Resonant soft x-ray scattering (RSoXS) is a relatively new technique that can provide structural information about organic thin films with chemical sensitivity. Pioneering work demonstrated that transmission soft x-ray scattering experiments can reveal the 20 to 30 nm domain spacing in microphase-separated polystyrene-b-polyisoprene thin films by working at resonance with the 1s →π*(C = C) transition.[Reference Virgili, Tao, Kortright, Balsara and Segalman1] Further work has transformed our understanding of the microstructure in polymer/fullerene mixtures[Reference Tumbleston, Collins, Yang, Stuart, Gann, Ma, You and Ade2–Reference Swaraj, Wang, Yan, Watts, Jan, McNeill and Ade5] and conjugated block copolymers (BCPs) used in photovoltaics[Reference Guo, Lin, Witman, Smith, Wang, Hexemer, Strzalka, Gomez and Verduzco6] by identifying length scales for phase or microphase separation, and has resulted in new structural models for biomolecules in solution, such as casein micelles[Reference Ingham, Erlangga, Smialowska, Kirby, Wang, Matia-Merino, Haverkamp and Carr7, Reference Ingham, Smialowska, Erlangga, Matia-Merino, Kirby, Wang, Haverkamp and Carr8] and bovine serum albumin.[Reference Ye, Le, Kuei, Zhu, Zwart, Wang, Gomez and Gomez9] Most RSoXS data to date are generated at the carbon edge,[Reference Gann, Young, Collins, Yan, Nasiatka, Padmore, Ade, Hexemer and Wang10] with a few exceptions of studies that include the oxygen[Reference Guo, Kozub, Kesava, Wang, Hexemer and Gomez11, Reference Kortright, Sun, Spencer, Jiang and Zuckermann12] and calcium edges.[Reference Ingham, Erlangga, Smialowska, Kirby, Wang, Matia-Merino, Haverkamp and Carr7, Reference Ye, Kiemle, Rongpipi, Wang, Wang, Cosgrove, Gomez and Gomez13]
Despite the opportunity to examine the distribution of nitrogen (N) in organic and biological compounds, very few studies have employed RSoXS at the N edge.[Reference Brady, Ku, Perez, Cochran, Schmidt, Weiss, Toney, Ade, Hexemer, Wang, Hawker, Kramer and Chabinyc14] The challenge lies in the x-ray transparent support, which is 50–100 nm thick Si3N4 that produces a significant RSoXS background at energies near the N edge. These Si3N4 supports are formed through a low-pressure chemical vapor deposition (LPCVD) process and have Young's moduli between 140 and 290 GPa[Reference Khan, Philip and Hess15] with a fracture toughness of about 6 MPa·m0.5.[Reference Merle and Göken16] These mechanical properties allow fabricated free-standing Si3N4 windows with a 1 mm × 1 mm opening to have a thickness of approximately 50 nm and enable the construction of liquid sample cells that can withstand the high vacuum in the RSoXS sample chamber.[Reference Ingham, Erlangga, Smialowska, Kirby, Wang, Matia-Merino, Haverkamp and Carr7, Reference Ye, Le, Kuei, Zhu, Zwart, Wang, Gomez and Gomez9] Nevertheless, fluorescence at the N edge when using Si3N4 supports adds a significant incoherent background and confounds RSoXS studies.
As an alternative to Si3N4, we designed and fabricated Al2O3 windows for RSoXS experiments. Atomic layer deposition (ALD) leads to Al2O3 films with Young's moduli near 200 GPa[Reference Tripp, Stampfer, Miller, Helbling, Herrmann, Hierold, Gall, George and Bright17] and a fracture toughness of about 2 MPa·m0.5,[Reference Miller, Foster, Zhang, Jen, Bertrand, Lu, Seghete, O'Patchen, Yang, Lee, George and Dunn18] which is comparable with values from LPCVD Si3N4 films. These mechanical properties enabled the fabrication of 46 nm thick free-standing Al2O3 films that have higher x-ray transmittance than commercially available 50 nm thick Si3N4. Using Al2O3 films as x-ray windows, we demonstrated RSoXS at the N K-edge for a poly(3-hexylthiophene-2,5-diyl)-block-poly((9,9-bis-(2-octyl) fluorene-2,7-diyl)-alt-(4,7-di(thiophene-2-yl)-21,3-benzothiadiazole)-5’,5”-diyl) BCP (P3HT-b-PFTBT). RSoXS of P3HT-b-PFTBT supported on Al2O3 windows reveals a structural feature at the N K-edge that is not observed using Si3N4 supports due to the fluorescence background from Si3N4.
Suitable substrates for soft x-ray scattering experiments require a x-ray transparent film and a support structure. Our approach relies on patterning Al2O3 films that are deposited on silicon wafers as shown in Fig. 1. The RSoXS substrate design contains 5 mm × 5 mm squares as the silicon frame with a 1.7 mm-diameter circle in the center of each square as the Al2O3 window. Al2O3 films were grown on a 4-inch silicon wafer through ALD and patterns were transferred to the Al2O3-coated wafer through photolithography. After photolithography, the silicon covering the circular patterned area was back etched using deep reactive-ion etching, which leaves an Al2O3 free-standing film with a diameter of approximately 1 mm. Following the nanofabrication process, resist was removed and the wafers were cleaved into individual Al2O3 windows. Spectroscopic ellipsometry revealed an Al2O3 window thickness of 46 nm. AFM images shown in Figure S1 of the Supplementary material reveal that the roughness of Al2O3 films is similar to the roughness of commercially available Si3N4 films. Nevertheless, shallow long depressions (trenches) are apparent in Al2O3, although the origin of these features is unclear.

Figure 1. Fabrication of Al2O3 windows by atomic layer deposition and photolithography. (a) Schematic of Al2O3 window fabrication process. (b) A 4-inch wafer after lithography and etch. (c) An Al2O3 window used as a support for RSoXS experiments.
The x-ray transmittance and scattering of Al2O3 windows as compared with Si3N4 windows were tested using RSoXS. As shown in Fig. 2(a), the x-ray transmittance spectra indicate that the 46 nm thick Al2O3 windows absorb less x-rays than the 50 nm thick Si3N4 windows. The scattering profile from the Al2O3 film has no structural peaks near the carbon K-edge [Fig. 2(b)] or the nitrogen K-edge [Fig. 2(c)], as expected. Data at the onset of the nitrogen K-edge (398.2 eV) is shown in Figure S2 of the Supplementary material. Scattering from the 46 nm thick Al2O3 window has lower intensity at high q (q > 0.01 Å−1) than scattering from the 50 nm thick Si3N4 window. The higher scattering intensity from Al2O3 at low q (q < 0.01 Å−1) could arise because the scattering contrast between Al2O3 and vacuum is higher than the scattering contrast between Si3N4 and vacuum (Figure S3 of the Supplementary material), such that any surface roughness of the Al2O3 window contributes more strongly to scattering. Alternatively, the Al2O3 windows may have more residual contaminants on their surfaces left from the photolithography process, or the long shallow trenches apparent throughout the samples (Figure S1 of the Supplementary material) could contribute to scattering at low q.
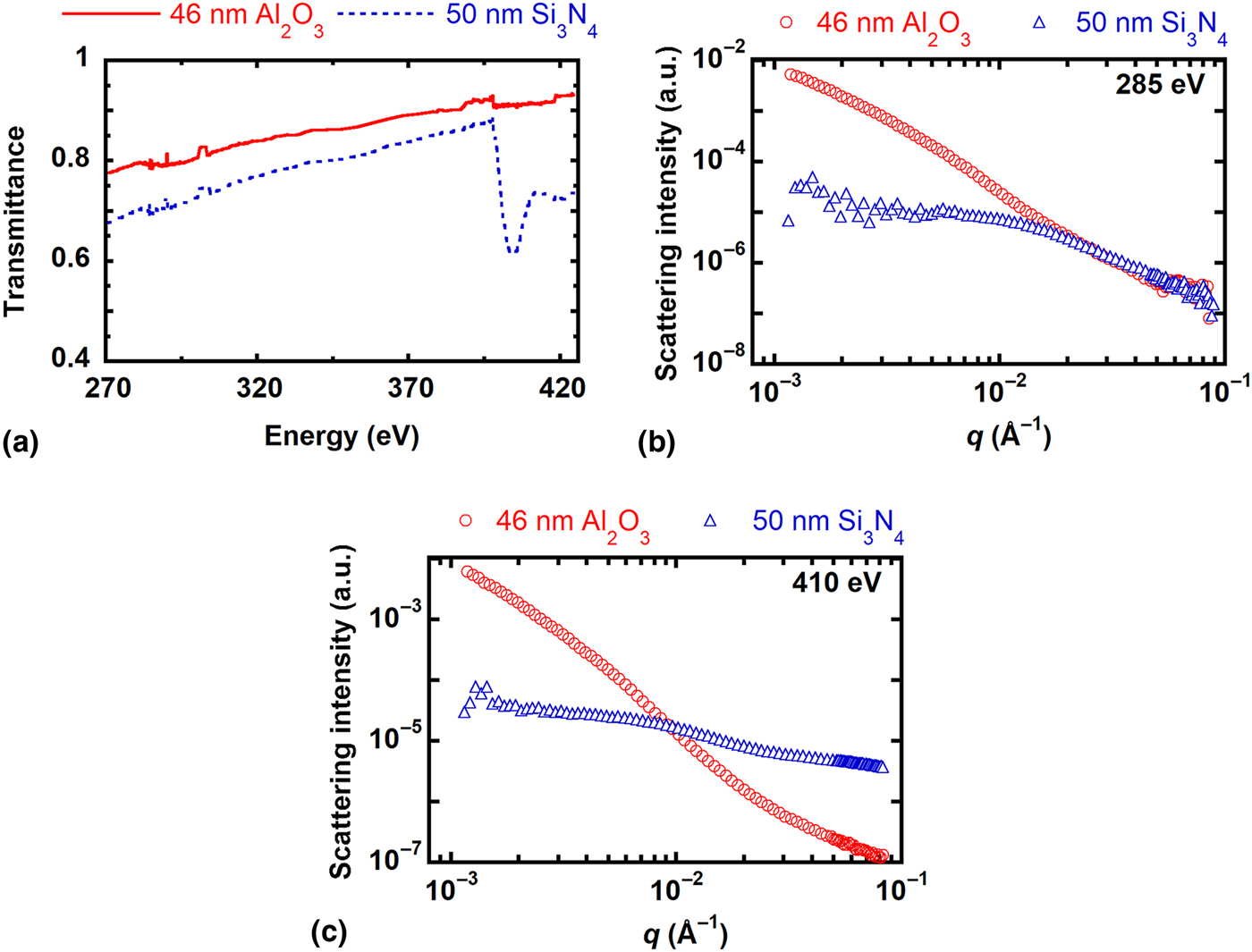
Figure 2. Absorption and scattering profiles of 46 nm thick Al2O3 and 50 nm thick Si3N4 substrates. (a) NEXAFS spectra near the carbon and nitrogen K-edges. Scattering profiles at (b) 285 eV (carbon K-edge) and (c) 410 eV (nitrogen K-edge).
A challenge presented by commercially available Si3N4 windows traditionally used for RSoXS studies is that they generate a high incoherent fluorescence background. X-ray emission that follows ionization generates a q independent constant in the scattering profile. Using a simple model, the influence of fluorescence background on scattering profiles is demonstrated in Figure S4 of the Supplementary material. As the fluorescence background increases, the I(q)q 2 profile starts to have a more pronounced upturn in the high q region (q > 0.02 Å−1) that can mask structural features of the sample. As shown in Figure S5a of the Supplementary material, Al2O3 has a similar scattering profile at the C K-edge and the N K-edge with no significant upturn. On the other hand, the scattering profile of Si3N4 becomes nearly constant above q = 0.01 Å−1 at the C K-edge while there is an upturn near q = 0.02 Å−1 at the N K-edge (Figure S5b of the Supplementary material). The upturn is attributed to fluorescence emission from N atoms in Si3N4. Thus, the Al2O3 windows offer a clear advantage over Si3N4 at the N edge for RSoXS studies.
We use P3HT-b-PFTBT as a model system to demonstrate the utility of Al2O3 windows for characterizing the morphology of nitrogen-containing materials. P3HT-b-PFTBT is a conjugated BCP with record performance as a single-component active layer in organic solar cell devices.[Reference Guo, Lin, Witman, Smith, Wang, Hexemer, Strzalka, Gomez and Verduzco6] Previous work has demonstrated that P3HT-b-PFTBT microphase separates into a lamellar morphology with a domain spacing of about 20 nm that depends on the molecular weight.[Reference Guo, Lin, Witman, Smith, Wang, Hexemer, Strzalka, Gomez and Verduzco6, Reference Guo, Lee, Lin, Strzalka, Wang, Hexemer, Jaye, Fischer, Verduzco, Wang and Gomez19, Reference Grieco, Aplan, Rimshaw, Lee, Le, Zhang, Wang, Milner, Gomez and Asbury20] P3HT-b-PFTBT was synthesized using previously published methods.[Reference Lee, Aplan, Seibers, Kilbey, Wang and Gomez21] As shown in Fig. 3(a), there are two nitrogen atoms per monomer in the PFTBT block. The NEXAFS spectra of the homopolymers (P3HT and PFTBT) and the BCP (P3HT-b-PFTBT) are plotted in Fig. 3(b). All three polymers have strong absorption near the carbon K-edge, and the sharp peak near 285 eV corresponds to the 1s→π* transitions of the C = C bonds. Near the nitrogen edge (380 to 420 eV), there is no feature in the P3HT NEXAFS spectra while there are absorption features in the PFTBT and P3HT-b-PFTBT spectra from the nitrogen atoms of PFTBT. The absorption is less apparent in P3HT-b-PFTBT because the number density of nitrogen is lower than it is in PFTBT. Nevertheless, the difference in NEXAFS spectra between P3HT and PFTBT due to the lack of nitrogen in P3HT should lead to significant RSoXS contrast at the N edge.
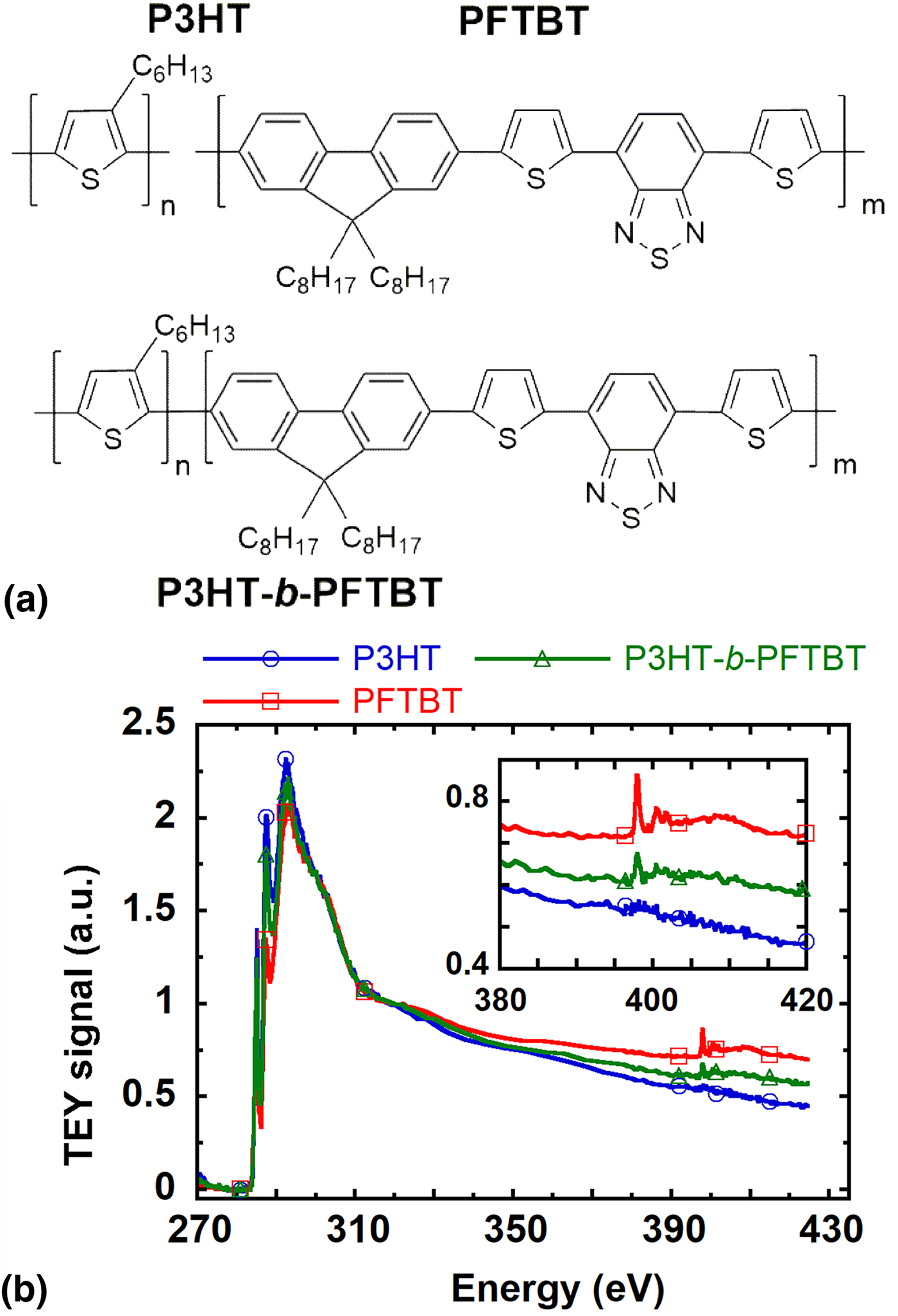
Figure 3. A model system for N RSoXS experiments. (a) Chemical structures and (b) Total Electron Yield (TEY) NEXAFS spectra of P3HT, PFTBT, and P3HT-b-PFTBT. The inset highlights spectra near the N edge.
Scattering profiles of P3HT-b-PFTBT thin films supported on Al2O3 windows and supported on commercially available Si3N4 windows are presented in Fig. 4. The background scattering from Al2O3 or Si3N4 windows was subtracted from the data. As shown in Fig. 4(a), the scattering profiles near the carbon K-edge (285 eV) of P3HT-b-PFTBT supported on Al2O3 or Si3N4 are very similar. The structural feature near q = 0.03 Å−1 corresponds to 20 nm and indicates that P3HT and PFTBT have distinct nanoscale domains that are separated by 20 nm on average. This feature near q = 0.03 Å−1 becomes more apparent in the Kratky plot (Iq 2 versus q) shown in Fig. 4(b), although an overall positive slope for the entire profile that is likely indicative of fluorescence from the polymer film is apparent.

Figure 4. RSoXS profiles and Kratky plots of scattering from a P3HT-b-PFTBT block copolymer (BCP) supported on a 46 nm thick Al2O3 substrate or a 50 nm thick Si3N4 substrate near the (a), (b) carbon K-edge (285 eV) and the (c), (d) nitrogen K-edge (410 eV).
Near the nitrogen edge (410 eV), there is a clear feature at approximately q = 0.02 Å−1 corresponding to about 30 nm in the scattering profile of P3HT-b-PFTBT supported on Al2O3 windows that is not present when measurements are taken using Si3N4 windows [Fig. 4(c)]. Data are also shown at the onset of the nitrogen edge (398.2 eV) in Figure S6 of the Supplementary material. When Si3N4 is used as a substrate, the background corrected Kratky plot has an overall positive slope with an upturn at high q starting from q = 0.03 Å−1, as shown in Fig. 4(d). The upturn at high q in Iq 2 is often a signature of a fluorescence (q-independent) background. Furthermore, when Si3N4 windows are used, the scattering profile at 285 eV reveals peaks associated with the microstructure of P3HT-b-PFTBT [Figs. 4(a) and 4(b)], but these peaks are not apparent at the N edge [Figs. 4(c) and 4(d)]. We thus speculate that scattering is re-absorbed and emitted as incoherent fluorescence when Si3N4 windows are used at the N edge.
When comparing the data collected at the carbon and nitrogen edge from BCP films with Al2O3 supports, there is a disagreement in terms of the apparent domain spacing (at q = 0.03 and 0.02 Å−1, respectively). Near the nitrogen edge, the scattering contrast is between P3HT and PFTBT domains, but near the carbon edge, the scattering contrast is more complicated. Because RSoXS is sensitive to differences in chain orientation and the 1s→π* transition dipole moment is anisotropic, we speculate that the structure between crystalline and amorphous P3HT leads to scattering peaks at a higher q.[Reference Vakhshouri, Smith, Chan, Wang, Salleo, Wang, Hexemer and Gomez22, Reference Collins, Cochran, Yan, Gann, Hub, Fink, Wang, Schuettfort, McNeill, Chabinyc and Ade23] Thus, the combination of C and N RSoXS reveals multiple aspects of the microstructure of P3HT-b-PFTBT films, including a center-to-center spacing of crystallites within P3HT domains of 20 nm and a spacing between P3HT and PFTBT domains of 30 nm.
In summary, we demonstrate that free standing 46 nm thick Al2O3 films enable examination of the structure of nitrogen-containing materials using RSoXS. Al2O3 windows reduce parasitic absorption and eliminate the fluorescence background near the nitrogen edge. As a consequence, structural features of P3HT-b-PFTBT are revealed near the nitrogen edge on Al2O3 substrates, even though such features are not apparent using commercial Si3N4 substrates. Fabricating x-ray transparent substrates, such as Al2O3 films, will enable RSoXS studies focused on nitrogen-containing systems that include some organic semiconductors, membrane proteins, and protein-nucleic acids complexes.
Supplementary material
The supplementary material for this article can be found at https://doi.org/10.1557/mrc.2018.195.
Acknowledgments
Financial support from NSF MRI-1626566 (E.D.G.) and the Grace Woodward Grant for Collaborative Research in Engineering and Medicine (E.W.G.) is acknowledged. D.Y. acknowledges support by an Advanced Light Source Doctoral Fellowship in Residence. The Advanced Light Source is supported by the Director, Office of Science, Office of Basic Energy Sciences, of the US Department of Energy under Contract No. DE-AC02-05CH11231. The authors thank Chad Eichfeld and Guy Lavallee for their help with the design of the lithography mask and etch process.