Introduction
Olive oil is defined as a common vegetable oil of culinary use, extracted from olives, fruits of the Olea europaea tree. This tree originates from the Upper Miocene, 20 million years ago. Since then, olive oil, recently named ‘liquid gold’, has been considered a Mediterranean treasure, and according to Georges Duhamel, French doctor, writer and poet, ‘the Mediterranean finishes where no grow the olives’. In Ancient Egypt, beneficial and healing properties were attributed to olive oil, as well as a divine character, with the olive tree being a signal of peace and prosperity. Nowadays, olive oil is consumed daily worldwide, being one of the fundamental pillars of the healthy Mediterranean diet, together with other foods such as fresh fruits, vegetables, legumes, seeds, moderate amounts of red wine, fish and meat rich in unsaturated fats. Scientific literature has revealed health benefits associated with olive oil consumption as well as its relationship with a lower prevalence of vascular disease, obesity, arthritis, cancer and age-associated cognitive decline, even the onset Alzheimer’s disease (AD)(Reference Román, Jackson and Reis1). However, in the last decade, the scientific community has investigated what bioactive components in olive oil cause these health benefits. Leaving aside unsaturated fatty acids and minor compounds, numerous studies have reported that olive polyphenols, predominantly present in extra virgin olive oil (EVOO), are responsible for most of the properties described so far. In fact, the European Food Safety Authority (EFSA) issued a health claim for EVOO, reporting that EVOO ‘contributes to the protection of blood lipids from oxidative stress’, providing that it contains at least 250 mg/kg of olive polyphenolic compounds(2). The olive polyphenol content depends on multiple factors (climate in place of cultivation, variety of the olive, level of ripening at the time of harvest and extraction method). Currently, some olive polyphenols have been recognised by the EFSA to have beneficial antioxidant properties at a minimum dose of 5 mg in 20 g of EVOO(Reference López-Huertas, Lozano-Sánchez and Segura-Carretero3). However, another study has reported that the antioxidant role of polyphenols in EVOO regarding rat redox status depends on the tissue. Specifically, this dose was beneficial for brain, blood, muscle and small intestine, and damaging for other tissues(Reference Kouka, Tekos and Papoutsaki4). To date, no studies have attributed to olive polyphenols a harmful effect on health, since both in vitro and in vivo toxicological studies have produced corroborating results(Reference Auñon-Calles, Giordano and Bohnenberger5,Reference Auñon-Calles, Canut and Visioli6) .
Nevertheless, we must consider that these polyphenols are also found in many other foods of vegetable origin typical in the Mediterranean diet(Reference Leri, Scuto and Ontario7). Hence, the daily intake of a substantial number of polyphenols from the Mediterranean diet reduces the incidence of many ageing-associated diseases, among them AD(Reference Abbatecola, Russo and Barbieri8). The Prevention with the Mediterranean Diet (PREDIMED) trial reported that a Mediterranean diet supplemented with EVOO and/or nuts, both foods rich in numerous bioactive polyphenols(Reference Manach, Scalbert and Morand9), provided beneficial effects for the prevention of chronic diseases(Reference Estruch, Ros and Salas-Salvado10,Reference Martinez-Gonzalez, Salas-Salvado and Estruch11) . Specifically, Scarmeas and collaborators reported a close link between intake of Mediterranean diet and a reduction in chronic diseases(Reference Scarmeas, Stern and Tang12). Furthermore, an interventional pilot study targeted to adults at risk of AD has suggested that a Mediterranean ketogenic diet supplemented with olive oil may prevent cognitive decline(Reference Neth, Mintz and Whitlow13). Moreover, Mediterranean-diet-induced structural benefits were observed in brain of older adults(Reference Gu, Brickman and Stem14). The health effects of wine are debated regarding prevention of or therapeutic approaches to different diseases, including AD. Polyphenols have antioxidant properties, which can attenuate oxidative damage in cells and tissues(Reference Peters, Peters and Warner15). However, the antioxidant effect is at the centre of the controversy concerning wine health benefits. Some scientists argue that the beneficial effects of wine were determined in studies with methodological limitations, which may have led to misinterpretations or biased conclusions(Reference Naimi, Stadtmueller and Chikritzhs16). In fact, most polyphenols have very low bioavailability and are largely transformed in the body. Maximum plasma levels of conjugated phenolic metabolites are reached between 1 and 2 h or 6 and 24 h after intake for the ones derived from small intestine absorption or the ones produced by the gut microbiota, respectively(Reference Teng and Chen17). Additionally, the antioxidant effect of conjugated metabolites is lower than that of the parent polyphenols because of the glycosylation, sulphation or methylation reactions(Reference Sobhani, Farzaei and Kiani18). Conversely, other researchers argue that antioxidant effects of the metabolite mixture occurring in vivo are higher than those of the individual compounds in vitro (Reference Wiczkowski, Szawara-Nowak and Topolska19). Moreover, literature suggests that flavonoid aglycones and/or their methylated derivatives may be active in the body since cells contain enzymes able to deconjugate the sulphated and glucuronidated polyphenol forms. Researchers who report beneficial health effects of wine also argue that polyphenol activity goes beyond antioxidant effects, since polyphenols may act as potential modulators of intracellular signalling cascades vital to cells(Reference Cueva, Gil-Sanchez and Ayuda-Duran20).
Among people aged 60 years and over, AD is the most common form of dementia-related neurodegenerative disease, characterised by cognitive function and memory impairments. Sporadic AD is an irreversible neurodegenerative disorder triggered by multiple factors. Neuropathologically, it is characterised by the presence of extracellular amyloid beta peptide (Aβ) plaques and intracellular neurofibrillary tangles (NFTs) of hyperphosphorylated tau protein in hippocampus and cerebral cortex(Reference Long and Holtzman21). These cerebral aggregations, favoured by decreased autophagy, induce oxidative stress accompanied by exacerbated mitochondrial dysfunction and neuroinflammation. Furthermore, the impairment of acetylcholinesterase activity and glutamate activation of N-methyl-d-aspartate receptors contribute to the neurological dysfunction and symptoms. Many recent publications have observed that the insulin resistance present in the brain of AD patients is closely associated with these cerebral formations(Reference Neth and Craft22). In summary, AD is a multifactorial disorder in which these cerebral alterations impair synapsis processes, inducing a progressive loss of neurons. Therefore, to demonstrate the neuroprotective role of polyphenols, their effects on each of these pathophysiological changes have been evaluated. In summary, polyphenols are natural substances, synthesised by plants. Their structure consists of at least two phenyl rings and one or more hydroxyl substituents, encompassing a wide range of molecules(Reference Sigla, Dubey and Garg23). Regarding their main functions, polyphenols are antioxidative, and they have protective effects against acute and chronic neurodegeneration, since they prevent Aβ polymerisation, modulate autophagy, induce mitochondrial biogenesis and protect against peripheral insulin resistance, among other effects(Reference Angeloni, Malaguti and Barbalace24).
This review focuses on the evidence regarding the new neuroprotective role in AD granted to polyphenols from EVOO and wine.
Bioactive components from olive oil and wine
Olive oil
Olive oil, and its production variant EVOO obtained directly from mechanical extraction of olives, is universally known for its health benefits and nutritional value due to the presence of several bioactive components such as monounsaturated and polyunsaturated fatty acids (MUFAs and PUFAs), polyphenols, squalene, triterpenes, phytosterols, tocopherols and different pigments. These bioactive compounds give EVOO certain properties (anti-inflammatory, anti-microbial, anti-tumoral, protective against neurodegenerative diseases) which have given it the nickname of ‘liquid gold’, being considered a superfood and symbol of the Mediterranean diet(Reference Piroddi, Albini and Fabiani25).
EVOO is composed mainly of triacylglycerols, containing a high number of fatty acids and, specifically, a considerable MUFA content (65–83 %). On the one hand, unsaturated acids account for up to 85 % of its composition, since it has a high content of oleic acid (C18:1), which may range between 70 % and 85 %, and other unsaturated acids such as palmitoleic acid (16:1) or linoleic acid (C18:2). On the other hand, palmitic and stearic acids are the principal saturated acid of EVOO, accounting for 14 % of its composition(Reference Jimenez-Lopez, Carpena and Lourenço-Lopes26). Oleic acid has effects on cardiovascular risk factors as well as on metabolic and gastrointestinal functions. Some of the biological effects of this MUFA are attenuation of inflammation and oxidative states in fasting conditions, modifications to lipoproteins and plasma lipid patterns, inhibition of coagulation, improvements in blood pressure and glucose homoeostasis and effects on membrane fluidity and composition of blood cells(Reference Piroddi, Albini and Fabiani25). It is also important to highlight some crucial PUFAs, such as linolenic acid (C18:3) and linoleic acid. These two PUFAs are fundamental for the development of the brain and retina and are basic components of the cell structure(Reference Piroddi, Albini and Fabiani25).
The main antioxidant compounds found in EVOO are polyphenols. The principal phenolic subclasses present in EVOO are phenolic alcohols, such as oleocanthal, oleuropein, oleuroside and oleacein, flavonoids, lignans, phenolic acids and secoiridoids. Due to the ageing and production of EVOO, secoiridoids are hydrolysed and transformed into free hydroxytyrosol (HT) and tyrosol(Reference Angeloni, Malaguti and Barbalace24). HT, which is present at a mean concentration of 137 mg/kg in olive oil, has many beneficial effects on human health, since it can stimulate several antioxidant mechanisms in the body and reduce reactive oxygen/nitrogen species as well as free radicals. In addition, it has been proposed that HT could play an important role in neuroprotection against several degenerative diseases like AD, since it reduces insulin resistance, mammalian target of rapamycin (mTOR) activation, nuclear factor-kappa beta (NF-κB) translocation and protein oxidation, among other effects(Reference Gorzynik-Debicka, Przychodzen and Cappello27).
Among the minority bioactive components of EVOO, squalene, accounting for up to 0·7 % (7 mg/g), is a crucial intermediate in the biosynthetic pathway of sterols in animals, also playing a key role as an intermediate metabolite in cholesterol metabolism, participating in the regulation of its absorption, synthesis, esterification and elimination. Furthermore, its antioxidant and anti-cancer properties have been demonstrated (Fig. 1)(Reference Sánchez-Fidalgo, Villegas and Rosillo28).
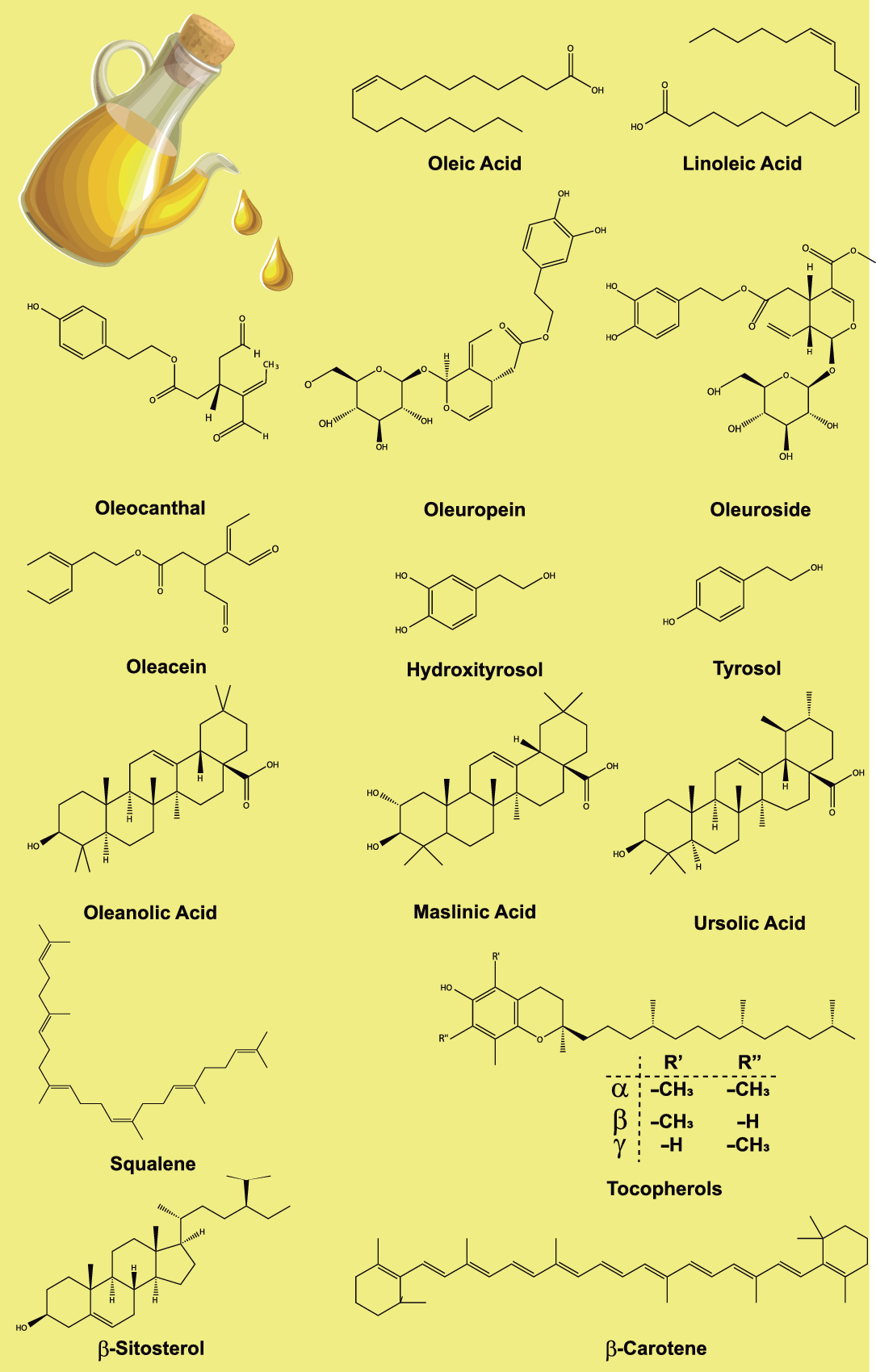
Fig. 1. Representative chemical structures of some relevant compounds present in extra virgin olive oil
Other bioactive molecules that appear in low concentration in EVOO are triterpenes. Specifically, oleanolic acid is present in concentrations between 17 and 344 mg/kg and maslinic acid between 19 and 250 mg/kg, and only traces of ursolic acid have been detected(Reference Piroddi, Albini and Fabiani25). The main biological activities related to these triterpenes are their anti-inflammatory, anti-cancer, anti-viral and several important neuroprotective effects, since oleanolic acid has a protective role in the brain of rats during hypoxic injury(Reference Rodríguez-Morató, Xicota and Fitó29). Furthermore, maslinic acid protects cortical neurons against oxygen–glucose deprivation-induced injury and glutamate toxicity, and ursolic acid has been described as protective against ischaemic injury in the brain of mice(Reference Rodríguez-Morató, Xicota and Fitó29).
More than 250 phytosterols have been identified, with β-sitosterol being the most prevalent. They are the principal unsaponifiable molecules in EVOO, with a concentration ranging from 70 to 260 mg/100 g. In addition, they have a similar structure and function to cholesterol, being crucial structural components of the cellular membrane having fundamental functions such as regulating membrane fluidity and permeability. Specifically, it has been described that phytosterols may play a role in signal transmission through membranes, improving neuronal plasticity. Furthermore, they can interact with proteins and other lipids in the cell membrane(Reference Moreau, Nyström and Whitaker30,Reference Vezza, Canet and De Marañón31) .
Regarding tocopherols, three isoforms of tocopherols are present in EVOO: α-, β- and γ- tocopherol, with α-tocopherol being the most abundant with a range of variability between 191·5 and 292·7 mg/kg of oil, depending on several variables such as year of harvest(Reference Jimenez-Lopez, Carpena and Lourenço-Lopes26). Their main function is to act as antioxidants and prevent lipid peroxidation of cellular membranes and lipoproteins(Reference Jimenez-Lopez, Carpena and Lourenço-Lopes26).
Finally, according to Jimenez-Lopez et al.(Reference Jimenez-Lopez, Carpena and Lourenço-Lopes26), EVOO contains a large variety of pigments, such as carotenoids and chlorophylls. These pigments can be detected in amounts up to 100 ppm of total carotenoids, with a concentration of β-carotene as high as 15 ppm. However, these values can vary depending on several factors such as the geographic origin of the EVOO. The main beneficial effects of these pigments are their antioxidant capacity and protective effect against the formation of age-related cataracts; specific subtypes of age-related cataracts are related etiologically with AD since genetic variation in delta catenin may cause cortical lens opacities and cognitive changes that could promote the development of AD(Reference Jimenez-Lopez, Carpena and Lourenço-Lopes26,Reference Jun, Moncaster and Koutras32) .
Wine
Wine is composed of water and ethanol, which together account for about 97 % on a weight-for-weight (w/w) basis. Wine ethanol results from grape sugar transformation during alcoholic fermentation. This hydroalcoholic solution contains a small percentage of several hundreds of compounds, some having their origin in grapes and others in yeast activity during fermentation. Among those compounds are polyphenols, which give red wine its flavour and colour. Polyphenols comprise a diverse range of molecules with one or more aromatic rings containing at least one hydroxyl group. Wine polyphenols are divided into two major groups: flavonoid and non-flavonoid. Flavonoids include flavonols, flavononols (also known as dihydroflavonols), anthocyanins, flavan-3-ols, flavanones and flavones. Non-flavonoid compounds include hydroxycinnamic and hydroxybenzoic acids, and stilbenes (Fig. 2)(Reference Visioli, Panaite and Tome-Carneiro33). Wine polyphenol profile and concentration depends on terroir, that is, it varies with grape variety, climate, soil and the oenological practices applied for winemaking and ageing and storage(Reference Prata-Sena, Castro-Carvalho and Nunes34). For example, flavonoids are the predominant phenolic components in both young and aged red wines; however, in young wines, the top three most abundant flavonoids are flavan-3-ols oligomers and polymers (750 mg/L), anthocyanidins (400 mg/L) and flavonols (100 mg/L), while in aged wine they are flavan-3-ols oligomers and polymers (1000 mg/L), flavonols (200 mg/L) and anthocyanidins (90 mg/L). Regarding non-flavonoid compounds, in young red wine the most representative ones are hydroxycinnamic acids (165 mg/L), while in aged red wine they are hydrolysable tannins (250 mg/L). Stilbenes are a non-flavonoid subclass whose concentration does not change with age (7 mg/L)(Reference Caruana, Cauchi and Vassallo35).

Fig. 2. Representative chemical structures of some relevant compounds present in wine
As previously mentioned, Aβ aggregation and the appearance of NFTs are the major hallmarks of AD (Reference Long and Holtzman21). Regarding wine, the major focus of research has been the evaluation of polyphenols’ potential for blocking Aβ aggregation in the brain. Several studies have been carried out assessing the potential of wine polyphenols to prevent the appearance of hyperphosphorylated tau protein aggregates. The metabolism of Aβ and tau is critically affected by autophagy(Reference Li, Liu and Sun36). Therefore, some trials have evaluated the potential of wine polyphenols as regulators of autophagy. Central nervous system diseases, including AD, are characterised by impaired mitochondrial bioenergetics, and often disturbed mitochondrial dynamics, which leads to neuronal death due to inadequate respiratory chain function and reduced ATP production(Reference Tillement, Lecanu and Papadopoulos37). Therefore, some authors explored the role of wine polyphenols in mitochondrial function, as a possible preventive mechanism of AD. Obesity, type 2 diabetes and insulin resistance are risk factors for the development of AD(Reference Neth and Craft22), and the role of wine polyphenols in this relationship have also been studied.
Next, we will review the main studies focused on the role of the majority polyphenols from EVOO and wine in the prevention/treatment of AD.
Neuroprotective effects of olive oil and wine polyphenols in Alzheimer disease
Aβ aggregation and toxicity
Olive oil
According to the ‘Amyloid Cascade Hypothesis’ one of the main causes of AD is overexpression and aggregation of Aβ in the cellular membrane, mainly around the synapse of neuronal tissue. There is a transmembrane glycoprotein called amyloid protein precursor (APP), which can be processed through two different pathways. In the non-amyloidogenic pathway, APP is cleaved by α-secretase and γ-secretase producing a soluble extracellular APP fragment (sAPPα), a short extracellular p3 peptide and a cytosolic APP intracellular domain (AICD). However, APP can be processed by β- (β-site amyloid precursor protein cleaving enzyme-1; BACE-1) and γ-secretase. This pathway is the amyloidogenic and produces Aβ which spontaneously aggregates into soluble oligomers and combines to form insoluble fibrils which are eventually deposited in diffuse senile plaques. The neurotoxic effect of Aβ depends on its state of aggregation, in which oligomers and protofibrils are more toxic than soluble monomers and mature fibrils(Reference Long and Holtzman21).
A possible role of polyphenols in the formation of these protein aggregates has been investigated for many years. More than forty polyphenolic compounds prevent Aβ polymerisation or joining to certain ions, inhibiting the aggregation of this peptide(Reference Velander, Wu and Henderson38). Leri et al.(Reference Leri, Natalello and Bruzzone39) demonstrated, by physical analysis experiments, that oleuropein inhibits the growth of oligomers Aβ1–42 and the formation of mature fibrils, and HT promotes the formation of non-toxic fibrils. Moreover, both polyphenols reduce the capacity of Aβ aggregates to interact with cell membranes and Aβ toxicity(Reference Leri, Natalello and Bruzzone39). Other important findings have been obtained from in vivo AD models, such as TgCRND8 mouse, in which oleuropein treatment reduces Aβ deposits in different cortical areas and in hippocampus, due to positive effect on the process of autophagy(Reference Grossi, Rigacci and Ambrosini40,Reference Luccarini, Grossi and Rigacci41) . A study on molecular mechanism revealed that oleuropein improves the clearance of Aβ deposits from neurons by up-regulation of autophagy-related genes, human beclin 1 gene (BECN1), Atg8/microtubule-associated light chain 3C (LC3), p62 and cathepsin B to accelerate the autophagy process(Reference Pantano, Luccarini and Nardiello42).
Wine
The potential AD benefits of rat brain-targeted polyphenols, assessed after red wine oral administration, were evaluated in primary neuron cultures originated from the AD-type amyloid-β neuropathology mouse model (Tg2576). Quercetin-3-O-glucuronide (0–5 µM) interfered with the early protein–protein interaction of Aβ1–40 and Aβ1–42, which promoted a decrease in the occurrence of neurotoxic oligomeric Aβ species(Reference Ho, Ferruzzi and Janle43). A different study showed that muscadine wine reduces Aβ neuropathology and attenuates Tg2576 spatial memory decline. The results suggest that muscadine wine effects are associated with a decrease of soluble high-molecular-weight oligomeric Aβ species in the brain(Reference Ho, Chen and Wang44).
In vitro, amyloid β-protein aggregation into high-molecular-weight oligomers is also inhibited by grape seed polyphenolic extract (200 mg/kg/d). The same study showed that those oligomers are also reduced in the brain of Tg2576 mice when this grape extract is orally administered, which results in a decrease in AD-type cognitive decline. In particular, authors found that grape seed extract reduces the Aβ aggregate specifically associated with memory loss in rats(Reference Wang, Ho and Zhao45). A different study showed, through cytotoxicity assays, that grape seed polyphenolic extract has protective effects when mixed with Aβ prior to or after peptide assembly and just before peptide addition to cells(Reference Ono, Condron and Ho46). A posterior experimental trial showed that, when APPSwe/PS1dE9 transgenic AD mouse model is fed for 9 months with polyphenol-rich grape seed extract, depending on the analysis methods used, total brain amyloid burden is reduced by 33–45 %(Reference Wang, Thomas and Zhong47).
Some in vitro studies report that resveratrol, the most famous wine polyphenol, has an anti-Aβ aggregation effect. In SH-SY5Y human neuroblastoma cells, resveratrol inhibits Aβ42 peptide fibrillisation in a dose-dependent way (10–100 μM) and depolymerises pre-built fibrils, resulting in non-toxic oligomers(Reference Feng, Wang and Yang48). In an in vitro assay using the 25–35 Aβ peptide fragment, which preserves the properties of neurotoxicity and aggregation of the entire peptide, it was found that resveratrol inhibits fibril formation with a half-maximal inhibitory concentration value of 5·6 μM(Reference Riviere, Richard and Quentin49). The specific and decisive mechanism by which resveratrol blocks in vitro aggregation is unknown, although several proposals have been made. Low-molecular-weight oligomers (20 kDa, tetramer) are stabilised when Aβ42 is co-incubated with resveratrol, and this remodelled resveratrol-capped oligomers has shown reduced cell toxicity(Reference Fu, Aucoin and Ahmed50). In this atomic force microscopy study, it was also observed that resveratrol binds to Aβ1–42 monomers at their N-terminus(Reference Fu, Aucoin and Ahmed50), which is consistent with studies showing that resveratrol can also bind directly to Aβ in different states, including monomer and fibril Aβ, inhibiting Aβ42 fibril formation(Reference Feng, Wang and Yang48,Reference Ge, Qiao and Qi51) . A different study showed that resveratrol can remodel soluble prefibrillar oligomers, fibrillar intermediates and fibrils into non-toxic alternative aggregated species(Reference Ladiwala, Lin and Bale52). Administration of resveratrol for 45 d diminishes β-amyloid plaque formation in mouse brain, with the largest decrease in the plaque area proportion in the medial cortex (48 %), striatum (89 %) and hypothalamus (90 %)(Reference Karuppagounder, Pinto and Xu53). Resveratrol can protect against Aβ plaque formation in APP/PS1 mouse hippocampus and prevent memory loss(Reference Porquet, Grinan-Ferre and Ferrer54). The decrease of Aβ levels and its extracellular accumulation in animals treated with resveratrol can also be mediated by adenosine monophosphate-activated protein kinase (AMPK)(Reference Porquet, Casadesus and Bayod55,Reference Vingtdeux, Giliberto and Zhao56) . Resveratrol can also prevent Aβ aggregation and storage through the stabilisation of transthyretin, which increases its circulating levels and promotes its assembly to Aβ peptide(Reference Santos, Rodrigues and Alemi57). Based on these studies, several derivatives of resveratrol were designed and synthesised as AD multi-targeted therapeutic agents(Reference Li, Wang and Kong58–Reference Xu, Zhang and Sheng60).
Quercetin has also a potent Aβ42 anti-aggregation effect (75·4 %), as shown in a study including both in vitro cell-based and in silico approaches(Reference Espargaro, Ginex and Vadell61). A different in vitro study showed that the OH– functional groups of quercetin B-ring are decisive for its potential to inhibit fibril aggregation. In accordance with this study’s results, quercetin was also shown to disrupt the mature fibrils(Reference Suganthy, Devi and Nabavi62). Quercetin decreases both number and area of amyloid plaques in the hippocampus and cortex of AD mice(Reference Wang, Li and Wu63). Quercetin treatment (25 mg/kg) for 3 months decreases β-amyloidosis in the 3xTg-AD model, contributing to cognitive protection and emotional function in animals(Reference Sabogal-Guaqueta, Munoz-Manco and Ramirez-Pineda64). Quercetin orally administered to 5xFAD amyloid model mice reduces cortex insoluble Aβ levels(Reference Zhang, Hu and Zhong65). Long-term (12 months) oral quercetin (100 mg/kg) administration to the 3xTg-AD mouse model has significant effects on β-amyloidosis(Reference Paula, Angelica Maria and Luis66). To improve polyphenol bioavailability, stability and ability to cross the blood–brain barrier (BBB), nano-based delivery systems have been developed. Recently, the protective and therapeutic effects of quercetin nanoparticles (30 mg/kg) were investigated in an aluminium chloride (AlCl3)-induced animal model of AD, and it was observed that they remarkably reduce the development of amyloid plaques(Reference Rifaai, Mokhemer and Saber67).
Gallic acid has also alleviated cognitive decline of APP/PS1 double transgenic mice through reduction of Aβ1–42 aggregation and neurotoxicity(Reference Yu, Chen and Liu68).
Neurofibrillary tangles
Olive oil
The presence of intracellular NFTs is another pathological marker of AD. These NFTs are composed of tau protein in a hyperphosphorylated state. Under normal conditions, tau protein provides stability to the microtubules for the proper functioning of the neuron, and its activity depends on its phosphorylation. In AD, tau is hyperphosphorylated and appears in different locations and not in the microtubules forming abnormal filaments, composed of tau and other microtubules associated with proteins. In this way, the microtubule is more unstable, so there are alterations in cellular transport, ultimately contributing to neuronal death. Cyclin-dependent kinase 5 (CDK5) and glycogen synthase kinase 3β (GSK3β) phosphorylate tau, and protein phosphatase 2 A (PP2A) is the main phosphatase that dephosphorylates tau, acting on specific phosphorylation sites(Reference Iqbal and Grundke-Iqbal69). It has been demonstrated. by physical assays in vitro, that oleuropein, oleocanthal and HT inhibit toxic aggregation of tau(Reference Rigacci and Stefani70).
Wine
Resveratrol increases in vitro tau dephosphorylation through down-regulation of extracellular signal-regulated kinases (ERK)1/2 and GSK3β, with the latter being a key factor for tau protein hyperphosphorylation and NFT genesis(Reference Jhang, Park and Kim71). Resveratrol blocking of tau protein hyperphosphorylation, throug GSK3β inhibition, was also observed using a mouse neuroblastoma cell line widely used to study neurotoxicity (N2a cells)(Reference He, Li and Rizak72,Reference Sun, Dong and Zhu73) . Moreover, resveratrol (2·5–50 µM) could inhibit tau protein hyperphosphorylation both by the blocking of calmodulin-dependent protein kinase II (CaMKII) and the triggering of protein PP2A(Reference He, Li and Rizak72). PP2A is the most important phosphatase that dephosphorylates tau, so its activation is a good strategy in the prevention and therapy of AD. It was shown that daily intraperitoneal injections of resveratrol (25 mg/kg) in mice, for 2 weeks, increase PP2A activity by reducing microtubule-associated ubiquitin ligase (MID1) expression, through its proteasomal breakdown and subsequent destabilisation of its mRNA(Reference Schweiger, Matthes and Posey74). Recently, it was shown that resveratrol prevents caspase-3 activation, tau dephosphorylation at Ser422 and genesis of misfolded protein aggregates, which protects optic nerve head astrocytes from oxidative-stress-induced cell death(Reference Means, Lopez and Koulen75). When 6-month-old male PS19 mice (expressing mutant human microtubule-associated protein tau) were treated with resveratrol, orally administered (gavage) (40 mg/kg body weight) once a day for 5 weeks, it was observed that resveratrol suppresses tau assemblage and tau oligomer-induced cytotoxicity(Reference Sun, Dong and Zhu73). Resveratrol decreases tau levels and improves cognitive function in rats(Reference Al-Bishri, Hamza and Farran76, Reference Lin, Wu and Sun77). Moreover, in rodents in which early AD was induced by angiotensin-II, these results where related with the reducing activity of the Aβ–caspase3–Akt–GSK3β-tau pathway(Reference Lin, Wu and Sun77). Both GSK3β and CDK5 activity decreases in resveratrol-fed senescence accelerated mouse (SAMP8), confirming that the inhibition of these two major tau kinases in AD prevents tau phosphorylation in the cortex and hippocampus of the animals(Reference Porquet, Casadesus and Bayod55).
Quercetin treatment attenuates tau phosphorylation via suppressing ER stress/NLRP3 (endoplasmic reticulum/Nod-like receptor family pyrin domain containing 3) inflammasome trigger in SH-SY5Y cell line(Reference Chen, Deng and Liu78). Quercetin reduces okadaic-acid-induced tau protein hyperphosphorylation, inhibits the activity of CDK5 and blocks the Ca2+–calpain–p25–CDK5 signalling pathway in murine hippocampal neuronal HT22 cells(Reference Shen, Luo and Li79). Decline of tauopathy in the hippocampus and amygdala of 3xTg-AD mouse model was observed after a 12-month treatment with quercetin (100 mg/kg) given orally; furthermore, this polyphenol improves cognitive functional recovery without altering emotional skills(Reference Paula, Angelica Maria and Luis66). Quercetin nanoparticles (30 mg/kg) reduce the neuronal degenerative changes by reducing NFTs(Reference Rifaai, Mokhemer and Saber67).
Anthocyanin-loaded polyethylene glycol gold nanoparticles administered to amyloid beta (Aβ)1–42 mouse model of Alzheimer’s disease regulate the p-PI3K/p-Akt/p-GSK3β (phosphoinositide 3-kinase/phospho-protein kinase B/phospho-glycogen synthase kinase 3 beta) pathway and consequently prevent tau protein at serines 413 and 404 hyperphosphorylation(Reference Ali, Kim, Rehman and Ahmad80).
Neuroprotective effects of gallic acid against AlCl3-induced AD in adult Wistar rat were recently studied, and histological analyses showed that NFTs and amyloid plaques in the external granular layer are protected by gallic acid(Reference Ogunlade, Adelakun and Agie81).
In vitro studies, with phaeochromocytoma cells (PC12), showed that caffeic acid inhibits calcium influx and tau phosphorylation, therefore ameliorating Aβ-induced neurotoxicity(Reference Sul, Kim and Lee82).
Autophagy
Olive oil
Autophagy is a ubiquitous physiological process through which old, damaged or poorly folded proteins or organelles are degraded in lysosomes. During ageing, autophagic activity decreases, and this fact has been linked to the onset of cancer, diabetes and various neuropathologies, including AD. In fact, it has been classified as a proteinopathy owing to the existence of aggregated proteins abnormally accumulated, which occurs early in AD development, and it is related to neuron apoptosis(Reference Li, Liu and Sun36). Therefore, autophagy is considered as a therapeutic target for this neuropathology. Many proteins mediate the autophagy process, one of them being the mammalian target of rapamycin complex 1 (mTORC1), which inhibits autophagy. This effect is due to a repressive effect of components such as unc-51-like autophagy-activating kinase 1 (ULK1) and autophagy-related proteins such ATG13 and ATG101, which play a key role in initiating the autophagic process(Reference Li, Liu and Sun36).
Polyphenols modulate autophagy through different pathways and different modes of action(Reference Benvenuto, Albonici and Focaccetti83). Oleuropein reduces aggregated proteins by a positive effect on autophagy inhibiting AMPK/mTOR, activation of gene expression of protein related to autophagy and acetylation of histone or EB transcription factor. As mentioned above, in the AD mouse model TgCRND8, which overexpresses the Swedish and Indiana mutations in the human amyloid precursor protein, oleuropein treatment reduces Aβ deposits in different brain areas. This effect is due to the activation of autophagy, by activating various genes related to the process such as, BECN1, LC3, p62 and cathepsin B, as well as the formation of autophagosome–lysosome to speed up the autophagy process(Reference Pantano, Luccarini and Nardiello42).
Wine
In the SH-SY5Y cell model, minimal concentrations of resveratrol (2 μM) block, in an autophagy-dependent manner, the effects of prion protein on mitochondrial transmembrane potential, Bcl-2-associated X protein (Bax) translocation to mitochondria, and cytochrome C release from the organelles(Reference Jeong, Moon and Bae84). Resveratrol induces autophagy, in dopaminergic SH-SY5Y cells challenged with rotenone via haem oxygenase(Reference Lin, Chen and Chuang85) and AMPK/SIRT1 (sirtuin-1) signalling(Reference Wu, Li and Zhu86). Moreover, neurotoxicity caused by Aβ25-35 in PC12 cells is attenuated by resveratrol, through inducing autophagy, which is partially mediated via activation of the TyrRS -PARP1-SIRT1 signalling pathway(Reference Deng and Mi87). A study with PC12 cells AD showed that resveratrol inhibits Aβ42-induced mitochondrial damage and increases mitophagy(Reference Wang, Jiang and Li88). In AD mouse models, resveratrol shows some protective effect against AD-like pathology through AMPK-mediated autophagy activation(Reference Vingtdeux, Giliberto and Zhao56).
Liu et al.(Reference Liu, Zhou and Yin89) synthesised quercetin modified polysorbate 80 (P-80)-coated AuPd core-shell structure and found that they induce autophagy of SH-SY5Y cells. In this study, quercetin promotes the fusion of autophagosomes and lysosomes, speeds up the Aβ clearance and protects SH-SY5Y cells from Aβ-induced cytotoxicity injury(Reference Liu, Zhou and Yin89).
Taken together, these results confirm that wine polyphenols can activate and/or promote autophagy to stimulate clearance of unwanted protein aggregates, and therefore they are a promising therapeutic approach against AD.
Mitochondrial function
Olive oil
In the pathophysiological frame of AD, mitochondrial energetic deficit is one of the early events involved in the advance of disease. Specifically, decreased mitochondrial ATP production as well as unbalanced mitochondrial dynamics have been shown in in vitro and in vivo studies of AD(Reference Reddy and Oliver90). These mitochondrial alterations are clinically translated to early memory loss because there is not enough energetic support to carry out the neurological processes. Recent studies have suggested that polyphenols from EVOO could ameliorate and/or revert the mitochondrial dysfunction characteristic of AD. Grewal et al.(Reference Grewal, Reutzel and Dilberger91) have reported that oleuroside, oleacein, ligstroside and oleocanthal elevated the ATP production in SH-SY5-APP659 cells and in the brain of an animal model of early AD. Moreover, other in vitro studies have demonstrated that oleocanthal and ligstroside induced an increase in mitochondrial biogenesis. These beneficial results were reflected in an improved spatial working memory and cognitive function in ligstroside-fed aged mice(Reference Grewal, Reutzel and Dilberger91). Along this same line, the beneficial and promising properties of HT, the foremost phenolic component of EVOO, on cerebral mitochondrial dysfunction are also noteworthy. Visioli and collaborators have currently demonstrated that HT increased ATP production, mitochondriogenesis and mitochondrial fusion in 7PA2 cells, a cellular model of AD with a well-characterised mitochondrial dysfunction(Reference Visioli, Rodríguez-Pérez and Gómez-Torres92). Other study reported that HT ameliorated mitochondrial dysfunction and cognitive behaviour in the brain of APP/PS1. However, the authors only analysed mitochondrial markers related to oxidative stress and inflammatory response Aβ-induced and not cellular energetic production(Reference Peng, Hou and Yang93). Additionally, it has been shown that the intake of a mixture of highly purified secoiridoid polyphenols improved energy metabolism in the brain of aged mice(Reference Reutzel, Grewal and Silaidos94). To date, all investigations seem to indicate that EVOO polyphenols exert remarkable neuroprotective effects on damaged cerebral mitochondria in AD, though appropriate clinical trials should be carried out to confirm these pre-clinical findings.
Wine
Resveratrol has some indirect mitochondrial effects, including decrease of oxidative stress and induction of mitochondrial biogenesis, through the activation of peroxisome proliferator-activated receptor gamma coactivator 1α (PGC1α). Following the work of Howitz et al., published in 2003, resveratrol has been one of the most-studied SIRT1 activator molecules(Reference Howitz, Bitterman and Cohen95). The mechanism of action is not fully elucidated, although several studies indicate that SIRTs mediate some of resveratrol effects(Reference Sanchez-Fidalgo, Villegas and Sanchez-Hidalgo96). Resveratrol up-regulates SIRT1-mediated mitochondrial biogenesis and stimulates AMPK and PGC-1α functions. In cell culture, activation of AMPK by resveratrol occurs by increasing cytosolic calcium levels, and by promoting phosphorylation of AMPK through the calcium/calmodulin-dependent protein kinase kinase β (CaMKK β) pathway. When orally administered, resveratrol crosses the mice BBB and activates brain AMPK(Reference Vingtdeux, Giliberto and Zhao56). In SH-SY5Y neuronal cells, resveratrol ameliorates mitochondria oxidative stress, apoptosis and cell death induced by hypoxia by improving transient receptor potential melastatin 2 (TRPM2) channel activation, decreasing mitochondrial oxidative stress and protecting the glutathione (GSH) and glutathione peroxidase (GPx) thiol redox system. This outcome results from an increase in the mitochondrial function restored via modulation of TRPM2 channel and calcium signalling(Reference Akyuva and Nazıroğlu97).
Quercetin (40 mg/kg) alleviates Aβ-induced mitochondrial dysfunction as evidenced by mitochondrial membrane potential recovery in APPswe/PS1dE9 transgenic mice. The results suggest that quercetin activates AMPK, which improves cognitive functioning in this animal model(Reference Wang, Li and Wu63).
Cerebral insulin resistance
Olive oil
Recently, epidemiological evidence has found insulin resistance in brain of AD patients because the insulin signalling pathway is blocked at the level of the insulin-receptor substrate 1 (IRS1) by Aβ-induced hyperphosphorylation(Reference Diehl, Mullins and Kapogiannis98). Consequently, at the cellular level, astrocytes do not capture glucose and do no transform it into lactate, the main fuel for neurons, exacerbating neuronal damage. A recent study has demonstrated that HT protects Aβ-treated astrocytes by improving insulin sensitivity, which suggests that HT as nutritional supplement could reduce insulin resistance associated with AD(Reference Crespo, Tomé-Carneiro and Pintado99). HT administration for 30 d also activated hippocampal neurogenesis and increased neuronal survival in aged mice(Reference D’Andrea, Ceccarelli and Bernini100). At the same stage, oleocanthal reverts the Aβ-induced glucose transporter type 1 (GLUT1) down-regulation in astrocytes, favouring astrocytic glucose uptake(Reference Batarseh, Mohamed and Al Rihani101). Many other polyphenols, such as oleacein, play a protective role in peripheral insulin resistance in obesity mice(Reference Cao, Xu and Zou102–Reference Lombardo, Lepore and Morittu104). Nevertheless, the effects on cerebral insulin resistance have not been analysed yet. On the whole, this evidence suggest that EVOO polyphenols could be used to improve the cerebral insulin resistance associated with AD. However, more in vitro and in vivo studies should be carried out to explain their mechanism of action in greater depth.
Wine
Memory-impairing effects of diet-induced obesity may be mediated by neuroepigenetic dysregulation of SIRT1 in the hippocampus. Resveratrol was administrated to a rat model of brain insulin resistance with intracerebroventricular injection of streptozotocin (ICV-STZ; 3 mg/kg, twice with an interval of 48 h) for 8 weeks (30 mg/kg, once per day). Resveratrol was able to reverse the ICV-STZ-induced decrease in SIRT1 activity and the increase in both ERK1/2 phosphorylation and tau phosphorylation. Consequently, resveratrol also reversed the impairment in cognitive capability(Reference Du, Xie and Cheng105). Similarly, obese mice fed a diet supplemented with resveratrol exhibited increased hippocampal SIRT1 activity and preserved hippocampus-dependent memory(Reference Heyward, Gilliam and Coleman106). In summary, resveratrol can prevent cognitive impairment induced by brain insulin resistance, by protecting hippocampus neurons from tau hyperphosphorylation through the SIRT1 pathway and from a decrease in hippocampus ERK1/2 activity. In a recent study, supplementation with 0·1 % resveratrol, over 16 weeks protected against high-fat diet (HFD)-induced memory loss in male wild-type mice and prevented memory loss in 5XFAD mice. These results confirm the potential of resveratrol to counteract the deleterious effects of this diet, by decreasing the excessive activation of proteasome and immunoproteasome, probably caused by metabolic stress(Reference Sarroca, Gatius and Rodríguez-Farré107).
Clinical trials
Olive oil
In the 1960s, population studies showed that inhabitants of the Mediterranean had a minor risk of developing AD and other ageing-associated disorders. Successive studies have demonstrated that consumption of Mediterranean diet is responsible for this reduced risk(Reference Manach, Scalbert and Morand9,Reference Martinez-Gonzalez, Salas-Salvado and Estruch11,Reference Scarmeas, Stern and Tang12) . Specifically, the randomised clinical trial PREDIMED-NAVARRA (ISRCTN:35739639) showed that a Mediterranean diet supplemented with EVOO (50 g/d) improved the cognitive function in 552 participants in comparison with a low-fat control diet, after 6·5 years of intervention(Reference Martínez-Lapiscina, Clavero and Toledo108). All evidence suggested that the foods of the Mediterranean diet, rich in polyphenols, are the most suitable for improving the cognitive function and stopping or preventing the onset of neurodegenerative disorders such as AD. A clinical trial aiming to evaluate the effect of EVOO (10 mg daily for 1 year) on AD is now recruiting (NCT: 04229186) patients between 50 and 70 years old, with mild cognitive impairment diagnosed. One group will receive EVOO and the other an olive oil poor in polyphenols for 12 months. They will examine biomarkers of neurodegeneration in cerebrospinal fluid (CSF), changes in cortical thickness, brain amyloid plaque load and neurological and neuropsychological features. A recent clinical trial (NCT03362996) has reported that EVOO annual consumption of 50 ml daily in older patients with mild cognitive impairment prevents progression of AD(Reference Tzekaki, Tsolaki and Pantazaki109). However, few nutritional intervention studies have been carried out to investigate the possible neuroprotective role of each phenolic component in EVOO. In this regard, we have identified two ongoing clinical trials: one will evaluate HT (NCT:04440020) effects, and the other oleocanthal (NCT:03824197), in patients with some clinical cognitive alteration diagnosed, such as memory loss or dementia. In both studies, cognitive imaging and neuroimaging will be carried out and several markers of neurodegeneration will be measured to demonstrate the neuroprotective effect of the polyphenols from olive oil. Currently, both clinical trials are recruiting patients between 55 and 85 years old, of both genders, with some clinical dementia diagnosed.
Wine
Regular wine consumption has been linked with a reduced risk of AD. The Canadian Study of Health and Aging showed that moderate wine consumption halved the risk of AD after 5 years follow-up(Reference Lindsay110). In the Italian Longitudinal Study on Aging, the influence of wine consumption on the incidence of mild cognitive impairment was evaluated in cognitively normal individuals and on its progression to dementia in 121 patients with mild cognitive impairment (65–84 years). The consumption of less than one drink of wine per day by patients with mild cognitive impairment decreased the rate of progression to dementia(Reference Solfrizzi, D’Introno and Colacicco111). Comparing current alcohol drinkers versus former or never drinkers, it was found that wine was also protective for dementia, with this effect being greatest among women who drank wine only (Göteborg Study)(Reference Mehlig, Skoog and Guo112). Moderate wine consumption was also independently associated with better cognitive results on all tests, after 7 years of follow-up, compared with low alcohol intake (Tromsø Study)(Reference Arntzen, Schirmer and Wilsgaard113).
Table grapes consumed twice daily (72 g), over 6 months, protected cerebral metabolism, which was correlated with improvement in attention/working memory performances as shown by a double-blind placebo-controlled longitudinal pilot study(Reference Lee, Torosyan and Silverman114).
Resveratrol administrated in single oral doses (250 and 500 mg) to healthy adults improved cerebral blood flow, but had no effect on short-term cognitive performance(Reference Kennedy, Wightman and Reay115). In healthy overweight older participants of a double-blind placebo-controlled interventional study, resveratrol supplementation (200 mg/d) over 26 weeks, improved their memory performance and functional connectivity of the hippocampus. It was concluded that resveratrol induces positive effects on glucose metabolism and subsequent neuronal function and cognitive performance(Reference Witte, Kerti and Margulies116). In a clinical phase 2 trial, 119 patients with mild to moderate AD, received 500 mg resveratrol per day for 52 weeks, and the dose was increased by 500 mg every 13 weeks. Compared with placebo, resveratrol promoted an increase in ventricular volume and a decrease in brain volume. Resveratrol also promoted a decrease of Aβ40 levels in plasma and CSF(Reference Turner, Thomas and Craft117). Resveratrol modulated neuro-inflammation and induced adaptive immunity in AD patients(Reference Moussa, Hebron and Huang118). A non-significant decrease in glycated haemoglobin A1c (HbA1c), which is a long-term marker of glucose control, was observed with a daily supplementation with 200 mg resveratrol over 26 weeks. Additionally, supplementation significantly increased resting-state functional connectivity between the right hippocampus and the right angular cortex and preserved the volume of the left, particularly anterior, hippocampus with a moderate effect size (non-significant). Memory performance and hippocampus microstructure did not improve with resveratrol supplementation(Reference Köbe, Witte and Schnelle119). Resveratrol administration to post-menopausal women (45–85 years) for 14 weeks improved the performance of cognitive tasks in the domain of verbal memory and in overall cognitive performance(Reference Evans, Howe and Wong120). In a more recent randomised placebo-controlled crossover trial, with postmenopausal women in the same age range, the consumption of 75 mg resveratrol twice daily for 12 months resulted in a 33 % improvement in overall cognitive performance. Moreover, resveratrol improved cerebrovascular function and insulin sensitivity in postmenopausal women(Reference Thaung Zaw, Howe and Wong121). A double-blind randomised controlled trial, in which elderly participants (60–79 years) received either resveratrol (200 mg/d) or placebo for 26 weeks, failed to show significant improvements in verbal memory(Reference Huhn, Beyer and Zhang122). Resveratrol neuroprotective benefits were not detected in a multicentric placebo-controlled phase 2 trial, in which AD patients were randomised to receive a dose of 500 mg of resveratrol every 13 weeks, up to 52 weeks(Reference Zhu, Grossman and Neugroschl123). These clinical trials show that resveratrol, at the doses administered, is safe and well tolerated; however, the beneficial effects of resveratrol in human clinical trials are far away from the ones observed in animal models. In general, there are no or small effects of resveratrol in human cognition. Several reasons can explain this difference, namely resveratrol dose/body weight and trial duration. In the future, better-designed long-term trials must be done to evaluate resveratrol efficacy. In Table 1, we recapitulate all the clinical trials mentioned above.
Table 1. Summary of the cited clinical trials in this review about polyphenols of EVOO and wine on AD

EVOO, extra virgin olive oil; –, missing data/recruiting patients.
Conclusions
Numerous studies show us the beneficial effects of Mediterranean diet, not only for health but also in the therapeutic and preventive framework of neurodegenerative diseases. In this work, we have focused on reviewing the state of the art of polyphenols from olive oil and wine on prevention and/or treatment of AD. It is well known that life expectancy is progressively increasing; however, along with life expectancy, prevalence of neurodegenerative ageing-associated disease such as AD is also increasing. Therefore, national public health systems have had to increase the budgetary spending for caring and mitigating the symptoms in patients with AD. Pre-clinical and clinical studies carried out over the last 5 years have reported that polyphenols from olive oil and wine are active components which exert a noteworthy neuroprotective effect. These polyphenols reduce the Aβ aggregation and tau-protein hyperphosphorylation, promoting autophagy and improving the damaged mitochondrial function and cerebral insulin resistance related to AD. Based on this evidence, it can be concluded that intake of polyphenols from EVOO and or wine could be a great therapeutic tool to prevent or delay the neurodegeneration present in AD. However, some limitations must be considered in future studies. Defining the bioavailability of polyphenols clearly would help to describe action mechanisms of these molecules because current data are still limited.
Another unclear issue is whether the polyphenols from daily consumption olive oil and wine are enough or, conversely, whether a nutritional supplement enriched with polyphenols would be more efficient to exert their neuroprotective effects. To elucidate this limitation, interventional trials with an accurate dose of polyphenols should be carried out. In addition, we must take into account that recruiting a homogeneous cohort of patients with the same degree of cognitive decline is very difficult.
Therefore, more longitudinal studies in humans are necessary to corroborate the efficacy of these nutraceuticals, as well as determine the most appropriate dose for this purpose. In summary, with this review we would like to highlight that, due to their polyphenol content, moderate consumption of wine and olive oil could be beneficial for our brain.
Financial support
This research received no specific grant from any funding agency, commercial or not-for-profit sectors.
Conflict of Interest
None.
Authorship
P.S. and E.B.-R. designed and planned the manuscript. M.R.-P., O.G.-T. and E.B.-R. wrote the sections on olive oil and P.S. those on wine. P.S. designed the figures and E.B.-R. the table. All authors provided critical feedback, helped shape the work and approved the submission of the final version.
Abbreviations
- AD
-
= Alzheimer disease
- AICD
-
= amyloid protein precursor intracellular domain
- AMPK
-
= adenosine monophosphate-activated protein kinase
- APP
-
= amyloid protein precursor
- ATG
-
= autophagy-related protein 13/101
- Aβ
-
= amyloid beta peptide
- BACE-1
-
= β-site amyloid precursor protein cleaving enzyme-1;
- BBB
-
= blood–brain barrier
- BECN
-
= human beclin 1 gene
- CaMKII
-
= calmodulin-dependent protein kinase II
- CaMKK β
-
= calcium/calmodulin-dependent protein kinase kinase β
- CDK5
-
= cyclin-dependent kinase 5
- CSF
-
= cerebrospinal fluid
- ER
-
= endoplasmic reticulum
- ERK
-
= extracellular signal-regulated kinases
- EVOO
-
= extra virgin olive oil
- GLUT1
-
= glucose transporter type 1
- GPx
-
= glutathione peroxidase
- GSH
-
= glutathione
- GSK3β
-
= glycogen synthase kinase 3β
- HT
-
= hydroxytyrosol
- HT22
-
= murine hippocampal neuronal cell line
- IRS1
-
= insulin-receptor substrate 1
- LC3
-
= microtubule-associated protein 1A/1B-light chain 3
- MID1
-
= microtubule-associated ubiquitin ligase
- mTOR
-
= mammalian target of rapamycin
- mTORC1
-
= mammalian target of rapamycin complex 1
- MUFAs
-
= monounsaturated fatty acids
- N2a
-
= mouse neuroblastoma cell line
- NF-κB
-
= nuclear factor-kappa beta
- NFTs
-
= neurofibrillary tangles
- NLRP3
-
= Nod-like receptor family pyrin domain containing 3
- p-Akt
-
= phospho-protein kinase B
- PARP1
-
= poly(ADP-ribose) polymerase I
- PC12
-
= phaeochromocytoma cells
- PGC1α
-
= peroxisome proliferator-activated receptor gamma coactivator 1α
- p-GSK3β
-
= Phospho-glycogen synthase kinase 3 beta
- PI3K
-
= phosphatidylinositol-3 kinase
- PP2A
-
= protein phosphatase 2 A
- PS1
-
= presenilin-1
- PUFAs
-
= polyunsaturated fatty acids
- SAMP8
-
= senescence-accelerated mouse
- SH-SY5Y
-
= human neuroblastoma cells
- SIRT1
-
= sirtuin-1
- TRPM2
-
= transient receptor potential melastatin 2
- ULK1
-
= unc-51-like kinase 1