Lung function measurement is important to diagnose lung diseases and to suggest preventive attention(Reference Flesch and Dine1). Malnourished children are prone to frequent and severe episodes of respiratory illnesses(Reference Rodríguez, Cervantes and Ortiz2). Immuno-programming during the fetal period and early infancy has been reported to be linked with the development of asthma and other respiratory illnesses in later life(Reference Stocks, Hislop and Sonnappa3). Many of these children experience fewer clinical features of respiratory illnesses with growing age, yet others continue to have asthma that persists even in adult life(Reference Morishita, Strufaldi and Puccini4). Moreover, fetal, postnatal and early-childhood nutrition and growth distortion can permanently alter the structure and function of the adult lung because these are critical periods of rapid growth and development of the respiratory system(Reference Harding and Maritz5,Reference Dezateux and Stocks6) . Lung size increases with body size after birth, and alveoli continue to increase in number, size and complexity with a simultaneous increase in gas-exchanging surface area from adolescence to adulthood(Reference Stocks and Sonnappa7).
Respiratory infection is the leading cause of death among children under 5 years of age, with a rate of 22 % of all under-5 deaths in Bangladesh(Reference Halder, Gurley and Naheed8). Each year, nearly 80 000 children under 5 years of age are admitted to hospital due to respiratory infections; however, the overall infection rate is assumed to be much higher(Reference Lozano, Naghavi and Foreman9). Chronic undernutrition is common in Bangladesh and is reflected best by stunting and low height-for-age z-score (HAZ)(Reference Saha, Chattapadhayay and Richardus10). Stunting has been reported to be independently associated with childhood asthma and other respiratory illnesses since height is a major determinant of lung size and pulmonary function(Reference Hawlader, Noguchi and El Arifeen11). Former studies have observed the relationship between birth weight and lung function, wherein some studies have reported on the impact of fetal growth restriction and the role of catch-up growth by BMI or BMI trends through early life on adult lung functions(Reference Peterson, Jacobs and Milla12–Reference Suresh, O’Callaghan and Sly15). So far, the relationship between growth trend by height-for-age during childhood and lung function, and how this growth trend impacts lung function at preadolescence are not known. Additionally, tracking longitudinal measurements of growth by height-for-age may reveal differences in lung function in children who had catch-up growth at preadolescence, which has been hypothesised as a crucial period of development but not yet tested and established. The long follow-up offers the opportunity to examine the growth trajectory through longitudinal measurements in a large cohort of children, and to determine the demographic and clinical factors associated with the lung function. Moreover, the lung function of Bangladeshi children with persistent stunting (PS) episodes from the postnatal period to childhood has not yet been evaluated. Among Bangladeshi adults (>40 years), the prevalence of chronic obstructive pulmonary disease (COPD) is 13·5 % according to a hospital-based study, which is also an unacceptably underdiagnosed public health problem in Bangladesh(Reference Alam, Chowdhury and Siddiquee16). Respiratory diseases are an epidemic in Bangladesh; however, most are not well aware to the diseases involved. Thus, it is important to assess the effects of inadequate growth by height-for-age on lung function at preadolescent age so that appropriate intervention could be implemented to halt or slow down pulmonary disease progression. The aim of the current study was to understand the association between early-childhood chronic undernutrition trajectory and lung function at preadolescence.
Materials and methods
Study design
The current observational study included an immune cohort that was nested in a 9-year follow-up of a large, randomised, population-based food and micronutrient supplementation trial among pregnant women, known as the Maternal and Infant Nutrition Interventions in Matlab (MINIMat) (trial registration: isrctn.org; identifier: ISRCTN16581394). The MINIMat immune cohort estimated child immune function and morbidity, blood pressure, metabolic markers, etc. The primary objective of the MINIMat study was to improve maternal health and birth size in Matlab, a sub-district in rural Bangladesh, through micronutrient and food supplementation. The details of the MINIMat cohort, study methodology and the primary outcomes have been published earlier(Reference Persson, Arifeen and Ekström17–Reference Arifeen, Ekström and Frongillo19).
Study participants
In the MINIMat study, a total of 4436 pregnant women between 6 and 8 weeks of pregnancy were enrolled from November 2001 to October 2003; and out of these, there were 3625 singleton live births. The MINIMat study and the Health and Demographic Surveillance System in the area closely monitored these children. A female co-medical staff member based in the sub-centre visited the mothers within 72 h of birth to measure weight, height, head circumference and knee-heel length of the newborn. Mothers and newborn children were assessed at birth, followed by monthly examinations of the dyads up to 12 months. During the second year of life, the children were assessed quarterly. The subsequent follow-ups were performed at 4·5 and 9 years of age. Information on each woman’s age, parity, education and household assets was collected from the surveillance system and through interviews with the women. Parity refers to the number of live or dead children delivered before the current pregnancy. Economic status was assessed by generating scores through a principal component analysis based on household assets, housing structure, land occupation and income.
A comprehensive follow-up of children born into the MINIMat trial was conducted between May 2007 and February 2009 when they were 4·5 years of age. The 3267 children who were live singleton births and had their birth anthropometry measured were eligible for the follow-up study. Informed consent was obtained from their parents or guardians. Of all eligible children, 2735 were tracked and followed up at 4·5 years of age. A range of outcomes was assessed during the follow-up, including child growth and body composition. For cost and logistic considerations, the children were divided into two groups on the basis of their year of birth (group A, April 2002–June 2003; group B, June 2003–June 2004). In group B, there were 1303 children who participated in the 4·5-year follow-up from December 2007 to February 2009.
Among group B MINIMat children, 640 were invited for spirometry test at 9 years of age. Out of these, forty-two children had migrated out from the study area, eight children were absent, and another thirty-nine children’s parents did not give consent. Finally, 551 children participated in the follow-up study at 9 years of age; of these, six refused to participate and five were unable to successfully undergo the respiratory test. A further twenty-three children were excluded due to a lack of data on birth weight and height measurements (collected beyond 72 h after birth). Therefore, a total of 517 children constituted the sample size for the analysis (Fig. 1).
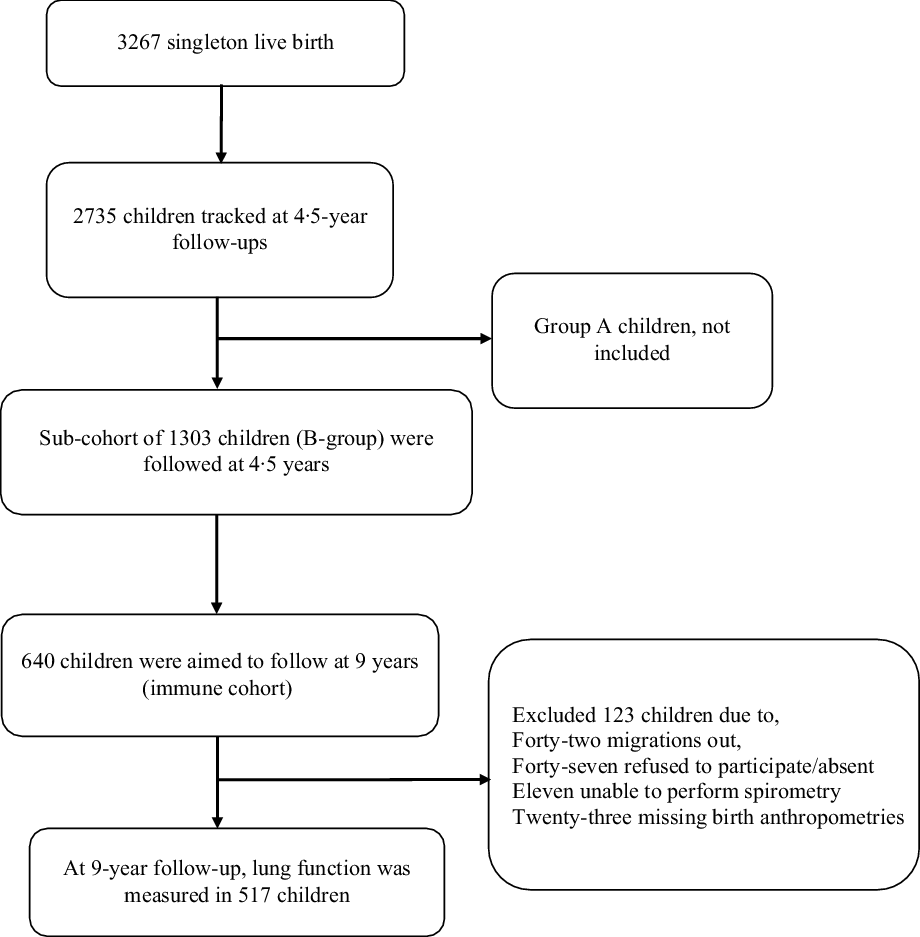
Fig. 1 Study participants
Anthropometry and chronic malnutrition
Maternal height and weight were measured during enrolment of the main MINIMat cohort at their 6–13 weeks of gestation. Maternal weight was measured using an electronic scale (Seca) with a precision of 100 g, and height was measured using locally made wooden scales with a precision of 0·1 cm. Weight and height of all the MINIMat children were measured by trained field staff from birth to all follow-up visits. The attending nurse performed anthropometry when deliveries took place at health facilities. The MINIMat cohort had established a birth notification system for those women who delivered at home. Trained co-medical staff members measured birth anthropometry mostly within 72 h of birth. Birth weight was measured using an electronic scale (Seca) with a precision of 10 g. Birth weight measurements taken within the first 24 h were used without adjustment. Measurements taken beyond 24 h to 30 d after birth were adjusted using a standard deviation score transformation with the assumption that the birth weight tends to remain the same(Reference Arifeen, Black and Caulfield20). At 4·5 and 9 years of follow-up, children’s weights were measured using an electronic scale (Tanita Corporation) with a precision of 0·1 kg, and height with a standing stadiometer (Holtain) with a precision of 0·1 cm.
For the current analysis, we included children whose data were available for all five follow-up visits (at 6 months, 1, 2, 4·5 and 9 years) of the MINIMat study and had data on birth anthropometry. Stunting status (HAZ <–2) of each child was calculated using the WHO Anthro software at each age. Children who remained stunted repeatedly at five follow-up visits were considered as persistently stunted (PS); those who were stunted at a minimum of 1 time point (periodic stunting) of these five follow-up visits (<5 points) were considered as intermittently stunted (IS); and those who never experienced stunting in any of these five follow-up visits were considered as of normal stature (NS). Small for gestational age (SGA) was defined if the birth weight was within <10 percentile values of the WHO reference(Reference Kiserud, Piaggio and Carroli21). During enrolment, maternal height and weight were measured at 6–13 weeks of gestation, and BMI was calculated using this information.
Measurement of child growth trajectory
The recorded birth weight and height were standardised with gestational age at birth and converted to z-scores. Weight and height at five follow-up visits were also converted to z-scores. The primary exposure in the current study was postnatal height gain that was respective for the age according to the WHO growth standard. Catch-up growth, also known as compensatory growth, was considered when a child returned to his genetic trajectory for size after a period of growth delay or growth arrest(Reference Ong, Ahmed and Emmett22). In the current study, the postnatal catch-up growth was defined as changes in standard deviation scores for HAZ among children who experienced stunting between 6 months and 9 years at various time (shorter) periods (6 months–1 year, 6 months–2 years, 6 months–4·5 years). The gain in HAZ between 6 months and 9 years that was >0·67 sd scores was considered to indicate clinically considerable catch-up in growth. A 0·67 sd score represented the width of each percentile band on standard growth charts, which follows 2nd to 9th percentile, 9th to 25th percentile, 25th to 50th percentile and so on. Similarly, a decline in HAZ of 0·67 sd score indicated growth faltering. Above and beyond, the impact of the speed of catch-up growth (by HAZ growth trajectory) on the state of lung function was also checked. The speed of catch-up growth was defined as changes in standard deviation scores for HAZ between 6 months and 1 year, 1–2 years, 2–4·5 years and 4·5–9 years.
Spirometry
Lung function test (forced vital capacity, FVC; forced expiratory volume per second, FEV1·0; maximum mid-expiratory flow, MMF; and peak expiratory flow, PEF) using a spirometer was measured at the age of 9 years. Children’s lung function was assessed using an electronic spirometer (Chestgraph HI-101; CHEST Ltd) in accordance with the American Thoracic Society (ATS) recommendation (American Thoracic Society 1995: three best measurements were recorded in the recording form). To minimise diurnal variation, lung function was measured in the morning time (09.00–11.00 hours). Children were examined by a general physician prior to spirometry tests and were found to be healthy, without any history of immune-related diseases such as allergic disorders, cancer, type 1 diabetes, etc. A trained technician explained the process in detail to the children and allowed them to exercise until they felt comfortable. Children were instructed to rest for at least 10 min after arrival at the sub-centres. The lung function was measured in a standing position using a nose clip and a disposable mouthpiece. Children were instructed to breathe in as deeply as possible, following the demonstration and practice, and then blow as hard and long as possible into the spirometer. Children were requested to perform repeated FVC manoeuvers, at least three times, and up to a maximum of eight times or until satisfactory results were achieved (FVC and FEV1·0) agreeing within 150 ml and forced expiratory time exceeding 6 s according to the ATS criteria (American Thoracic Society, 1995). The research team also visually assessed the curves for smoothness and any broken or double lines that might occur due to a cough or any hesitation. The best trial of each child (maximum sum of FEV1·0 and FVC) was included in the analysis. For the current analysis, sex and child age (age (months) at 9-year follow-up) standardised mean values of each lung function indicator were calculated from raw values.
Data analysis
Numeric data were expressed as mean and sd and compared by independent t test or Mann–Whitney test or Kruskal–Wallis test or post-hoc Bonferroni test. Categorical data were presented as numbers and compared using χ 2 test. Child growth trajectory by HAZ (from 6 months to 9 years) was the primary exposure of interest because, according to the WHO malnutrition and growth concept, in the first several years of life a child experiences the most devastating outcome of disorder in terms of mortality and morbidity; these are related with later growth and development(Reference Lutter, Peña-Rosas and Pérez-Escamilla23). The values of each indicator of lung function (FVC, FEV1·0, FEV1·0/FVC ratio, MMF and PEF) were the outcome measures. For the current study, two mean differences were estimated by independent t test, and three or more groups’ mean differences were estimated by ANOVA if the distribution was normal. For skewed distribution, the Kruskal–Wallis test was performed to check the mean differences among groups. Post-hoc Bonferroni test was performed to check groupwise mean differences in lung function among all growth trajectory groups, that is, mean lung function differences between NS group and unaltered growth velocity group, or mean differences between catch-up group and unaltered growth velocity group, etc. Generalised linear regression models were employed to determine the effect of catch-up growth on lung function indices of children at 9 years. The covariates included in the growth velocity model were parity (nulliparous or multiparous), maternal age at pregnancy, maternal height and birth weight of children standardised with gestational age at birth, history of asthma (yes or no), family history of asthma (yes or no) and reported episode of dry cough in the past 6 months (yes or no). Covariates selected in the regression models were based on known risk factors. Analyses were conducted using the statistical software package IBM SPSS Statistics version 23, and statistical significance (two-sided) was considered at P-value <0·05.
Results
The mean age of children at the measurement of lung function was 8·9 (sd 0·1) years, and 48·2 % were male. The mean birth gestational week was 39·2 (sd 1·5), and 5·4 % of children were born preterm (born before 37 weeks of gestation). The mean birth weight was 2755·9 (sd 392·9) g, and 23·6 % of children were of low birth weight (LBW; <2500 g). Among the study children, 80·7 % (n 417) were SGA. Of these, 29·9 % (n 122) were LBW, and 6·5 % (n 27) were preterm at birth. A total of 24·1 % (n 114) children at 6 months, 32·1 % (n 157) at 1 year, 47·3 % (n 223) at 2 years, 28·6 % (n 148) at 4·5 years and 28·6 % (n 148) at 9 years of age were stunted. During all the five follow-up visits, 8·3 % (n 43) of children had PS, 46·6 % (n 241) had IS, and the rest 45·1 % (n 233) had NS. Of children who ever experienced stunting (n 265), 21·9 % (n 58/265) demonstrated catch-up growth at 9 years. Among the PS children (n 43), only seven (16·3 %) had catch-up growth, sixteen (37·2 %) reported growth faltering, and twenty (46·5 %) remained in the same growth velocity.
Growth trajectory by height-for-age z-score
The growth of 452 children was followed from the age of 6 months to 1 year. Of these children, ten (2·2 %) had catch-up growth, eighty-two (18·1 %) showed growth faltering, 161 (35·6 %) remained in the same growth velocity among those who ever experienced stunting, with 199 (44·0 %) children having normal growth (HAZ ≥2·0) in both follow-ups. Among stunted children, nine (2·1 %) demonstrated catch-up growth, 135 (31·2 %) showed growth faltering, while 103 (23·8 %) remained in the same growth velocity, and the rest 186 (43·0 %) had normal growth from 6 months to 2 years of age (n 433). The growth of 472 children was tracked from the age of 6 months to 4·5 years. Of the stunted group, twenty-nine (6·1 %) had catch-up growth, seventy-nine (16·7 %) reported growth faltering, with 157 (33·3 %) maintaining the same growth velocity and 208 (44·0 %) remaining in the NS status. In total, 473 children were followed from the age of 6 months to 9 years. Of those with a stunted stature, fifty-eight (12·3 %) showed catch-up growth, fifty-seven (12·1 %) demonstrated growth faltering, with 150 (31·7 %) remaining in the same growth velocity, while 208 (44·0 %) had NS status (Fig. 2).

Fig. 2 Distribution of growth trajectories in different follow-up periods. , catch-up;
, unaltered;
, slow-down of faltering;
, normal
A higher proportion of male, preterm and LBW children showed catch-up growth in comparison to female, at-term and normal birth weight (NBW) children, respectively (Table 1). The mean z-scores of birth weight and height were lower in children who showed catch-up growth than those with growth faltering or remaining in the same growth velocity or in the NS group. Other anthropometric mean differences among groups at different follow-up visits are given in Table 2.
Table 1 Characteristics of study children by height-for-age growth trajectory (6 months–9 years)

* Differences within groups estimated by χ 2 test and mean differences estimated by one-way ANOVA (Mann–Whitney U test).
Table 2 Children’s growth (z-score) at five follow-up visits by height-for-age growth trajectory (6 months–9 years)

* The Kruskal–Wallis test.
Boys showed higher lung function values compared with girls (Table 3). The average lung function (FVC/ml, FEV1·0/ml per s, PEF/ml per s) was lower in children who were LBW than those who were NBW (Table 4). The mean values of FVC/ml and FEV1·0/ml per s were lower in PS and IS children than in NS children (Table 5).
Table 3 Means of lung function indicators by child’s sex

FVC, forced vital capacity; FEV1·0, forced expiratory volume per second; MMF, maximum mid-expiratory flow; PEF, peak expiratory flow.
* The independent t test.
Table 4 Means of lung function indicators by child’s birth weight status and term of birth

LBW, low birth weight; NBW, normal birth weight; FVC, forced vital capacity; FEV1·0, forced expiratory volume per second; MMF, maximum mid-expiratory flow; PEF, peak expiratory flow.
* Mean values of lung function were standardised with sex and child’s age (months) at 9-year follow-up.
† The independent t test.
Table 5 Means of lung function indicators by chronic malnutrition trajectory

FVC, forced vital capacity; FEV1·0, forced expiratory volume per second; MMF, maximum mid-expiratory flow; PEF, peak expiratory flow.
* Mean values of lung function were standardised with sex and child’s age (months) at 9-year follow-up.
† The Mann–Whitney U test.
Growth trajectory by height-or-age with lung function
In general, significant mean differences in FVC/ml, FEV1·0/ml per s, MMF/ml per s and PEF/ml per s values were observed within the four comparison groups of children who had NS throughout the study period, and who showed catch-up growth, who remained in the same growth velocity, as well as those who had growth faltering from the stunted group (at different follow-up visits) (P < 0·05). The FVC/ml, FEV1·0/ml per s, MMF/ml per s and PEF/ml per s values were also higher in stunted children who had catch-up growth from 6 months to 4·5 years than did children who showed faltering growth velocity (P < 0·05). On the other hand, in children who showed catch-up growth from 6 months to 9 years, their FVC/ml and MMF/ml per s values were also higher than for children who remained in the same growth velocity, and accordingly, their PEF/ml per s values were also higher than that of children who had faltered growth. In contrast, the mean values of FVC/ml, FEV1·0/ml per s, MMF/ml per s and PEF/ml per s were identical between children who had NS and who showed catch-up growth from the stunted group (Table 6).
Table 6 Means of lung function indicators by height-for-age growth trajectory
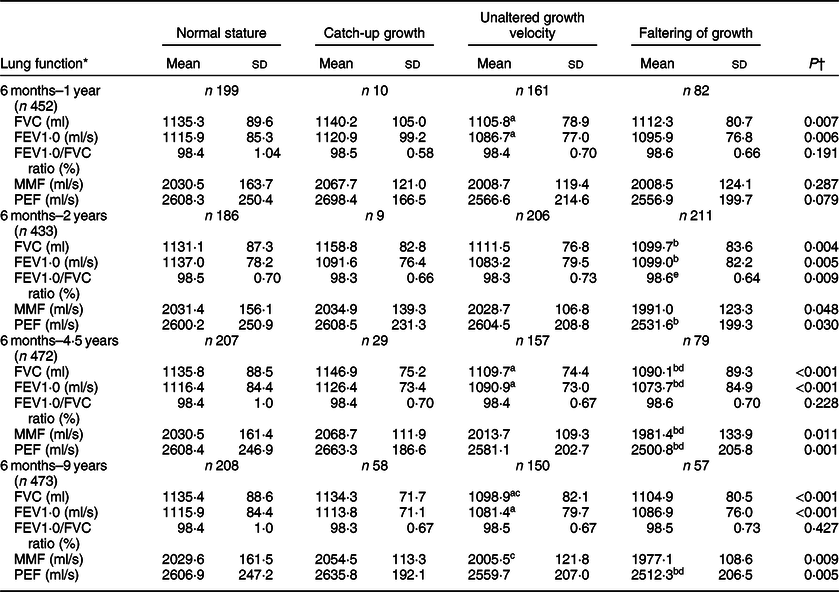
FVC, forced vital capacity; FEV1·0, forced expiratory volume per second; MMF, maximum mid-expiratory flow; PEF, peak expiratory flow.
* Mean values of lung function were standardised with sex and child’s age (months) at 9-year follow-up.
† The Mann–Whitney U test.
a Significant mean differences between normal stature and unaltered growth velocity groups, P < 0·05 (Bonferroni test).
b Significant mean differences between normal stature and growth faltering groups, P < 0·05 (Bonferroni test).
c Significant mean differences between catch-up and unaltered growth velocity groups, P < 0·05 (Bonferroni test).
d Significant mean differences between catch-up and growth faltering groups, P < 0·05 (Bonferroni test).
e Significant mean differences between unaltered growth velocity and growth faltering groups, P < 0·05 (Bonferroni test).
The FVC/ml, FEV1·0/ml per s and PEF/ml per s values were higher in children who demonstrated NS from the age of 1–2 years than that of children who had faltered growth (P < 0·05). The mean differences in FVC/ml, FEV1·0/ml per s, MMF/ml per s and PEF/ml per s values were observed within the NS group, catch-up group and children who remained in the same growth velocity from the age of 2–4·5 years (P < 0·05). Nevertheless, the mean values of FVC/ml and FEV1·0/ml per s were lower in children who demonstrated catch-up growth from the age of 4·5–9 years than that of children who maintained NS, those who remained in the same growth velocity as well as those who had faltering growth (online Supplemental Table 1). The adjusted regression coefficient also showed similar associations, indicating that the average lung function indices (FVC/ml, FEV1·0/ml per s, MMF/ml per s and PEF/ml per s) of children who experienced catch-up growth from the stunted group (at different ages during follow-up visits) were similar to that of NS children and better than that of stunted children who maintained the same growth velocity or had growth faltering (Table 7).
Table 7 Association between lung function indicators and height-for-age growth trajectory

FVC, forced vital capacity; FEV1·0, forced expiratory volume per second; MMF, maximum mid-expiratory flow; PEF, peak expiratory flow.
* Mean values of lung function were standardised with sex and child’s age (months) at 9-year follow-up.
† Referenced for normal growth.
‡ Adjusted variables; longitudinal growth trends, birth weight standardised with gestational age, parity, history of asthma, child-reported dry cough in the past 6 months, family history of asthma, maternal height and maternal age at pregnancy.
Discussion
This birth cohort study has revealed that stunted children with catch-up growth in HAZ especially before the age of 4·5 years had significantly improved lung function indices that were similar to that of NS children at the age of 9 years. In the current study, it was observed that children with PS throughout childhood had a greater reduction in lung function indices at preadolescent age. A higher proportion of children had growth faltering at the age of 2 years and their lung function value was lower, indicating a higher risk for future lung diseases. This early-life growth faltering possibly affects a crucial stage of lung growth and development, as generations of twenty-three airways and formations of 300 million alveoli occur within the first 2–3 years of human life(Reference Merkus, ten Have-Opbroek and Quanjer24). In healthy children, lung volume increases and reaches its determined size in late adolescence, then begins to decline steadily with increasing age(Reference Gibson and Doyle25), because lung function also follows the same timeline of growth. Research indicates that early childhood growth is an important determinant of suboptimal lung function in later adulthood(Reference Speizer and Tager26), and such early decline in lung function is associated with the subsequent development of chronic airflow obstruction(Reference McGeachie, Yates and Zhou27). Supporting the statement, the current study confirms that children who had growth faltering in all growth measurement trajectories demonstrated the lowest lung capacity at preadolescence. The aforementioned observations indicate that the risk of disease progression might increase with increasing age in children particularly with growth deprivation in early childhood. In contrast, children who reach a clinical normal growth (height-for-age) level at preschool age and demonstrate comparable lung function status with NS children may show an equal amount of respiratory activities of NS children in adulthood.
In the current study, a higher proportion of children showed catch-up growth from the age of 2–9 years (mostly at 4·5 years), while catch-up growth before the age of 4·5 years had more impact on lung function than that at the age of 9 years. Earlier studies reported that catch-up growth (by weight) in early life may be detrimental to later lung function(Reference Kotecha, Watkins and Heron14,Reference Hancox, Poulton and Greene28) . Children with a steady weight gain have shown a greater increase in FEV1·0 than those with periodic losses in weight(Reference Peterson, Jacobs and Milla12), which was observed for growing height (6 months–9 years) in the current study, indicating that children with catch-up growth in HAZ demonstrated improved exhaling capabilities. Thus, catch-up growth, especially at preschool age, had a greater impact on the improvement of lung function, which may eventually reduce the progression and disease severity later in life.
Stunting, a hallmark of chronic malnutrition, represents a systemic dysfunction during an important phase of physical development. The current study, similar to others, also observed deteriorating lung function in stunted children at the age of 9 years(Reference Weitz and Garruto29), along with a new observation that PS children had even a greater reduction in lung function (FFV1·0). Nearly half of the MINIMat children experienced at least an episode of stunting phenomenon up to 9 years of age, whereas a limited number of stunted children managed to catch up in growth during the study period. Thus, children with a higher growth faltering velocity generally had poor lung function in comparison to NS children. On the other hand, a higher proportion of preterm, SGA and LBW children showed a catch-up in growth at the age of 9 years, as these children with adverse birth events tended to return to their genetic trajectory for size after a period of delayed or arrested growth(Reference Ong, Ahmed and Emmett22). Our findings on chronic malnutrition, such as stunting, LBW and lung function, are broadly consistent with previous studies that have reported that undernutrition and LBW could lead to a more advanced change in lung structure and function(Reference Cai, Shaheen and Hardy13,Reference Gibson and Doyle25) . Such alterations can adversely influence respiratory health throughout the lifespan(Reference Gibson and Doyle25,Reference Luu, Katz and Leeson30) .
Postnatal undernutrition can create significant changes in lung function beyond merely an effect on lung size(Reference Riley and Thakker-Varia31–Reference Ong, Mehta and Ogston33). The current study observed a poor lung function among height-deprived growing children, in whom limb growth was compromised during or after an episode of growth faltering. Thus, these findings partly support the thrifty phenotype hypothesis that underlines that deprived growth is often linked with selective preservation of vital organs at the expense of other organs and tissues(Reference Hales and Barker34,Reference Barker35) . A prime function of the lung is to maintain oxygen supply to organs and tissues, especially the brain; however, in height-compromised children with shorter legs but preserved upper body height, lung function may be preserved by decreasing the ‘load’ on the lungs(Reference Wells36,Reference Lelijveld, Kerac and Seal37) . Therefore, a well-maintained lung function is needed in order to support brain energy metabolism(Reference Wells36,Reference Barker38) . Stunted children have a higher respiratory quotient (ratio of CO2 produced to O2 consumed while food is being metabolised) in the fasting state or 30 min after a meal as a consequence of altered metabolism compared with NS children(Reference Hoffman, Sawaya and Verreschi39). Both undernutrition and growth restriction are related to the weakening of pulmonary function in a vicious circle, which was observed in the current study(Reference Emery40).
The findings of the current study raise concerns that a higher proportion of children could neither manage to acquire catch-up growth nor were able to maintain the same growth velocity at preschool age (4·5 years), whereas their growth restriction was associated with compromised lung function at preadolescence. Therefore, their disease progression and disease susceptibility might be different at adulthood and that is attributable to the global threat of increasing chronic disease prevalence. A poorer lung function remains the basis of COPD diagnosis and a key predictor of disease prognosis(Reference Mannino, Watt and Hole41). Smoking, malnutrition, early-life infections, asthma and genetic predisposition are also important risk factors for COPD. According to the WHO, the global trend for COPD has been rising in many countries. In 2015, 3·17 million global deaths were attributed to COPD alone, where >90 % of COPD deaths occurred in low and middle-income countries(Reference Mathers and Loncar42). A hospital-based study reported that the prevalence of COPD was 13·5 % among Bangladeshi adults (>40 years), which is also an unacceptably underestimated public health problem in Bangladesh(Reference Alam, Chowdhury and Siddiquee16). With the findings of the current study, it is predictable that a higher number of MINIMat children who ever experienced stunting may experience a pulmonary disease in later life, which may also be true countrywide in Bangladesh with chronic undernutrition as a common phenomenon.
A major strength of the current study is its prospective design with multiple follow-up visits from birth to preadolescence. However, the current study had certain limitations. As with most previous studies, lung function was measured only once, and there was a lack of data on plethysmography, an essential diagnostic method of restrictive lung disease. The current study observed mean differences in lung function indicators by child’s sex. However, the current study did not have enough power to perform a stratified analysis by child’s sex to examine the association between lung function and child’s growth trajectory. Therefore, child sex and age ((in months) at 9-year follow-up) standardised mean values of each lung function indicator were calculated from raw values and used in the current analysis (except for raw lung values presented in Table 3). Furthermore, the MINIMat study participants from group A and group B belonged to the same locality, same culture and almost from the same socioeconomic strata. Therefore, no sensitivity analysis was conducted to examine sociodemographic differentials between group A and group B participants. Data on growth indicators for group B were not available. Information on maternal smoking during pregnancy is not common in Bangladesh’s sociodemographic perspective. In the MINIMat project, 0·05 % reported smoking on a daily basis during pregnancy. However, an earlier study has stated that the explored relationship was independent of maternal smoking status at pregnancy(Reference Edwards, Osman and Godden43). Another limitation of the current study was the absenteeism of some participants at every follow-up visit, but these missing data were not accounted for into the analysis. The current study adds to the evidence that the initiation of respiratory disease risk might be determined in early childhood with a poor performance of lung function. Thus, the current study advocates the need for preventive measures for LBW, growth-restricted and chronically undernourished children in future health services delivery. In response to the growing burden of non-communicable chronic diseases, the current study recommends identifying and monitoring high-risk children and the development of preventive strategies to reduce the burden of non-communicable diseases or slow down their progression and the detrimental aftereffects of associated risk factors.
Conclusions
Our data suggest that catch-up growth and/or recovery from stunting at preschool age is associated with better lung function at preadolescence. This observation underscores the importance of adopting potential life-course strategies aiming to prevent stunting as well as lung disease progression.
Acknowledgements
Acknowledgements: We sincerely thank all study participants who provided enormous efforts in the several follow-ups for the current study. Our gratitude extends to the field team members and data management staff for their support in data collection. Financial support: Financial support was provided by the International Centre for Diarrhoeal Disease Research, Bangladesh; the UK Medical Research Council; the Swedish Research Council; UNICEF; the UK Department for International Development; JSPS KAKENHI (grant number JP18256005); the Child Health and Nutrition Research Initiative; Uppsala University; the US Agency for International Development under cooperative agreement #388-G-00-02-00125-00; the Australian International Development Agency; the Government of Bangladesh; the Government of the Netherlands; the Swedish International Development Cooperative Agency; and the Swiss Agency for Development and Cooperation. Conflicts of interest: The authors have no conflicts of interest to declare. Authorship: F.F. and Y.W. conceptualised and designed the study, carried out most of data gathering, performed data analysis, drafted the initial manuscript and played a major role in writing the manuscript. Y.W., R.R. and E.-C.E. designed the data collection instruments, collected data, reviewed and revised the manuscript. S.A. helped with study design, carried out a significant portion of data gathering, initial analyses and reviewed and revised the manuscript. A.S.G.F. and M.J.C. helped with study design and reviewed and revised the manuscript. All authors read and approved the final manuscript as submitted. Ethics of human subject participation: Written informed consent was obtained from the parents. All procedures involving human research participants were approved by the ethics review committees of the icddr,b. The study followed the guidelines of the Declaration of Helsinki.
Supplementary material
For supplementary material accompanying this paper visit https://doi.org/10.1017/S1368980019004853