table S2.
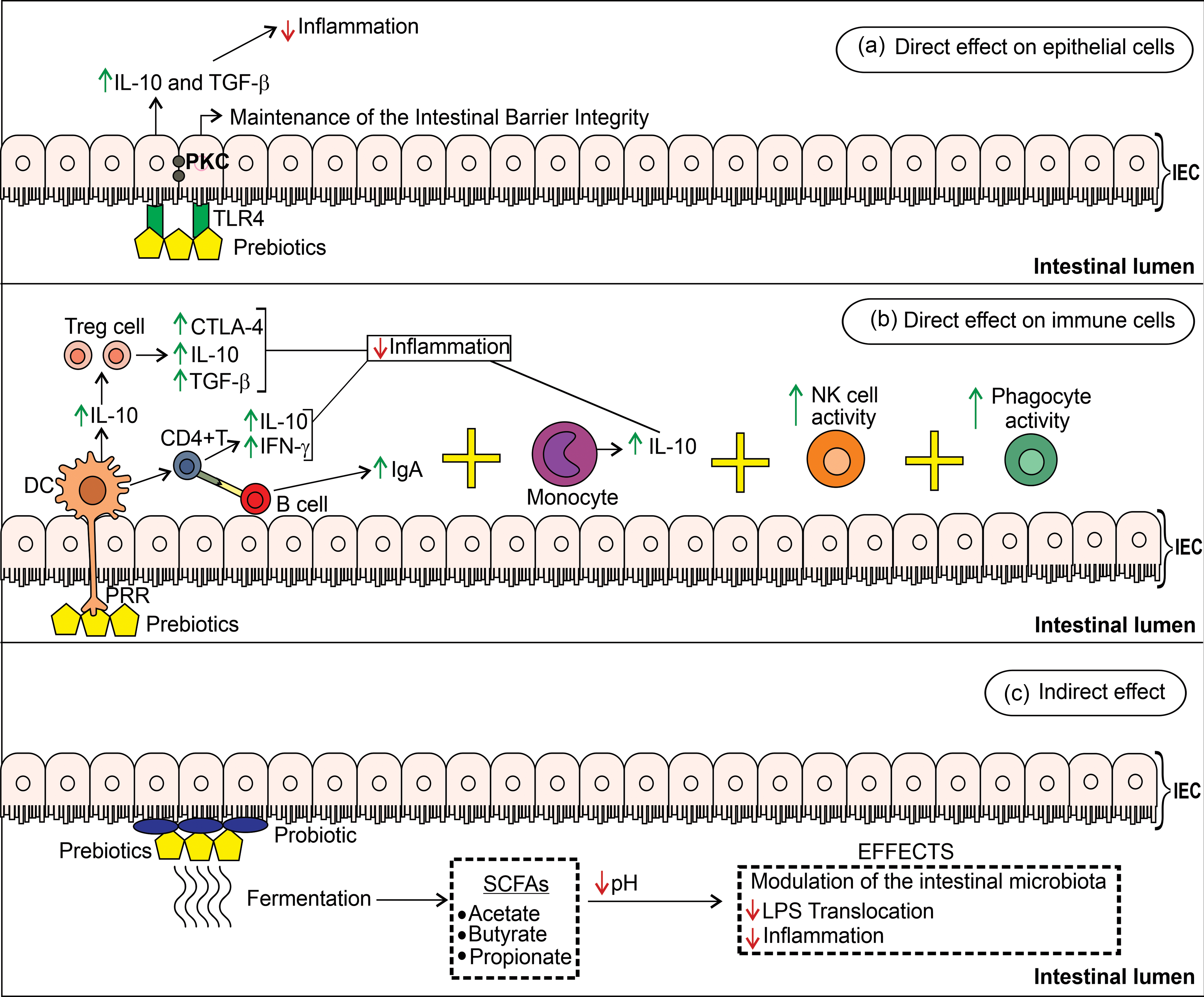
Fig. 2. Potential mechanisms and effects of prebiotics on the intestinal immune system. (a) Prebiotics act directly on IEC by binding to their TLR4 and activate protein kinase C (PKC) to maintain the integrity of the intestinal barrier and regulate intestinal inflammation through synthesising inhibitory cytokines IL-10 and TGF-β. (b) The direct action of prebiotics on immune cells regulates the inflammatory response to the pathogen. Prebiotics bind to pathogen recognition receptors (PRR) on the surfaces of dendritic cells which secrete IL-10 to stimulate Treg cells to express cytotoxic T-lymphocyte-associated protein 4 (CTLA-4) receptors, IL-10, and TGF-β. Dendritic cells activate CD4+ T cells to release IL-10 and IFN-γ (Reference Sanders, Merenstein and Reid42,Reference Brosseau, Selle and Palmer54) . IFN-γ production by CD4+T cells helped in clearing influenza and dengue virus(Reference Tian, Seumois and de-Oliveira-Pinto172,Reference Shinde, Hansbro and Sohal173) . CD4+ T cells interact with B cells and stimulate IgA production. Prebiotics can also bind to the TLR4 receptor for monocytes which secrete IL-10, and increase the activity of NK cells (involved in defence against viruses and tumour cells) and phagocytes (i.e. macrophages) to combat the offending agent. (c) Prebiotics reduce inflammation by indirect mechanisms when fermented by probiotic intestinal bacteria which produce short-chain fatty acids (SCFA)(Reference Corrêa-Oliveira, Fachi and Vieira174).
A case–control study investigated the association between dietary patterns and COVID-19 in 2884 front-line healthcare workers from six countries (France, Germany, Italy, Spain, UK, United States) who were screened based on substantial exposure to patients with COVID-19(Reference Kim, Rebholz and Hegde100). Individuals who reported consuming plant-based diets or pescatarian diets, including prebiotics foods, were associated with lower odds of moderate-to-severe COVID-19. Despite the large sample size, diverse health professionals from different countries, and careful adjustment of potential confounding factors, this study relied on a self-report population predominantly composed of male physicians, without the inclusion of individuals affected by the most severe cases of COVID-19. This evidence points to the need to replicate the study both in women and in non-health professionals, with detailed data on the consumption of macro- and micronutrients to elucidate the associations between plant-based and pescatarian diets with the severity of COVID-19(Reference Kim, Rebholz and Hegde100).
Studies on the administration of prebiotics in animal models with viral infections are scarce, since most publications address the prevention and treatment of rotavirus infection in childhood(Reference Azagra-Boronat, Massot-Cladera and Knipping101–Reference Rigo-Adrover, Knipping and Garssen103), with a few studies investigating the effect of prebiotics on other types of viral infections in adult rodents.
One study showed that inulin at a dose of 12 g for 2–4 weeks of treatment significantly increased the population of Bifidobacterium and Lactobacillus, which increased the SFCA production, thus modulating the gut microbiota(Reference Vandeputte, Falony and Vieira-Silva104). Prebiotics, such as wheat bran, GOS and FOS may raise butyrate levels, which in turn reduce inflammation and improve conditions in asthmatic patients(Reference Anand and Mande105). Butyrate alone cannot explain the effects of prebiotics on the gut immune system; propionate and acetate probably also play key roles in the regulation of expression of immune system genes(Reference Gourbeyre, Denery and Bodinier106).
Pectin and 1-kestose may induce the proliferation of Faecalibacterium prausnitzii, known for its anti-inflammatory effects(Reference Tochio, Kadota and Tanaka107), and pectin also increased the proliferation of Eubacterium eligens DSM 3376 in vitro, which increased the secretion of anti-inflammatory IL-10(Reference Chung, Meijerink and Zeuner108). GOS and FOS may cause IL-10 secretion in blood monocyte-derived dendritic cells stimulated by TLR4 binding(Reference Lehmann, Hiller and van Bergenhenegouwen109).
Although it is clear that prebiotics have effects on the microbiota, such as modification, stimulation and antipathogenic effect, little is known about the specific action of each type of prebiotic in the various genera and species that make up the gut microbiota(Reference Gourbeyre, Denery and Bodinier106). This point can consequently be a limiting factor in prospecting the use of prebiotics in prevention and adjuvant treatment and in the period of COVID-19 remission. In this context, the concomitant use of prebiotics and probiotics in synbiotic formulation could provide more benefit in COVID-19 owing to synergic effects between components.
Emerging prebiotics, such as phenolic compounds, can act as antioxidants and direct enzyme inhibitors and may block virus–cell interaction(Reference Chojnacka, Witek-Krowiak and Skrzypczak110). Luteoxanthin and violaxanthin from Urtica dioica (Reference Upreti, Prusty and Pandey111), rutin(Reference García-Iriepa, Hognon and Francés-Monerris112) and neochlorogenic acid from Lianhuaqingwen (an herb product of traditional Chinese medicine)(Reference Chen, Wu and Chen113) can be potent ligands/inhibitors of ACE2 to prevent SARS-CoV-2/ACE2 binding. However, this ACE2 inhibitory function by phenolic compounds needs to be confirmed in humans with COVID-19, as well as whether the synergic interaction of these compounds would bring greater benefits in the treatment of this disease.
Another important point to investigate is whether synbiotic formulations of these phenolic compounds with oligosaccharides, as well as Bifidobacterium and Lactobacillus strains, could protect against infection by SARS-CoV-2 while controlling the inflammatory response. Various technologies (omics and bioinformatics) can be used to check the effectiveness and safety of new natural products with antiviral action.
Pros and cons of prebiotics and probiotics in the prevention and treatment of COVID-19
The cytokine storm which occurs in patients infected with COVID-19 contributes to worsening of symptoms and development of acute respiratory distress syndrome, pneumonia, sepsis and multiple organ dysfunction(Reference Robba, Battaglini and Pelosi114–Reference Chen and John Wherry117). Drug therapies have been tested to control the immune system; however, many medications have serious side effects which can cause dysbiosis in the gut–lung axis and worsen COVID-19 symptoms(Reference Alhazzani, Møller and Arabi118).
At this delicate moment in which the number of cases and deaths is still increasing in many countries despite beginning vaccination, researchers are looking for foods or bioactive compounds with potential functional properties and micro-organisms that offer prevention and benefits in the adjuvant treatment of COVID-19. To date, there are a few clinical studies which have evaluated the impact of consuming probiotics and prebiotics in people affected by COVID-19(Reference d’Ettorre, Ceccarelli and Marazzato63–Reference Li, Cheng and Xu65,Reference Ahmadi, Salari and Sharifi119–Reference Saber-Moghaddam, Salari and Hejazi121) . However, there are no evaluations on introducing prebiotics and probiotics in the remission period of this disease. Some RCTs regarding probiotic and/or prebiotic use in COVID-19 are in progress and are registered in the ClinicalTrials.gov and International Clinical Trials Registry Platform (ICTRP) databases (ACTRN12620000480987; IRCT20101020004976N6; IRCT20200923048815N1; NCT04366089; NCT04366180; NCT04390477; NCT04399252; NCT04420676; NCT04507867; NCT04521322; NCT04621071; NCT04666116; NCT04734886; NCT04756466; NCT04793997; NCT04798677; NCT04813718; NCT04847349; NCT04877704; NCT04884776; NCT04937556; NCT04941703; and NCT04950803), or have been finalised but have not yet published their results (NCT04458519, NCT04517422 and NCT04854941).
Thus, considering the levels of scientific evidence, we sought RCTs and RCT meta-analyses which support the preventive and therapeutic potential of prebiotics, probiotics and their associations in signs and symptoms of viral infections. Through indirect evidence, we highlight studies on the effect of prebiotics, probiotics and synbiotics consumption on the incidence, duration and clinical outcomes related to viral infections, which can be promising in the pathophysiology of COVID-19 (Table 1). Only two RCT have been conducted to date, demonstrating direct evidence of the use of curcumin, a polyphenol which is considered an emerging prebiotic, in the treatment of COVID-19(Reference Ahmadi, Salari and Sharifi119,Reference Pawar, Mastud and Pawar120) . Oral administration of curcumin (80–525 mg twice a day) for 2 weeks substantially reduced morbidity and mortality and improved recovery time in patients with mild, moderate and severe symptoms of COVID-19 (Table 1). The promising effects of this polyphenol were evaluated in a prospective open-label non-RCT which administrated nanocurcumin in soft gel (80 mg twice a day) for 2 weeks in patients (n = 21) aged 18–75 years with mild to moderate symptoms of COVID-19(Reference Saber-Moghaddam, Salari and Hejazi121). The main effects found were resolution of fever, cough, tachypnoea, chill and myalgia; increased lymphocyte count and oxygen saturation; and shorter length of supplemental oxygen use and hospitalisation(Reference Saber-Moghaddam, Salari and Hejazi121).
Table 1. Effects of the consumption of prebiotics, probiotics and synbiotics on the incidence, duration and symptoms related to viral infections
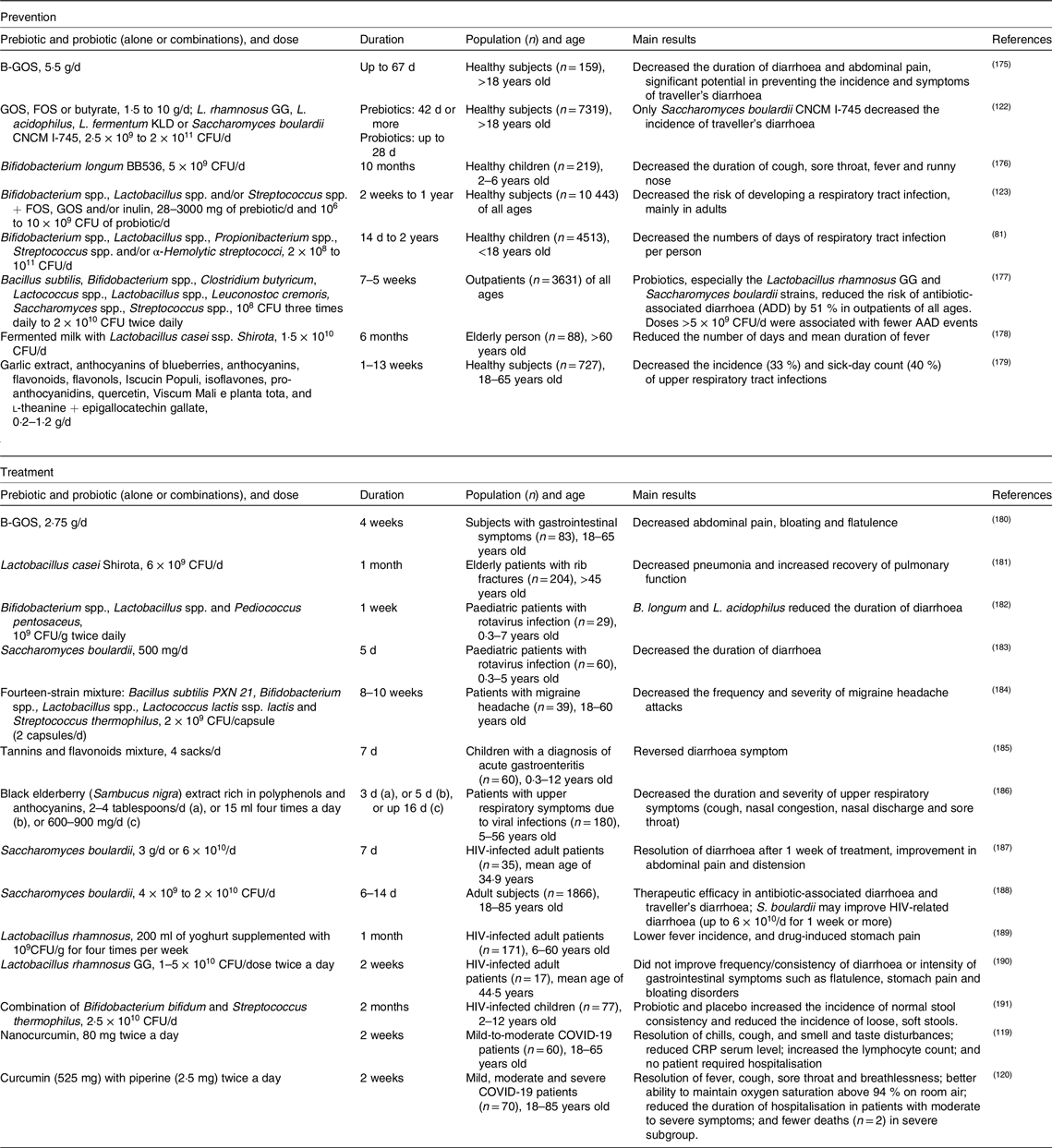
B., Bifidobacterium; B-GOS, Bimuno-galactooligosaccharides; CFU, colony-forming units; FOS, fructo-oligosaccharides; GOS, galacto-oligosaccharides; HIV, human immunodeficiency virus; L., Lactobacillus; spp., species; ssp., subspecies.
Clinical studies generally have different forms (i.e. powder, capsules, tablets, soft gels or foods), doses (0·20–10 g/d) and durations of prebiotics administration (3 d to 43 weeks); as well as probiotics alone or mixed, with varying doses (106–2 × 1011 CFU/d) and administration time (5 d to 104 weeks) (Table 1). A large variation was similarly observed in the synbiotic administration of prebiotics (0·03–3 g/d) and probiotics (106–10 × 109 CFU/d) dose, as well as in the administration time (2–52 weeks).
We also noted that most studies have an experimental design with supplementation of prebiotics, probiotics or synbiotics, possibly due to the ability to administer doses that are better defined compared with the consumption of foods containing these components (Table 1). Some studies cited mild adverse events in healthy children and adults consuming prebiotics and probiotics, such as loose stools, abdominal pain, diarrhoea, constipation, vomiting, poor appetite, hives, rash and dry skin(Reference Wang, Li and Ge81,Reference McFarland and Goh122,Reference Chan, Tao and Chan123) .
Despite variation in methodology and evidence, the studies previously cited in conjunction with data from some guidelines(124–Reference Hojsak, Fabiano and Pop126) point to beneficial effects of the consumption of prebiotics, probiotics and synbiotics, which may contribute to the prevention and treatment of some symptoms of COVID-19 and other viral infections (Fig. 3), provided they are associated with a healthy diet.
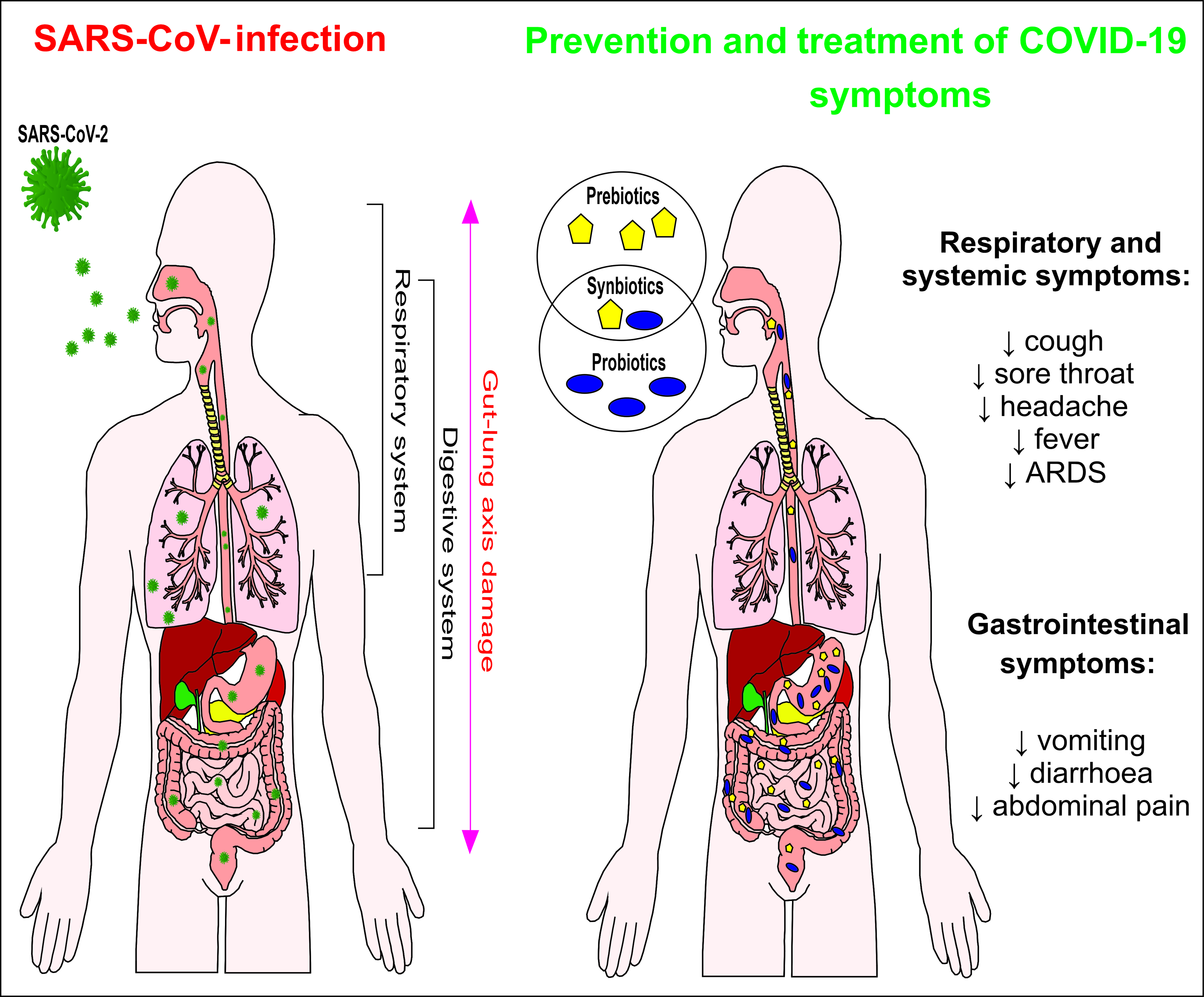
Fig. 3. Potential effects of the consumption of prebiotics (), probiotics (
) or synbiotics (
) against COVID-19 symptoms. ARDS, acute respiratory distress syndrome; SARS-CoV-2, severe acute respiratory syndrome coronavirus 2 (
).
Furthermore, probiotics use (Lactobacillus plantarum alone or in combination with other probiotics and Lactobacillus rhamnosus GG strain in combination with other probiotics) at an average dose of 5 × 109 CFU/d for 3–28 d has been associated with a reduction in infection complications such as ventilator-associated pneumonia in critically ill adult patients(Reference Manzanares, Lemieux and Langlois127).
Probiotics (Lactobacillus spp., Bifidobacterium spp., Enterococcus faecealis, Clostridium butyricum, Bacillus mesentericus, Streptococcus faecalis, Pediacoccus pentosaceus and/or Leuconostoc mesenteroides) and synbiotics (different probiotic strains + GOS, FOS, β-glucan, inulin, pectin, resistant starch, oat fibre, oligofructose and/or malto-oligosaccharide) in a dosage range of 107–1011 CFU/d for 3–28 d reduced the duration of antibiotic therapy and the incidence of pneumonia, sepsis, abdominal distention, diarrhoea and other surgery-related complications(Reference Skonieczna-Żydecka, Kaczmarczyk and Łoniewski128). These beneficial effects were associated with the reduction in plasma levels of inflammatory markers such as CRP and IL-6, as well as an increase in blood concentration of acetic, butyric and propionic acids. However, the limited amount of data from the studies included in this meta-analysis(Reference Skonieczna-Żydecka, Kaczmarczyk and Łoniewski128) made it difficult to determine the most suitable probiotic strains and synbiotics profile to avoid complications related to surgery, as well as the best intervention time and ideal supplement dose.
Specific prebiotic and probiotic intake can minimise gastrointestinal symptoms of COVID-19 and the effects of using antibiotics which worsen these symptoms(Reference Lin, Jiang and Zhang129,Reference Agamennone, Krul and Rijkers130) and reconstitute the gut microbiome, along with consequent modulation of the immune system, a decrease in vulnerability to infections and increased number of resistance genes(Reference Peters, van de Steeg and van Bilsen19,Reference Guo, Goldenberg and Humphrey131) . Moreover, the inclusion of prebiotics and probiotics in the diet can be a potential therapeutic intervention against persistent health damage derived from antibiotics use such as the development of asthma and other respiratory diseases(Reference Becattini, Taur and Pamer132).
Some meta-analyses show the potential of prebiotics (including emerging ones, such as phenolic compounds), probiotics and synbiotics to modulate the immune system of healthy and sick people: AIDS, respiratory and skin allergies, chronic obstructive pulmonary disease, asthma, and other diseases. Prebiotics reduce CRP(Reference McLoughlin, Berthon and Jensen133–Reference Zheng, Guo and Wang135); probiotics also reduce CRP as well as TNF-α and IL-1β levels, and increase IL-10 levels, leucocyte count, NK cell count and activity, and T-cell and monocyte percentages(Reference Zheng, Guo and Wang135–Reference Kazemi, Soltani and Ghorabi139); synbiotics reduce CRP and TNF-α levels and increase leucocyte, NK-cell, T-cell and monocyte counts(Reference McLoughlin, Berthon and Jensen133,Reference Zheng, Guo and Wang135,Reference Kazemi, Soltani and Ghorabi139) . After a month of synbiotic mixture supplementation, there was an increase in CD4 count in HIV-infected women(Reference Kazemi, Djafarian and Speakman140); however, Fu et al.(Reference Fu, Chu and Ashuro141) found no significant difference in CD4 counts in groups of patients treated with probiotics, prebiotics, synbiotics or placebo. It is not yet clear whether probiotic use in HIV-infected subjects can improve the systemic immune response(Reference Ceccarelli, Borrazzo and Pinacchio64).
Oesophageal cancer patients received daily synbiotics (Bifidobacterium breve Yakult, Lactobacillus casei Shirota, and galacto-oligosaccharides) at a dose of 3 g during 6 weeks of neoadjuvant chemotherapy and presented a lower frequency of severe lymphopenia, febrile neutropenia and diarrhoea(Reference Motoori, Yano and Miyata142), constituting clinical characteristics which are also seen in COVID-19 patients(Reference Azkur, Akdis and Azkur143–Reference Feng, Li and Sun146).
A therapeutic approach with prebiotics, probiotics and synbiotics can modulate other key points in the severity of COVID-19 cases: (a) increased production of Treg cells to control inflammation(Reference Chowdhury, Cámara and Verma147–Reference Dwivedi, Kumar and Laddha149); (b) reduced ferritin synthesis and regulation of iron metabolism by polyphenols (natural iron chelators), helping to reduce inflammation and oxidative stress(Reference Speer, D’Cunha and Botek150–Reference Lakey-Beitia, Burillo and La Penna152); (c) reduced D-dimer level involved in COVID-19 coagulopathy(Reference Stiksrud, Nowak and Nwosu153); (d) increase in immune efficacy of COVID-19 vaccine(Reference Yeh, Shih and Liu154,Reference Lei, Shih and Liu155) ; and (e) reduced occurrence of persistent post-COVID-19 symptoms such as dyspnoea, tiredness, and joint and chest pain(Reference Carfì, Bernabei and Landi156).
It is important to warn that the consumption of prebiotics should be planned according to the health status of the subject, as some prebiotics (i.e. GOS and FOS) cause increased intestinal osmolality and gastrointestinal symptoms in patients with irritable bowel syndrome(Reference Schumann, Klose and Lauche157). Also, some microbial strains can cause bacteraemia (e.g. Lactobacillus strains rhamnosus, acidophilus, casei and GG; Bacillus subtilis; Bifidobacterium longum and B. breve), sepsis (B. infantis) and fungaemia (i.e. Saccharomyces boulardii and S. cerevisiae) in immunocompromised subjects. Therefore, this situation requires adequate quality control of administered probiotic micro-organisms(Reference Sotoudegan, Daniali and Hassani158–Reference Costa, Moreira and Lorenzo160).
Another concern is that the global market still offers products with contaminants such as pathogenic bacteria which cause adverse effects to consumer health, mainly in vulnerable populations; incorrect identification of prebiotic components and probiotic strains; and reduced functional properties over product useful life. Thus, competent organisations must create rigorous regulations of production and marketing to guarantee the consumption of safe and effective products(Reference Gibson, Hutkins and Sanders86,Reference Vermeulen, Luijendijk and van Toledo161–Reference Swanson, Gibson and Hutkins163) .
Traditionally consumed probiotics are generally recognised as safe (GRAS) or Qualified Presumption of Safety (QPS), according to the Food and Drug Administration (FDA) and European Food Safety Authority (EFSA), respectively(164,Reference Koutsoumanis and Allende165) . Even with the growing number of studies about health and immune benefits of probiotics, these claims are not yet approved by the FDA and EFSA. Prebiotics is also not yet a term recognised by the FDA or the European Union(Reference Gibson, Hutkins and Sanders86). Thus, assessing the safety of consuming prebiotics and probiotics which are intended to be used in a therapeutic category is essential; their safe intake level must be established, and they must obtain government approval for consumption(Reference Kumar, Salminen and Verhagen166). However, it is emphasised that the legislation or regulatory guidelines applied to prebiotics and probiotics vary significantly among countries, which in this time of the COVID-19 pandemic highlights the need to build globally uniform standards(Reference Verma, Niamah and Patel167).
Future perspectives
Due to the urgency to find ways to prevent and minimise COVID-19 symptoms and its drug treatment associated with gut dysbiosis(Reference Dhar and Mohanty7), the consumption of probiotics, prebiotics and synbiotics can be encouraged given the levels of scientific evidence which have demonstrated its beneficial effects on health, especially on the immune system and at the systemic level, provided they are combined with a healthy diet(Reference Morais, Passos and Maciel168). It should also be considered that several fresh and processed foods, as well as supplements available on the market, have prebiotic and probiotic properties which can facilitate consumption even during social distancing(Reference Markowiak and Śliżewska90). Walton et al.(Reference Walton, Gibson and Hunter14) emphasise that the dietetic approaches discussed have a scientific basis in addition to generally being safe and simple to implement. Despite vaccination and pharmaceutical treatments, the search for supporting strategies that reduce syndrome severity and duration can not only bring benefits to individuals affected by COVID-19 but also to health systems worldwide, mainly due to the emergence of new variants of the SARS-CoV-2 virus(Reference Walton, Gibson and Hunter14).
We emphasise that specialists (i.e. United Kingdom All-Party Parliamentary Group (APPG) on the Human Microbiome; International Society for Immunonutrition; International Scientific Association for Probiotics and Prebiotics; World Gastroenterology Organisation; Institute for the Advancement of Food and Nutrition Sciences: International Society of Microbiota; and others) in conjunction with government actions should plan and develop guidelines to inform the population about the proper use of prebiotics, probiotics and synbiotics in disease and health to avoid indiscriminate consumption. An example of this effort was made by APPG on the Human Microbiome when it sent the ‘Call for a government evaluation of the link between nutrition and the gut microbiome with respect to the COVID-19 pandemic’ for the Secretary of State for Health and Social Care of the United Kingdom. This statement points out the safe use of specific probiotics and/or prebiotics in COVID-19 treatment to achieve a healthy microbiota which benefits the human immune system(Reference Gibson and Calder169).
In this scenario, future studies on animal models, as well as prospective, multicentre randomised, triple- or double-blind, placebo-controlled clinical studies, need to be conducted involving the administration of different probiotic strains and prebiotic components, either isolated or combined to obtain synergistic effects with the establishment of doses, intervention time and action mechanisms against COVID-19. The studies should focus on prevention, the adjuvant treatment of mild to moderate symptoms of COVID-19, and the remission period of this disease, and should also consider inter-individual variability, especially in individuals who have chronic diseases capable of altering the gut microbiota.
Acknowledgements
This work was supported by the Improvement of Higher Education Personnel (Coordenação de Aperfeiçoamento de Pessoal de Nível Superior) – CAPES, Brazil (K.S.B., M.H.A.V., M.L.R.B. and J.G.d.A., grant number 0001) and the Consejo Nacional de Ciencia y Tecnología – Conacyt, Mexico, (J.G.d.A., grant number 921107). CAPES and Conacyt had no role in the design, analysis or writing of this article.
All authors contributed equally to the elaboration of the manuscript. K.S.B., J.G.d.A., M.H.A.V., M.L.R.B., M.B.S.B., R.O.P and J.S.A. contributed to the conceptualisation, data curation, investigation, methodology, and roles/writing – original draft. K.S.B. and J.S.A. supervised the project.
The authors have no conflicts of interest.
Supplementary material
For supplementary material/s referred to in th5 is article, please visit https://doi.org/10.1017/S0954422421000317