Impact statement
Our review discusses the ecological consequences of the late Pleistocene extinctions on major aspects of Earth systems as well as on the diet, distribution and behavior of surviving mammals. We demonstrate that the late Pleistocene loss of megafauna was pervasive and left legacies detectable within the modern atmosphere, geosphere, hydrosphere and biosphere. Moreover, the ecological roles that extinct and modern megafauna play in the Earth system are not replicated by smaller-bodied animals. Our review highlights the importance of integrating a paleontological perspective into modern conservation efforts to develop a more synoptic understanding of ecosystems.
Introduction
Paleontologists are fond of noting that extinction is the ultimate fate of any species. After all, turnover is a natural ongoing process in ecological communities; the average duration of a species is about 1–3 Ma (Raup, Reference Raup1981, Reference Raup1991; Foote and Raup, Reference Foote and Raup1996; Alroy, Reference Alroy2000; Vrba and DeGusta, Reference Vrba and DeGusta2004). Only rarely does the rate of extinction within a short temporal window rise to the level where we consider it unusual – a mass extinction event. There have been five of these that we know of in the geologic record (Raup and Sepkoski, Reference Raup and and Sepkoski1982; Sepkoski, Reference Sepkoski1984), and an additional widespread event during the late Pleistocene/Holocene (~100 ka to modern) that some modern scientists refer to as a mass extinction (May et al., Reference May, Lawton and May1995; Pimm et al., Reference Pimm, Russell, Gittleman and Brooks1995; Kolbert, Reference Kolbert2014; Ceballos et al., Reference Ceballos, Ehrlich, Barnosky, García, Pringle and Palmer2015, Reference Ceballos, Ehrlich and Dirzo2017). This latter event was unusual in a number of ways.
First, while certainly catastrophic, extinctions during the late Quaternary do not (or have not yet) risen to the level of a mass extinction. They fell well short of the threshold of 75% of species loss and moreover, were taxonomically selective targeting largely mammals and nonvolant birds (Martin and Klein, Reference Martin and Klein1984). Only if we project into the future and consider ongoing biodiversity loss part of the same process do we approach the threshold where this event rises to a mass extinction (Barnosky et al., Reference Barnosky, Matzke, Tomiya, Wogan, Swartz, Quental, Marshall, McGuire, Lindsey, Maguire, Mersey and Ferrer2011; Plotnick et al., Reference Plotnick, Smith and Lyons2016; Smith, Reference Smith2021). Second, the late Pleistocene extinctions were both spatially and temporally transgressive. They began earlier in Eurasia (~100–80 ka), then spread to Australia (~60–50 ka), North and South America (15–12 ka; Barnosky et al., Reference Barnosky, Koch, Feranec, Wing and Shabel2004; Lyons et al., Reference Lyons, Smith and Brown2004; Smith et al., Reference Smith, Elliott Smith, Lyons and Payne2018, Reference Smith, Elliott Smith, Lyons, Payne and Villaseñor2019) and finally islands (Holocene; Turvey, Reference Turvey2009; Slavenko et al., Reference Slavenko, Tallowin, Itescu, Raia and Meiri2016; Fromm et al., Reference Fromm, Meiri and McGuire2021). Furthermore, the intensity of extinctions was only slightly elevated from background in Eurasia and Africa (Smith et al., Reference Smith, Hamilton and Brown2010). Third, these extinctions were strikingly size- and diet-biased, disproportionately targeting large-bodied herbivorous mammals (Lyons et al., Reference Lyons, Smith and Brown2004; Smith et al., Reference Smith, Elliott Smith, Lyons and Payne2018). Finally, there was a clear and novel mechanism for this extinction – humans (Martin, Reference Martin, Martin and Wright1967, Reference Martin, Martin and Klein1984, Reference Martin2005; Martin and Steadman, Reference Martin, Steadman and RDE1999; Roberts et al., Reference Roberts, Flannery, Ayliffe, Yoshida, Olley, Prideaux, Laslett, Baynes, Smith, Jones and Smith2001; Lyons et al., Reference Lyons, Smith and Brown2004, Reference Lyons, Amatangelo, Behrensmeyer, Bercovici, Blois, Davis, DiMichele, Du, Eronen, Tyler Faith and Graves2016b; Miller et al., Reference Miller, Fogel, Magee, Gagan, Clarke and Johnson2005; Koch and Barnosky, Reference Koch and Barnosky2006; Haynes, Reference Haynes2009; Turvey, Reference Turvey2009; Turvey and Fritz, Reference Turvey and Fritz2011; Sandom et al., Reference Sandom, Faurby, Sandel and Svenning2014; Smith et al., Reference Smith, Elliott Smith, Lyons and Payne2018, Reference Smith, Elliott Smith, Lyons, Payne and Villaseñor2019).
Interestingly, while considerable research has focused on the role of humans in the terminal Pleistocene megafauna extinctions (e.g., Martin, Reference Martin, Martin and Wright1967, Reference Martin, Martin and Klein1984, Reference Martin2005; Lyons et al., Reference Lyons, Smith and Brown2004, Reference Lyons, Miller, Fraser, Smith, Boyer, Lindsey and Mychajliw2016a; Miller et al., Reference Miller, Fogel, Magee, Gagan, Clarke and Johnson2005; Koch and Barnosky, Reference Koch and Barnosky2006; Sandom et al., Reference Sandom, Faurby, Sandel and Svenning2014), much less has focused on the environmental legacy of the loss of tens of millions of large-bodied individuals from the landscape (but see Johnson, Reference Johnson2009; Malhi et al., Reference Malhi, Doughty, Galetti, Smith, Svenning and Terborgh2016; Smith et al., Reference Smith, Doughty, Malhi, Svenning and Terborgh2016a, Reference Smith, Elliott Smith, Lyons, Payne and Villaseñor2019; Galetti et al., Reference Galetti, Moleón, Jordano, Pires, Guimaraes, Pape, Nichols, Hansen, Olesen, Munk and de Mattos2018; Smith, Reference Smith2021). Given the precarious state of large-bodied mammals today (Cardillo et al., Reference Cardillo, Mace, Jones, Bielby, Bininda-Emonds, Sechrest, Orme and Purvis2005; Dirzo et al., Reference Dirzo, Young, Galetti, Ceballos, Isaac and Collen2014; Ceballos et al., Reference Ceballos, Ehrlich, Barnosky, García, Pringle and Palmer2015, Reference Ceballos, Ehrlich and Dirzo2017; Ripple et al., Reference Ripple, Estes, Beschta, Wilmers, Ritchie, Hebblewhite, Berger, Elmhagen, Letnic, Nelson and Schmitz2014, Reference Ripple, Wolf, Newsome, Betts, Ceballos, Courchamp, Hayward, Van Valkenburgh, Wallach and Worm2019), it may well be that more than any other extinction in the history of life on our planet, the late Pleistocene megafauna extinction can inform modern conservation biology by providing insights about what might happen if our current biodiversity crisis is not slowed or halted (Barnosky et al., Reference Barnosky, Lindsey, Villavicencio, Bostelmann, Hadly, Wanket and Marshall2016; Malhi et al., Reference Malhi, Doughty, Galetti, Smith, Svenning and Terborgh2016; Smith et al., Reference Smith, Doughty, Malhi, Svenning and Terborgh2016a, Reference Smith, Elliott Smith, Lyons and Payne2018; Galetti et al., Reference Galetti, Moleón, Jordano, Pires, Guimaraes, Pape, Nichols, Hansen, Olesen, Munk and de Mattos2018; Smith, Reference Smith2021).
To assess the influence of the terminal Pleistocene extinction on Earth systems, we first need to appreciate just how uniquely size biased it was; no other extinctions in the Cenozoic mammal record approached the degree of size selectivity seen at this time (Alroy, Reference Alroy and MacPhee1999; Smith et al., Reference Smith, Elliott Smith, Lyons and Payne2018). Indeed, body mass is not usually a good predictor of extinction risk for mammals in the fossil record (Smith et al., Reference Smith, Elliott Smith, Lyons and Payne2018). However, by the late Pleistocene, there was a highly significant positive association between body size and extinction risk – the average mass of victims was more than 2–3 orders of magnitude higher than survivors (Smith et al., Reference Smith, Elliott Smith, Lyons and Payne2018). Moreover, this size bias occurred on each continent and within each trophic guild, and it followed the movement of humans across the globe (Lyons et al., Reference Lyons, Smith and Brown2004; Smith et al., Reference Smith, Elliott Smith, Lyons and Payne2018, Reference Smith, Elliott Smith, Lyons, Payne and Villaseñor2019). Thus, in examining the legacy of this extinction, we are necessarily focusing on the consequences of truncating the right side of the body size distribution of mammals; that is, losing all of the largest bodied species within communities (Figure 1). In North America, for example, all mammals >600 kg went extinct (Lyons et al., Reference Lyons, Smith and Brown2004).
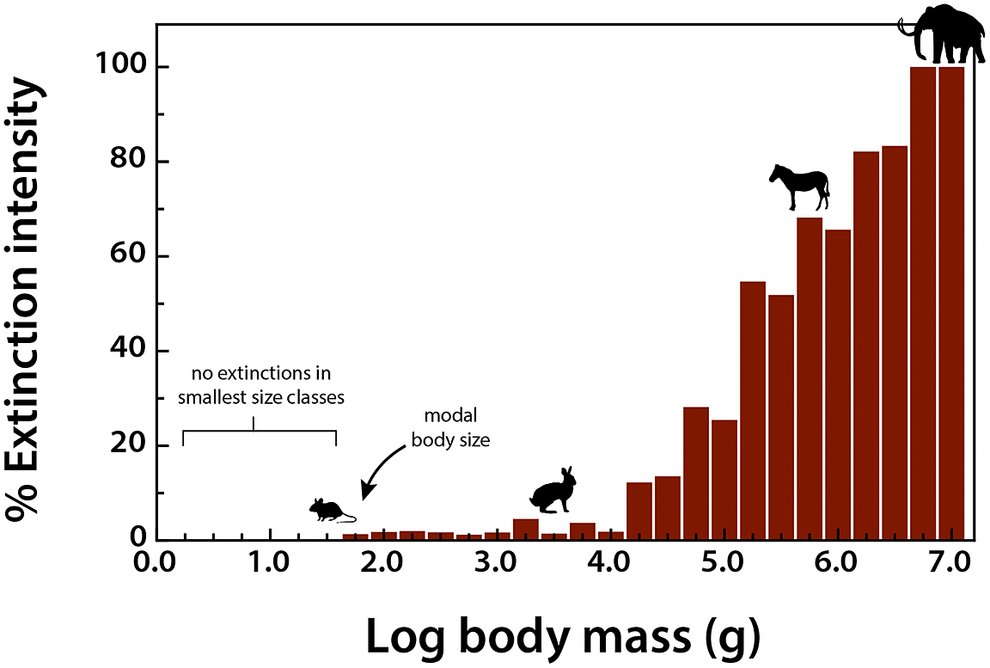
Figure 1. Proportion of extinctions within each body size class of terrestrial nonvolant mammals over the late Pleistocene and Holocene. Data are averaged across the globe; insular mammals are not shown. Because the median body mass of mammals is under 100 g (log2), most mammals were unaffected by this event; however, extinction risk rose sharply with larger body mass. Such extreme size-selectivity is unique in the vertebrate fossil record (Data from Smith et al., Reference Smith, Elliott Smith, Lyons and Payne2018).
Large-bodied mammals play an important, even ‘oversized’ role in ecosystems (Owen-Smith, Reference Owen-Smith1988). This arises from their large mass and high energetic requirements, which require physical space, abundant forage, large home ranges and dictate large-scale movement (Peters, Reference Peters1983; Owen-Smith, Reference Owen-Smith1988). Thus, megafauna potentially influence all aspects of the Earth system – the atmosphere, geosphere, hydrosphere and biosphere, leading to unique and pervasive influences not replicated by smaller-bodied animals (Hyvarinen et al., Reference Hyvarinen, Te Beest, le Roux, Kerley, de Groot, Vinita and Cromsigt2021). Logically, the megafauna of the terminal Pleistocene had a much greater impact on Earth systems because of their even larger size (Figure 2; Haynes, Reference Haynes2012; Smith et al., Reference Smith, Doughty, Malhi, Svenning and Terborgh2016a). The Columbian mammoth (Mammuthus columbi), for example, was approximately three times more massive than the average modern elephant and more than an order of magnitude larger than a domestic cow (Smith et al., Reference Smith, Lyons, Ernest, Jones, Kaufman, Dayan, Marquet, Brown and Haskell2003). Even carnivores were bigger; species of extinct sabertooth cats weighed up to 400 kg, about twice the average size of an African lion (Smith et al., Reference Smith, Lyons, Ernest, Jones, Kaufman, Dayan, Marquet, Brown and Haskell2003). Because many life history and ecological attributes of animals scale non-linearly with size (Peters, Reference Peters1983), this suggests Pleistocene megafauna had a disproportionately greater influence on environments and biotic interactions than do modern megafauna (Smith et al., Reference Smith, Doughty, Malhi, Svenning and Terborgh2016a).
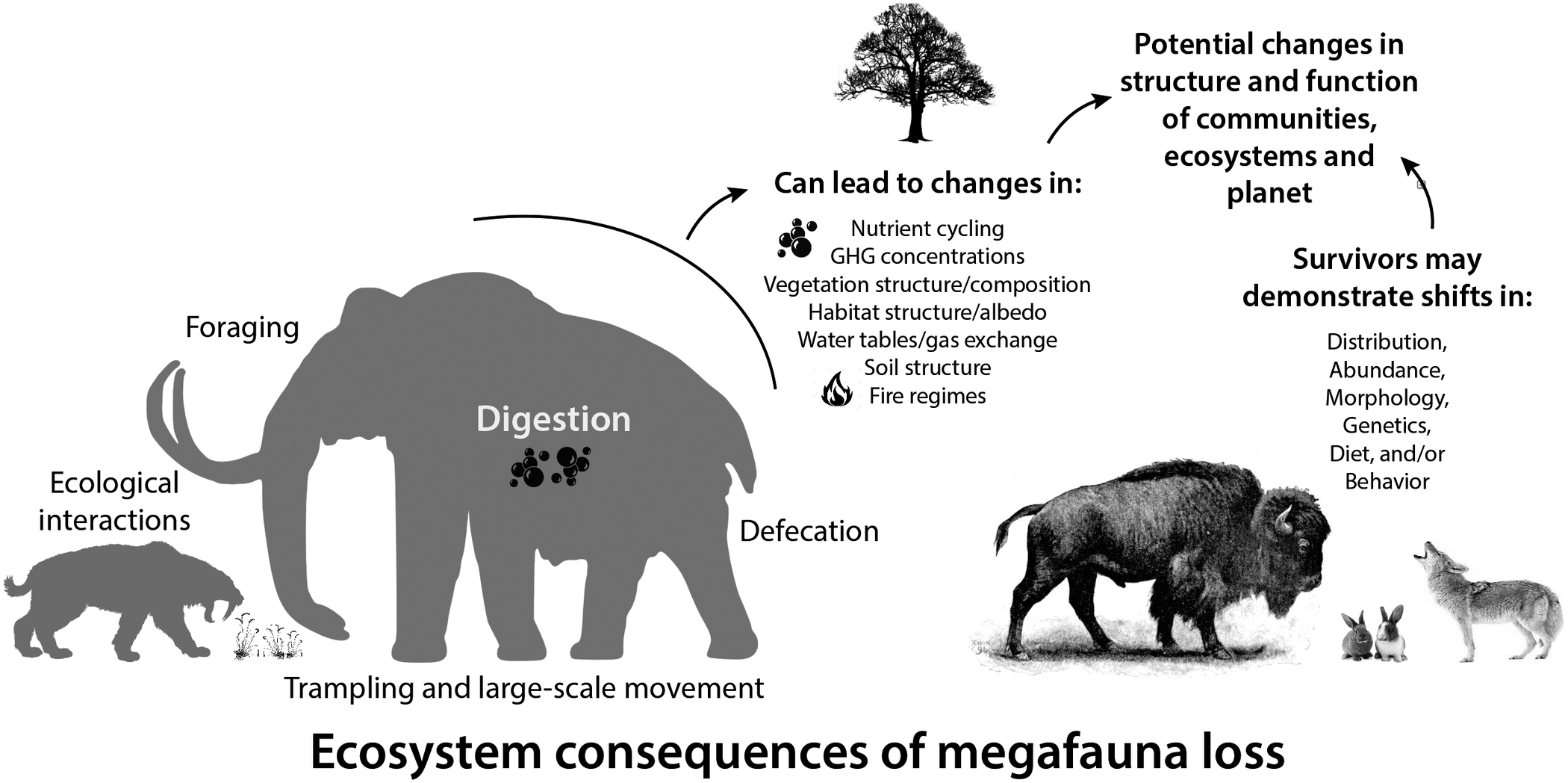
Figure 2. Consequences of the loss of large-bodied mammals in terrestrial ecosystems. Megafauna were (and are) unique members of ecological communities and their physiological, life history, and ecological activities have disproportionate influences on community, ecosystem and earth system processes. Silhouettes here and elsewhere from Phylopic (http://phylopic.org).
Here, we explore how essential activities, such as foraging, digestion and movement of megafauna influenced various physical aspects of the environment, vegetation structure and composition, nutrient cycling and gas exchange (Figure 2). Much of this work borrows from what we know about contemporary mammals and/or is based on modeling efforts. We also explore what we know thus far about the response of surviving mammals in the early Holocene to the extinction of their congeners, particularly in terms of potential dietary shifts, changes in morphology and/or behavior and distribution shifts. Finally, we examine how these changes influenced the overall function of communities at various spatial scales and the ecological interactions within them. Because much of our collective expertise is with North American ecosystems, we often use this continent as a case example. Moreover, many of our more detailed explorations are taken from work at Hall’s Cave, an exceptional well-dated late Quaternary fossil site in the Edward’s Plateau of Texas where we have worked for the past 8 years. However, we note our findings are likely applicable globally, and when and where we can, we highlight research in other ecosystems and continents. Our aim is to provide a synoptic understanding of the ecological legacy of the terminal Pleistocene megafaunal extinction on surviving animals and ecosystems.
Movement
Being very large brings physical challenges as animals interact with their environment (Owen-Smith, Reference Owen-Smith1988). Here, we discuss how megafauna influence the landscape through their movements up and down slope, over large distances, and the consequences of their mass on soil and water tables. While researchers have explored these effects to some extent with extant animals (i.e., Owen-Smith, Reference Owen-Smith1988), extinct megaherbivores almost certainly had much more profound influences on their environment; these remain poorly characterized.
Slope
Body size influences movement (Peters, Reference Peters1983). For example, the path that a large-bodied mammal takes as it travels up and down mountain slopes is different than that of smaller mammals (Reichman and Aitchison, Reference Reichman and Aitchison1981; Figure 3). This reflects substantial differences in the energetic costs of transport, which are mediated by body mass, gravity and the slope of the incline. For example, for a horse to move up a 15% incline requires a 630% increase in metabolism relative to moving along a flat surface (Reichman and Aitchison, Reference Reichman and Aitchison1981). Consequently, trail angles significantly decrease with increasing body size (Figure 3). While small mammals may scamper straight up a slope, larger ones make many turns as they traverse a mountain. It follows that the difference in the way animals walk up (or down) a mountain slope leads to differences in the steepness and physiographic terracing of game trails when megaherbivores are present. The permeance of such trails is also influenced by the weight and likely the foot type of the animal; megaherbivores with hard hoofs are much more likely to produce hard-packed trails. There are likely environmental consequences; studies of hiking trails have demonstrated that modification of slopes can lead to erosion, changes in water movement if the trail channels rain water or snow melt, and influence surrounding vegetative communities (Yoda and Watanabe, Reference Yoda and Watanabe2000). While national parks and other entities have examined the physiographic effects of hiking trails (e.g., Yoda and Watanabe, Reference Yoda and Watanabe2000), little work to date has examined how game trails made by animals of different body sizes might influence the landscape.
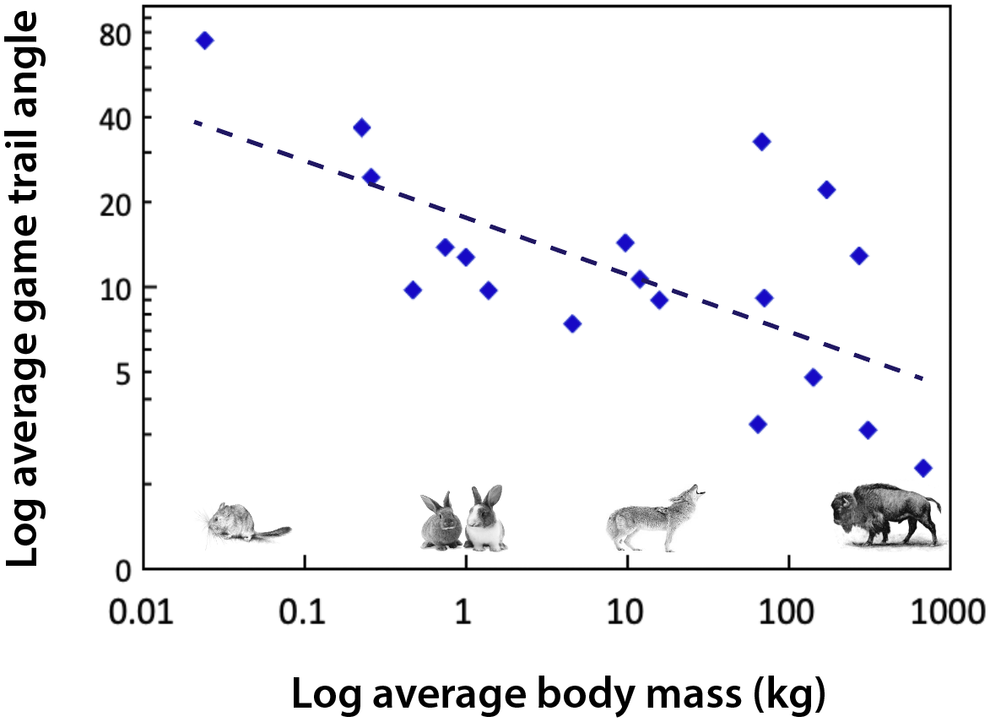
Figure 3. The angle of animal game trails up mountain slopes as a function of body mass. Data were extracted from Figure 2 in Reichman and Aitchison (Reference Reichman and Aitchison1981) and are binned by species; mammals plotted range from a mouse (0.025 kg) to a bison (680 kg). Trail angles were determined by measuring footprints in snow and include measurements conducted on a variety of different slope inclines. Since the trail angle is influenced by slope incline (Reichman and Aitchison, Reference Reichman and Aitchison1981) this contributes to considerable scatter in the data. If data had been standardized for slope, this relationship might be steeper. Equation: Log trail angle (degrees) = −0.1935 log body mass (kg) + 1.2415; df = 17; r 2 = 0.4158; P < .01.
Distance
Modern megafauna move enormous distances (Owen-Smith, Reference Owen-Smith1988). Even within their greatly restricted modern geographic range (e.g., Mahmood et al., Reference Mahmood, Vu, Campos-Arceiz, Akrim, Andleeb, Farooq, Hamid, Munawar, Waseem, Hussain and Fatima2021), elephants can travel more than 2,800 km annually (Mills et al., 2018). Yet, this is only a fraction of their historic movement patterns. In the past, mammoths and other large-bodied mammals may have roamed across entire or even multiple continents (Kahlke, Reference Kahlke2015; Wooller et al., Reference Wooller, Bataille, Druckenmiller, Erickson, Groves, Haubenstock, Howe, Irrgeher, Mann, Moon and Potter2021; Miller et al., Reference Miller, Fisher, Crowley, Secord and Konomi2022). While we know little about landscape effects of mammoth movement, modern studies of elephants suggest as these giants move through the landscape they have detectable impacts on the density of trees and forest structure (Asner and Levick, Reference Asner and Levick2012). Their bulk means that it is inevitable that they will knock down trees and create gaps in the vegetation as they move through forests. By creating gaps in what would otherwise be closed-canopy vegetation, megafauna provide opportunities for other plants to establish (Asner and Levick, Reference Asner and Levick2012). The subsequent changes in forest structure can be profound; elephants are two times more important than fire in regulating tree falls and recruitment (Asner et al., Reference Asner, Vaughn, Smit and Levick2016). For example, elephant damage has led to a notable lack of small trees in Gabon’s forest compared to the Amazon and reduced alpha diversity of the vegetation (Terborgh et al., Reference Terborgh, Davenport, Niangadouma, Dimoto, Mouandza, Scholtz and Jaen2016a, Reference Terborgh, Davenport, Ong and Campos‐Arceizb). Not surprisingly, areas where megafauna are not present tend to demonstrate increases in woody cover (Asner et al., Reference Asner, Levick, Kennedy-Bowdoin, Knapp, Emerson, Jacobson, Colgan and Martin2009, Reference Asner, Vaughn, Smit and Levick2016; Gill et al., Reference Gill, Williams, Jackson, Lininger and Robinson2009; Asner and Levick, Reference Asner and Levick2012; Barnosky et al., Reference Barnosky, Lindsey, Villavicencio, Bostelmann, Hadly, Wanket and Marshall2016). Given the relationship between geographic range size, home range size and body size (Brown, Reference Brown1995) as well as the expected migration distances, extinct megafauna would have had effects across much larger areas than modern animals do today.
Soil compaction
The largest extinct Pleistocene megafauna exceeded 10 tons (Smith et al., Reference Smith, Lyons, Ernest, Jones, Kaufman, Dayan, Marquet, Brown and Haskell2003) and even the smallest of them generally weighed more than a ton. Moreover, it is likely that many of these species traveled in social herds, much like modern large-bodied mammals. The concentration of large-bodied animals within an area undoubtedly led to localized compression of soils, which may have reduced habitat for burrowing animals such as gophers. Moreover, by removing air pockets, soil compaction can alter water and gas exchange with the atmosphere, which in turn, can have substantial regional and even global effects on climate (see section ‘Biogeochemical change’). Soil compaction also influences permeability, which directly influences nutrient exchange and the diversity of microbes within soils (Wang et al., Reference Wang, Zhang, Staley, Gao, Ishii, Wei, Liu, Cheng, Hao and Sadowsky2019). Several studies demonstrate that bioturbation and soil compaction by modern herbivores alters microbial composition (Liu et al., Reference Liu, Kan, Yang and Zhang2015; Wang et al., Reference Wang, Zhang, Staley, Gao, Ishii, Wei, Liu, Cheng, Hao and Sadowsky2019); for example, regions where muskoxen graze in Greenland have much higher microbial diversity than do un-grazed soils (Aggerbeck et al., Reference Aggerbeck, Nielsen, Mosbacher, Schmidt and Hansen2022). Others report that soil compaction and grazing by megaherbivores reduce carbon and nitrogen pools (Mosbacher et al., Reference Mosbacher, Michelsen, Stelvig, Hjermstad-Sollerud and Schmidt2019).
Foraging
Because megaherbivores rely on abundant, but low-quality resources, they necessarily spend the majority of their time foraging. The high absolute energetic requirements of megaherbivores, coupled with their large-scale movements, means their impacts are widespread and would have been even more so in the past. Thus, foraging has important ecosystem consequences, which we discuss below. These include changes in vegetation structure and composition, the openness and patchiness of vegetation, influence on fuel loads and fire regimes, long-distance dispersal of seeds, as well as physically disturbing the soil surface, changing the albedo of the surface, and recycling nutrients. Such effects vary along rainfall and soil fertility gradients (Augustine and McNaughton, Reference Augustine and McNaughton2006; Waldram et al., Reference Waldram, Bond and Stock2008; Pringle et al., Reference Pringle, Prior, Palmer, Young and Goheen2016; Hyvarinen et al., Reference Hyvarinen, Te Beest, le Roux, Kerley, de Groot, Vinita and Cromsigt2021). Note that much of our speculation about the role of megaherbivores in Pleistocene ecosystems is based on studies of one system – modern African elephants in savannah ecosystems (Hyvarinen et al., Reference Hyvarinen, Te Beest, le Roux, Kerley, de Groot, Vinita and Cromsigt2021), which may provide slightly distorted inferences. However, it is likely that Pleistocene megafauna had somewhat similar, albeit much greater, impacts on late Quaternary ecosystems.
Vegetation structure and composition
Numerous studies have documented the important influence of large-bodied herbivores on plant structure and composition, and on maintaining habitat heterogeneity (e.g., Owen-Smith, Reference Owen-Smith1987, Reference Owen-Smith1988, Reference Owen-Smith1989; Cumming et al., Reference Cumming, Fenton, Rautenbach, Taylor, Cumming, Cumming, Dunlop, Ford, Hovorka, Johnston and Kalcounis1997; Whyte et al., Reference Whyte and Du Toit2003; Western and Maitumo, Reference Western and Maitumo2004; Waldram et al., Reference Waldram, Bond and Stock2008; Johnson, Reference Johnson2009; Haynes, Reference Haynes2012; Rule et al., Reference Rule, Brook, Haberle, Turney, Kershaw and Johnson2012; Keesing and Young, 2014; Asner et al., Reference Asner, Vaughn, Smit and Levick2016; Bakker et al., Reference Bakker, Gill, Johnson, Vera, Sandom, Asner and Svenning2016a, Reference Bakker, Pages, Arthur and Alcoverrob; Ratajczak et al., Reference Ratajczak, Collins, Blair, Koerner, Louthan, Smith, Taylor and Nippert2022). Browsers, such as deer or antelope, tend to have an inhibiting effect on woody vegetation, while the effects of grazers, such as zebra, bison and horses, appear to be mixed (Bakker et al., Reference Bakker, Gill, Johnson, Vera, Sandom, Asner and Svenning2016a, Reference Bakker, Pages, Arthur and Alcoverrob). Large-bodied grazers can help maintain open grasslands and inhibit woodland regeneration through the trampling and destruction of tree seedlings, but they apparently can also have a positive effect on woodlands by reducing potential competition between grasses and shrubs (Johnson, Reference Johnson2009; Bakker et al., Reference Bakker, Gill, Johnson, Vera, Sandom, Asner and Svenning2016a, Reference Bakker, Pages, Arthur and Alcoverrob). Complicating our understanding of how multiple species of megaherbivores in the late Pleistocene would have impacted plant communities is that the dietary preferences of some megaherbivores appear to have been variable across time and/or space. For example, stable isotope analysis revealed that within a single location, camels (Camelops sp.) in central Texas foraged across the entire C3 and C4 vegetation spectrum during the late Pleistocene (Smith et al., Reference Smith, Elliott Smith, Villaseñor, Tomé, Lyons and Newsome2022). Individual camels within the population could be characterized as browsers, mixed feeders or grazers, suggesting a high degree of individual specialization. Other work finds similar results for Palaeolama (Franca et al., Reference de Melo França, de Asevedo, Dantas, Bocchiglieri, dos, Avilla, Lopes and da Silva2015).
The maintenance of open grassland ecosystems at the expense of woodlands is beneficial for other herbivores; by shifting carbon storage from where it was locked inside woody tissues to the leaves of grass, carbon becomes more freely accessible (Johnson, Reference Johnson2009). Interestingly, foraging by bison in large herds alters the nutrient quality of the plants upon which they feed (Geremia et al., Reference Geremia, Merkle, Eacker, Wallen, White, Hebblewhite and Kauffman2019). By feeding intensively in a local patch over a period of weeks, bison stimulate plant growth, but delay plant maturation causing the plants to remain high-quality forage for longer periods of time. In effect, they engineer their own ‘green wave’. During the late Pleistocene, when large herds of bison would have been more common, grazing would have altered the quality of graze in the ecosystems where they occurred, facilitating the coexistence of other large herbivores. It is possible that the increased quality of forage would have supported larger population sizes, although this has not been investigated.
Because extinct megafauna were so much larger than contemporary animals, the physiographic effects they had on landscapes were more transformative. For example, modeling suggests that as much as 29% of the savanna woody cover in the South American continent may have been lost following the megafauna extinction (Doughty et al., Reference Doughty, Wolf, Morueta‐Holme, Jørgensen, Sandel, Violle, Boyle, Kraft, Peet, Enquist and Svenning2016a). Furthermore, the heterogeneity of the landscape may also have been altered. Not only were woody savannas of South America more open and grassy when megafauna were present, but also there were mosaics of different vegetation types (Doughty et al., Reference Doughty, Wolf, Morueta‐Holme, Jørgensen, Sandel, Violle, Boyle, Kraft, Peet, Enquist and Svenning2016a). These likely reflected differential space use by megafauna relative to smaller-bodied mammals; perhaps areas they could not access because of their size (Doughty et al., Reference Doughty, Wolf, Morueta‐Holme, Jørgensen, Sandel, Violle, Boyle, Kraft, Peet, Enquist and Svenning2016a). In Australia, the loss of megafauna led to a wholescale transformation of mixed rainforest into sclerophyll vegetation (Rule et al., Reference Rule, Brook, Haberle, Turney, Kershaw and Johnson2012). The northern hemisphere saw the loss of the vast ‘mammoth steppe’ – the largest biome of the Pleistocene consisting of a diverse assemblage of grasses, forbs and sedges – which had been maintained at least partially through grazing and other activities by large-bodied mammoth, camelids and bison (Zimov et al., Reference Zimov, Chuprynin, Oreshko, Chapin, Reynolds and Chapin1995, Reference Zimov, Zimov, Tikhonov and Chapin2012; Guthrie, Reference Guthrie2001; Johnson, Reference Johnson2009).
Similarly, in the Neotropics, the megafauna extinction left a significant imprint on the current plant trait and ecosystem biogeography (Dantas and Pausas, Reference Dantas and Pausas2020). Not only did some ecosystems shift from grasslands to forest after the extinction, but also the distribution of plant defense traits (i.e., spinescence, leaf size and wood density), which had been correlated with Pleistocene megaherbivore density and distribution, became decoupled (Dantas and Pausas, Reference Dantas and Pausas2020). This led to many ‘evolutionary anachronisms’; that is, taxa whose ecological traits and adaptations are now obsolete for contemporary ecosystems (Janzen and Martin, Reference Janzen and Martin1982; Barlow, Reference Barlow2002; Guimarães et al., Reference Guimarães, Galetti and Jordano2008). Consequently, the modern distribution of anti-herbivore defense traits in the Neotropics reflects the historical distribution of extinct megafauna rather than that of extant animals (Dantas and Pausas, Reference Dantas and Pausas2020).
Species diversity
An ecosystem engineer is a species whose activities increase or create habitat in an environment (Jones et al., Reference Jones, Lawton and Shachak1994). While the degree of the effect varies between ecosystems (marine vs. terrestrial) and environments (forests, deserts, vs. grasslands), ecosystem engineers tend to increase species richness within the habitat (Jones et al., Reference Jones, Lawton and Shachak1994; Romero et al., Reference Romero, Gonçalves‐Souza, Vieira and Koricheva2015; Coggan et al., Reference Coggan, Hayward and Gibb2018). Indeed, modern ecosystem engineers can increase species richness in a community by 25% (Romero et al., Reference Romero, Gonçalves‐Souza, Vieira and Koricheva2015). Very large-bodied animals are especially important as engineers (Owen-Smith, Reference Owen-Smith1988) – a niche largely lost from communities after the late Pleistocene megafauna extinction.
Bison, one of the few surviving large-bodied taxa in North America, demonstrate this clearly; their reintroduction into tall prairie grasslands more than doubled native plant species richness (Ratajczak et al., Reference Ratajczak, Collins, Blair, Koerner, Louthan, Smith, Taylor and Nippert2022). This effect came about for several reasons. First, because bison consumed the dominant plant species in the habitat, they facilitated increases in the abundance and presence of other plant taxa. Second, their physical disturbances of the environment, such as the production of ‘bison wallows’ (depressions in the soil) greatly increased habitat heterogeneity, thereby also increasing plant species diversity. While grazing by cattle also increased plant diversity in tall grass prairie habitats, the effect was much weaker than that of the larger-bodied bison (Ratajczak et al., Reference Ratajczak, Collins, Blair, Koerner, Louthan, Smith, Taylor and Nippert2022).
Large-bodied mammals strongly influence the architecture of the environment with ecological engineering effects dependent on the mammal and habitat (Estes et al., Reference Estes, Terborgh, Brashares, Power, Berger, Bond, Carpenter, Essington, Holt, Jackson and Marquis2011; Young et al., Reference Young, McCauley, Dirzo, Goheen, Agwanda, Brook, Otárola-Castillo, Ferguson, Kinyua, McDonough and Palmer2015; Goheen et al., Reference Goheen, Augustine, Veblen, Kimuyu, Palmer, Porensky, Pringle, Ratnam, Riginos, Sankaran and Ford2018). In some ecosystems, megaherbivores may maintain habitat for other taxa, while in others, they reduce it (Keesing, Reference Keesing1998, Reference Keesing2000; Estes et al., Reference Estes, Terborgh, Brashares, Power, Berger, Bond, Carpenter, Essington, Holt, Jackson and Marquis2011; Young et al., Reference Young, McCauley, Dirzo, Goheen, Agwanda, Brook, Otárola-Castillo, Ferguson, Kinyua, McDonough and Palmer2015). Either has implications for smaller-bodied vertebrates and the ecosystem. For example, the experimental exclusion of large-bodied herbivores from sites in Kenya and East Africa led to increases in small mammal abundance likely because of increased food quality (Keesing, Reference Keesing1998, Reference Keesing2000; Young et al., Reference Young, McCauley, Dirzo, Goheen, Agwanda, Brook, Otárola-Castillo, Ferguson, Kinyua, McDonough and Palmer2015). However, the increased density of small-bodied mammals led in turn to sharp increases in the number of medium and small-sized predators (Keesing, Reference Keesing1998, Reference Keesing2000). Cover also matters. In shrublands and savannahs, megaherbivore grazing can decrease small mammal biodiversity if the reduction of cover leads to more exposure to predators (Moser and Witmer, Reference Moser and Witmer2000). However, by damaging trees and increasing the structural complexity of the habitat, megaherbivores can also increase the ability of taxa to avoid detection by predators (Pringle, Reference Pringle2008). All of these effects are influenced by the size of the animal, and so would likely have been much greater in the past.
Seed dispersal
Many plants rely on animals for seed dispersal. Mammalian seed dispersers serve that role through multiple pathways including transporting seeds that get caught in their fur, and through foraging. However, the effectiveness of mammal-mediated seed dispersal is influenced by body size. While foraging by small-bodied mammals has detectable effects on the structure of forests (e.g., Guimarães et al., Reference Guimarães, Galetti and Jordano2008; Campos-Arceiz and Blake, Reference Campos-Arceiz and Blake2011; Bueno et al., Reference Bueno, Guevara, Ribeiro, Culot, Bufalo and Galetti2013; Pires et al., Reference Pires, Guimarães, Galetti and Jordano2018), they do not replicate the role megafauna play in plant dispersal (Janzen and Martin, Reference Janzen and Martin1982; Barlow, Reference Barlow2002). Smaller-bodied herbivores collect and cache seeds, but often discard the kernel while eating some of the flesh of the fruit (Barlow, Reference Barlow2002; Johnson, Reference Johnson2009). Even if they eat seeds, they do not transport them as far as larger bodied herbivores because of their smaller home range (Kelt and Van Vuren, Reference Kelt and Van Vuren2001). Indeed, on average extinct megaherbivores dispersed seeds 10 times further than do contemporary smaller-bodied herbivores (Pires et al., Reference Pires, Guimarães, Galetti and Jordano2018). Moreover, germination for many large-seeded plants requires passage through the guts of large-bodied herbivores; this process removes the pulp and mechanically and/or chemically abrades the seed (Traveset et al., Reference Traveset, Rodriguez-Perez and Pias2008). Many seeds are too large for surviving small-bodied herbivores to effectively process. Such plants are another example of an evolutionary anachronism (see section ‘Vegetation structure and composition’). Even in regions with extant megaherbivores, large-seeded plants that depend on them for dispersal are declining in abundance or exhibiting a contraction of their geographic range because of reductions in the abundance of megafauna (Janzen and Martin, Reference Janzen and Martin1982; Barlow, Reference Barlow2002; Doughty et al., Reference Doughty, Wolf, Baraloto and Malhi2016c). For example, 4.5% of tree species in the Congo require an animal the size of an elephant (or larger) to disperse their seeds (Beaune et al., Reference Beaune, Fruth, Bollache, Hohmann and Bretagnolle2013). These trees are experiencing population declines because of decreases in the abundance of forest elephants. Although there is as yet little evidence of plant extinctions as a result of seed dispersal limitations, declines in tree abundance have important implications for carbon flux and biomass in forests (Doughty et al., Reference Doughty, Wolf, Morueta‐Holme, Jørgensen, Sandel, Violle, Boyle, Kraft, Peet, Enquist and Svenning2016a, c).
Fire regimes
The role of herbivores in mediating the fire regime within landscapes is unequivocal (Owen-Smith, Reference Owen-Smith1987, Reference Owen-Smith1988; Flannery, Reference Flannery1994; Waldram et al., Reference Waldram, Bond and Stock2008; Johnson, Reference Johnson2009; Johnson et al., Reference Johnson, Prior, Archibald, Poulos, Barton, Williamson and Bowman2018; Rouet-Leduc et al., Reference Rouet-Leduc, Pe’er, Moreira, Bonn, Helmer, Shahsavan Zadeh, Zizka and van2021 and references therein). As discussed above, foraging alters the abundance of flammable woody vegetation and/or grasses, and promotes habitat heterogeneity, which in turn can influence the frequency, intensity and extent of fire within ecosystems (Owen-Smith, Reference Owen-Smith1987, Reference Owen-Smith1988; Bakker et al., Reference Bakker, Gill, Johnson, Vera, Sandom, Asner and Svenning2016a; Johnson et al., Reference Johnson, Prior, Archibald, Poulos, Barton, Williamson and Bowman2018). Moreover, in the absence of herbivores, organic matter can accumulate and enhance both the frequency and intensity of fires. Given these effects, promotion of grazing by contemporary herbivores has been proposed as a tool for reducing wildfire risk in some habitats (Johnson et al., Reference Johnson, Prior, Archibald, Poulos, Barton, Williamson and Bowman2018; Rouet-Leduc et al., Reference Rouet-Leduc, Pe’er, Moreira, Bonn, Helmer, Shahsavan Zadeh, Zizka and van2021). This appears to be most effective when a mix of browsers and grazers is employed (Rouet-Leduc et al., Reference Rouet-Leduc, Pe’er, Moreira, Bonn, Helmer, Shahsavan Zadeh, Zizka and van2021). Other studies demonstrate livestock grazing appears to reduce fire frequency in tropical ecosystems, likely because grazing inhibits tree recruitment (Bernardi et al., Reference Bernardi, Staal, Xu, Scheffer and Holmgren2019).
Given the results from modern studies, it is not surprising that a fundamental change in fire regimes has been reported for the late Pleistocene at many fossil localities following the megafauna extinction (Burney et al., Reference Burney, Robinson and Burney2003; Bond and Keeley, Reference Bond and Keeley2005; Gill et al., Reference Gill, Williams, Jackson, Lininger and Robinson2009, Reference Gill, Williams, Jackson, Donnelly and Schellinger2012; Rule et al., Reference Rule, Brook, Haberle, Turney, Kershaw and Johnson2012; Gill, Reference Gill2014; Bakker et al., Reference Bakker, Gill, Johnson, Vera, Sandom, Asner and Svenning2016a, Reference Bakker, Pages, Arthur and Alcoverrob). For example, sedimentary charcoal records indicate a widespread increase in fire across the globe from the late Pleistocene through the Holocene, although these authors largely attributed it to climate shifts (Daniau et al., Reference Daniau, Bartlein, Harrison, Prentice, Brewer, Friedlingstein, Harrison‐Prentice, Inoue, Izumi, Marlon and Mooney2012). The shift in vegetation from mixed rainforest to sclerophyll vegetation in Australia resulted in an increase in fire frequency (Flannery, Reference Flannery1994; Rule et al., Reference Rule, Brook, Haberle, Turney, Kershaw and Johnson2012). Not only were fires more frequent, but also they were likely more intense (Flannery, Reference Flannery1994; Waldram et al., Reference Waldram, Bond and Stock2008), which can lead to feedback loops between herbivore densities and fires. Larger, more intense fires can change herbivore foraging behavior in a way that increases the chances of more large fires (Waldram et al., Reference Waldram, Bond and Stock2008). Similar shifts in vegetation and fire frequency were reported in North America (e.g., Gill et al., Reference Gill, Williams, Jackson, Lininger and Robinson2009).
Digestion
Large-bodied herbivores require enormous quantities of food. For example, a wild adult elephant can feed up to 18 h a day, and consume as much as 200–300 kg of vegetation within that time frame (Sukumar et al., Reference Sukumar2006; https://elephantconservation.org). To meet these immense energetic needs, megaherbivores depend on plant structural carbohydrates such as cellulose and related components, which are the most abundant organic compounds within terrestrial landscapes (Van Soest, Reference Van Soest1982). However, vertebrates lack cellulolytic enzymes to digest plant fiber. Thus, all terrestrial multicellular herbivores have evolved fermentation chambers housing symbiotic microflora to exploit these abundant resources. The ‘giant fermentation vats’ megaherbivores employ to digest plant fiber have many ecological and biochemical consequences and can influence Earth systems at global scales. We outline some of these processes, including changes in biochemical cycling and gas exchange, as well as nutrient flow across the landscape.
Biogeochemical change
A byproduct of the microbial fermentation of plant fiber in the rumen or cecum is methane, a particularly potent greenhouse gas (Van Soest, Reference Van Soest1982; Clauss and Hummel, Reference Clauss and Hummel2005). Methane production scales positively with body mass (Smith et al., Reference Smith, Elliott and Lyons2010, Reference Smith, Lyons, Wagner and Elliott2015, Reference Smith, Hammond, Balk, Elliott, Lyons, Pardi, Tomé, Wagner and Westover2016b), so larger animals produce more methane than do smaller ones. Moreover, the very largest herbivores, such as those present in the late Pleistocene, may be digestively less efficient and thus experience even greater methanogenesis (Clauss and Hummel, Reference Clauss and Hummel2005). Because atmospheric methane concentrations were lower at the terminal Pleistocene (e.g., ~680 to 700 vs. ~1895 ppbv CH4 today), enteric fermentation was a proportionately greater source to the global pool. Thus, it is probable that the widespread extinction of herbivorous megafauna in the late Pleistocene influenced atmospheric gas exchange and global climate.
Several authors have calculated the enteric production of methane by megafauna at the terminal Pleistocene. For example, using a series of allometric equations relating methane production, geographic range and population density with body mass, Smith and colleagues (Smith et al., Reference Smith, Elliott and Lyons2010, Reference Smith, Hammond, Balk, Elliott, Lyons, Pardi, Tomé, Wagner and Westover2016b) calculated the annual decrease in the methane source pool resulting from the extinction. They found that methane production by late Pleistocene mammals totaled ~139 Tg yr.−1, similar to what livestock contribute to the global budget today (Figure 4A; Smith et al., Reference Smith, Hammond, Balk, Elliott, Lyons, Pardi, Tomé, Wagner and Westover2016b). Importantly, the terminal Pleistocene extinction resulted in an annual loss to the global atmospheric pool of ~69.6 Tg of CH4, a ~35% reduction of the overall tropospheric input at this time (Smith et al., Reference Smith, Hammond, Balk, Elliott, Lyons, Pardi, Tomé, Wagner and Westover2016b). Similar results have been obtained by others. For example, using a bottom-up approach, Zimov and Zimov (Reference Zimov and Zimov2014) calculated that Pleistocene mammals may have contributed 120–170 Tg to the annual global methane budget. Their estimate was based on computations of primary productivity, which they assumed was consumed by vertebrate herbivores.

Figure 4. Changes in methane emissions by wildlife over time. (A) Enteric methane emissions by wild (teal) and domestic (spotted) herbivores. The reduction of CH4 emissions resulting from extinctions or extirpation of animals is indicated in red. Shown are the emissions at the terminal Pleistocene and today. Modern emissions are largely sourced from domesticated livestock. Redrawn after Smith et al. (Reference Smith, Hammond, Balk, Elliott, Lyons, Pardi, Tomé, Wagner and Westover2016b). (B) The isotopic ‘signature’ of methane in ice cores. Drawn after data from Zimov and Zimov (Reference Zimov and Zimov2014). As wildlife has been extirpated across the globe, and more recently, has been replaced by domesticated livestock, there has been a corresponding shift in the sourcing of methane. Because methane is an important greenhouse gas, the amount and sourcing of emissions are important. (C) Methane concentrations from the GISP2 ice core (Brook, Reference Brook2009). Note that there was an abrupt drop in global methane concentration in the atmosphere coincident with the extinction of megafauna at the terminal Pleistocene; this coincided with the onset of the Younger Dryas cold episode. Redrawn after Smith et al. (Reference Smith, Hammond, Balk, Elliott, Lyons, Pardi, Tomé, Wagner and Westover2016b).
This shift in the carbon biogeochemical cycle is evident in the ice core record (Figure 4B, C). Analysis of occluded air bubbles within the GISP2 and EPICA highly resolved ice cores (EPICA, 2004; Brook, Reference Brook2009), reveals an abrupt drop in methane concentrations shortly after humans entered the New World, coincident with the extinction. This methane drop is significantly more abrupt (7–10 times) than other declines over the past 800 ka, suggesting novel contributing mechanisms (Figure 4C; Smith et al., Reference Smith, Hammond, Balk, Elliott, Lyons, Pardi, Tomé, Wagner and Westover2016b). Moreover, there was a significant change in the sourcing of atmospheric methane. The isotopic value (i.e., δ13C and δ2H) of methane varies with sources, meaning that it is possible to determine if a sample is derived from biomass burning, gas clathrates, permafrost, herbivores or boreal/tropical wetlands (Zimov and Zimov, Reference Zimov and Zimov2014). While the Pleistocene isotopic signature suggests megaherbivores were the main contributors to the global pool, early Holocene methane emissions largely originated from the degrading frozen soils of the mammoth steppe biome (Figure 4B; Zimov and Zimov, Reference Zimov and Zimov2014). Hence, it is highly probable these changes in atmospheric methane resulted from the widespread extinction of megafauna.
Interestingly, these decreases in methane concentration are also synchronous with the onset of the Younger Dryas stadial, an abrupt transition ~12.8 ka where climate returned to near glacial conditions in the higher latitudes of the Northern Hemisphere. Did the extirpation of hundreds of millions of large-bodied animals contribute in any way to this climatic event? Although methane is ∼200 times less abundant than carbon dioxide in the atmosphere, its much greater efficiency in trapping radiation leads to a significant role in radiative forcing of climate. How much the decrease in methane contributed to the rapid drop in temperature at the Younger Dryas remains unclear, although simplistic modeling suggests a temperature drop of at least ~0.5°C (Smith et al., Reference Smith, Hammond, Balk, Elliott, Lyons, Pardi, Tomé, Wagner and Westover2016b). The role of large vertebrates in regulating climate through the production of methane may not be confined to mammals; it has been speculated that herbivory by sauropods may have helped maintain warm Mesozoic climates (Wilkinson et al., 2012).
Note that paradoxically, the extinction of megaherbivores in the Americas may also have ultimately contributed to the rise in methane that occurred at the end of the Younger Dryas (Figure 4). As mentioned earlier, the wide-scale habitat alteration from the vast mammoth steppe of the Pleistocene to the more waterlogged habitats of the Holocene has been attributed in part to the absence of grazing by megaherbivores (Zimov et al., Reference Zimov, Chuprynin, Oreshko, Chapin, Reynolds and Chapin1995; Guthrie, Reference Guthrie2001; Johnson, Reference Johnson2009). Heavy grazing not only suppressed woody re-growth (Zimov et al., Reference Zimov, Chuprynin, Oreshko, Chapin, Reynolds and Chapin1995) but may have also stimulated above-ground grass production and high rates of soil moisture transpiration (Zimov et al., Reference Zimov, Chuprynin, Oreshko, Chapin, Reynolds and Chapin1995; Johnson, Reference Johnson2009). In the absence of heavy grazing by megaherbivores, water tables may have risen, leading to a slowdown in the rate of nutrient breakdown and recycling, an increase in organic matter accumulation, and a decrease in soil fertility (Zimov et al., Reference Zimov, Chuprynin, Oreshko, Chapin, Reynolds and Chapin1995; Johnson, Reference Johnson2009); factors promoting increased methane emissions. There is good evidence that the near ubiquitous presence of black mats (a thin layer of organic material created under moist conditions such as elevated water tables, ponds, bogs and wet meadows) across North America dates to just after the megafaunal extinction (Haynes, Reference Haynes2008).
Today of course, megafauna have largely been replaced across the earth by livestock (Smith et al., Reference Smith, Doughty, Malhi, Svenning and Terborgh2016a, Reference Smith, Hammond, Balk, Elliott, Lyons, Pardi, Tomé, Wagner and Westover2016b; Bar-On et al., Reference Bar-On, Phillips and Milo2018). Indeed, as the overall biomass of mammals on the planet has grown fourfold over the past few hundred years, the biomass of wild mammals has decreased by a factor of 6 (Bar-On et al., Reference Bar-On, Phillips and Milo2018). There are now more than 1.5 billion cattle, 1.2 billion sheep, 1 billion goats, >950 million pigs on Earth (Robinson et al., Reference Robinson, Wint, Conchedda, Van Boeckel, Ercoli, Palamara, Cinardi, D’Aietti, Hay and Gilbert2014). In part because of these increases in livestock cultivation and industrialization, the methane concentration in the atmosphere has risen dramatically in recent centuries and is now 162% higher than pre-industrial levels (https://www.noaa.gov/news-release/increase-in-atmospheric-methane-set-another-record-during-2021). Globally, animal agriculture contributed about 30% of human-derived methane emissions in 2021 (https://www.ccacoalition.org/en/resources/global-methane-assessment-full-report). For example, of the 650 million metric tons of carbon equivalent of methane the United States released in 2021, >40% was derived solely from livestock emissions and manure management (https://www.epa.gov/ghgemissions/inventory-us-greenhouse-gas-emissions-and-sinks).
Redistribution of nutrients
As we discussed earlier (see section ‘Distance’), the fossil record for some of the largest Pleistocene megafauna suggests they had virtually continental-wide distributions (FAUNMAP Working Group, 1994; Lyons and Smith, Reference Lyons, Smith, Smith and Lyons2013). This is not surprising because space use is positively related to body size (Brown, Reference Brown1995). For example, even within their vastly restricted modern geographic distribution, elephant home range can reportedly exceed 8,500 to 10,000 km2 (Lindeque and Lindeque, Reference Lindeque and Lindeque1991; Ngene et al., Reference Ngene, Okello, Mukeka, Muya, Njumbi and Isiche2017) and some individuals have been logged traveling more than 2,800 km annually (Mills et al., 2018). As animals travel through the landscape they also engage in activities such as foraging, digestion and ultimately, defecation. Because the passage rate of food through the body is positively associated with body size (Peters, Reference Peters1983), elimination by megaherbivores occurs at some distance from where food was consumed (Doughty et al., Reference Doughty, Roman, Faurby, Wolf, Haque, Bakker, Malhi, Dunning and Svenning2016b; Veldhuis et al., Reference Veldhuis, Gommers, Olff and Berg2018). Moreover, the digestion process liberates nutrients within vegetation that would otherwise be ‘locked’ within plant biomass for many decades (Owen-Smith, Reference Owen-Smith1988; Johnson, Reference Johnson2009). Thus, the extensive lateral movement of megafauna can play a key role in the redistribution of nutrients such as carbon, sodium, phosphorus, and nitrogen and is particularly important in nutrient-poor systems or low-productivity environments (McNaughton et al., Reference McNaughton, Banyikwa and McNaughton1997; Doughty et al., Reference Doughty, Wolf and Malhi2013, Reference Doughty, Roman, Faurby, Wolf, Haque, Bakker, Malhi, Dunning and Svenning2016b, Reference Doughty, Wolf, Baraloto and Malhic; Schmitz et al., Reference Schmitz, Raymond, Estes, Kurz, Holtgrieve, Ritchie, Schindler, Spivak, Wilson, Bradford and Christensen2014; Veldhuis et al., Reference Veldhuis, Gommers, Olff and Berg2018).
Megafauna can also transport nutrients across habitat types. For example, today hippos contribute to the lateral transfer and recycling of silicon by foraging on riverine grasslands, but defecating in rivers and ponds (Schoelynck et al., Reference Schoelynck, Subalusky, Struyf, Dutton, Unzué-Belmonte, Van, Vijver, Post, Rosi, Meire and Frings2019). Conversely, by foraging on aquatic vegetation, but defecating on land, moose transfer aquatic-derived nitrogen to terrestrial ecosystems (Bump et al., Reference Bump, Tischler, Schrank, Peterson and Vucetich2009). The mass drownings associated with the annual migration of wildebeests result in the transfer of carbon, nitrogen and phosphorous from the Serengeti into the Mara River watershed (Subalusky et al., Reference Subalusky, Dutton, Rosi and Post2017). And, brown bears transport marine-derived nutrients to terrestrial environments in areas with large salmon runs (Hilderbrand et al., Reference Hilderbrand, Hanley, Robbins and Schwartz1999). All of these processes significantly alter primary productivity and biodiversity in environments. Such enhancement of biogeochemical cycling is not replicated by small-bodied herbivores who eat less, have shorter passage rates, and much more restricted home ranges.
Several studies have suggested that the extinction of large-bodied mammals in the terminal Pleistocene fundamentally changed the pattern of nutrient deposition on landscapes, leading to declines in the fertility of terrestrial, aquatic and marine environments (Doughty et al., Reference Doughty, Wolf and Malhi2013, Reference Doughty, Roman, Faurby, Wolf, Haque, Bakker, Malhi, Dunning and Svenning2016b, Reference Doughty, Wolf, Baraloto and Malhic). For example, Doughty et al. (Reference Doughty, Wolf, Baraloto and Malhi2016c) explored the role of megafauna in the distribution of sodium on the landscape. Using an allometric-based modeling approach, they found significant differences in the spatial distribution of sodium related to the loss of megafauna. The lack of lateral transport by megafauna has resulted in modern ecosystems with higher sodium concentrations along coastal environments and reduced concentrations inland. Similarly, the fertility of oceans and terrestrial surfaces has likely decreased significantly since the late Pleistocene (Doughty et al., Reference Doughty, Roman, Faurby, Wolf, Haque, Bakker, Malhi, Dunning and Svenning2016b). Using a random walk mathematical formulation, Doughty et al. (Reference Doughty, Roman, Faurby, Wolf, Haque, Bakker, Malhi, Dunning and Svenning2016b) computed that across the earth, the ability of animals to transport nutrients away from a point source has decreased to 6% of its former capacity. Overall, before the late Pleistocene extinction, the long-range movements of large-bodied mammals led to a more fertile planet.
Changes in behavior or foraging patterns can also alter the patchiness of nutrients on landscapes. Because of their large size, megaherbivores are mostly immune to predation and so not unduly influenced by the distribution of predators on the landscape. However, smaller-bodied herbivores do respond to the ‘landscape of fear’ (Brown et al., Reference Brown, Laundré and Gurung1999; Laundré et al., Reference Laundré, Hernández and Altendorf2001) and when predators are present they tend to curtail movement through areas perceived as higher risk (Le Roux et al., Reference Le Roux, Kerley and Cromsigt2018). This leads to concentrations of dung, and hence nutrients, in areas where mesoherbivores aggregate, which tend to be more open and have greater visibility. Thus, in a world without megaherbivores, there is limited nutrient diffusion away from these fear-driven prey aggregations (Le Roux et al., Reference Le Roux, Kerley and Cromsigt2018).
Surviving mammals
The size-selective nature of the late Pleistocene extinction meant that energy flow within the community was fundamentally altered. An assumption underlying ecological theory is that energy does not go unused (Ernest and Brown, Reference Ernest and Brown2001), so this suggests that surviving species had a number of potential options – they could persist without change, alter their diet and/or morphology to better exploit newly available resources and/or become more abundant or widespread in the landscape. It is also possible that other taxonomic groups co-opted the energy that once flowed through megafauna, or that the ‘excess energy’ in the ecosystem allowed invasive species to establish. Here, we explore potential dietary, morphological and distributional changes in the surviving mammals.
Dietary changes
When species are lost, ecological vacancies are created, which presents opportunities for survivors. In perhaps the most famous example, extinction of the non-avian dinosaurs ~65 Ma allowed mammals to expand into the ecological niches left vacant and ultimately led to their radiation and the many mammalian forms we see today (Alroy, Reference Alroy1998; Smith et al., Reference Smith, Boyer, Brown, Costa, Dayan, Ernest, Evans, Fortelius, Gittleman, Hamilton and Harding2010; Grossnickle et al., Reference Grossnickle, Smith and Wilson2019). The loss of megafauna in the Americas at the terminal Pleistocene may also have provided many ecological opportunities for surviving species (Martin and Klein, Reference Martin and Klein1984; Barnosky et al., Reference Barnosky, Koch, Feranec, Wing and Shabel2004; Lyons et al., Reference Lyons, Smith and Brown2004, Reference Lyons, Miller, Fraser, Smith, Boyer, Lindsey and Mychajliw2016a; Koch and Barnosky, Reference Koch and Barnosky2006; Smith et al., Reference Smith, Doughty, Malhi, Svenning and Terborgh2016a, Reference Smith, Elliott Smith, Villaseñor, Tomé, Lyons and Newsome2022; Tóth et al., Reference Tóth, Lyons, Barr, Behrensmeyer, Blois, Bobe, Davis, Du, Eronen, Faith and Fraser2019). In this section, we explore whether the extinction led to dietary shifts and/or expansion in surviving mammals. Did the survival of herbivore and carnivore species fill the roles left by the loss of their larger counterparts? We focus on inferences determined from geochemical proxies, namely stable isotope analysis (Box 1), which has rapidly become a common method to examine the present and past ecology of animal populations (e.g., Newsome et al., Reference Newsome, Martinez del Rio, Bearhop and Phillips2007; Clementz, Reference Clementz2012; Layman et al., Reference Layman, Araujo, Boucek, Hammerschlag‐Peyer, Harrison, Jud, Matich, Rosenblatt, Vaudo, Yeager and Post2012; Koch et al., Reference Koch, Fox-Dobbs and Newsome2017).
Box 1. Stable isotope analysis.
Stable isotope analysis involves measuring the relative abundances of different stable isotopes in an organic sample, such as the ratio of 13C:12C or 15N:14N. Note that these measurements are distinct from radiogenic dating methods which rely on the decay of unstable isotopes such as 14C. Stable isotope ratios are reported in delta values – δ – defined in units of parts per thousand, or per mil (‰) (Sharp, Reference Sharp2017). All samples and reference materials are calibrated against international standards to allow comparison among laboratories and analytical methods. The accepted standards for the two most commonly measured isotope systems in biological studies – δ 13C and δ 15N – are Vienna-Pee Dee Belemnite (VPDB) and atmospheric N2, respectively.
The utility of a stable isotope methodology in biology and ecology relies on a few key observations. Firstly, carbon isotope values (δ 13C) vary among different types of primary producers due to differences in photosynthetic mechanisms, biochemistry and growth rates (Fogel and Cifuentes, Reference Fogel, Cifuentes, Engel and Macko1993). In terrestrial environments, plants using C4 photosynthesis (e.g., grasses, corn, sugarcane and many monocots) have higher δ 13C values by 10–15‰ relative to plants with the C3 photosynthetic pathway (e.g., trees, rice, most dicots; Ehleringer and Cerling, Reference Ehleringer and Cerling2002). Consequently, δ 13C values in consumers provide a record of habitat use and of browse versus graze in extinct and extant animals (Ehleringer and Cerling, Reference Ehleringer and Cerling2002). δ 15N values of consumers can be used to understand relative trophic positioning, as 14N is preferentially excreted (Vanderklift and Ponsard, Reference Vanderklift and Ponsard2003), and so δ 15N values tend to increase with increasing consumer trophic level.
One important consideration is that both carbon and nitrogen values at the base of the food web are strongly influenced by climatic variables (Austin and Vitousek, Reference Austin and Vitousek1998). Nonetheless, isotopic analysis allows researchers to characterize both the overall structure of local food webs as well as the flows of energy from basal production sources through to top consumers as long as these factors are considered (Ben-David and Flaherty, Reference Ben-David and Flaherty2012).
In paleoecological or zooarchaeological studies, taphonomy must also be considered, as original tissue must remain in order to infer diet/ecology of ancient animals through stable isotope analysis. Clementz (Reference Clementz2012) and Koch et al. (Reference Koch, Fox-Dobbs and Newsome2017) present useful summaries of substrates that can be used for isotopic analysis of vertebrate paleoecology. Of these, collagen extracted from bone or dentine is among the most widely used, as this substrate contains carbon and nitrogen which can be measured for stable isotope ratios and used to assess protein diagenesis via evaluation of [C]:[N] atomic ratios (Ambrose, Reference Ambrose1990).
As noted elsewhere, large-bodied herbivores were the most effected by the late Pleistocene extinction (Lyons et al., Reference Lyons, Smith and Brown2004; Smith et al., Reference Smith, Elliott Smith, Lyons and Payne2018). In North America, survivors included pronghorn (Antilocapra americana), several cervids (Odocoileus virginianus, Odocoileus hemionus, Rangifer tarandus, Alces alces, Cervus elaphus), bovids (such as Ovis canadensis, Oreamnos americanus and Ovibos moschatus) and a smaller species of bison (Bison bison). The next largest surviving herbivore, at just over 30 kg, was a peccary (Smith et al., Reference Smith, Brown, Haskell, Lyons, Alroy, Charnov, Dayan, Enquist, Morgan Ernest, Hadly and Jones2004, Reference Smith, Elliott Smith, Lyons, Payne and Villaseñor2019). With the loss of so many competitors, resource and habitat availability likely increased dramatically. As the majority of extinct taxa were grazers on C4 vegetation (Smith et al., Reference Smith, Elliott Smith, Villaseñor, Tomé, Lyons and Newsome2022), leaving this guild particularly depauperate, there was great opportunity for ecological expansion into a C4 grazing niche by surviving species.
Despite this, little evidence exists for dietary shifts among the surviving large-bodied herbivores. For example, there was no change in the mean δ 13C value of bison (B. bison), deer (Odocoileus sp.) or antelope (A. americana) following the extinction in central Texas (Smith et al., Reference Smith, Elliott Smith, Villaseñor, Tomé, Lyons and Newsome2022). Thus, somewhat paradoxically, surviving herbivore species apparently did not take advantage of the vacant ecological/resource space left by the extinct megafauna. However, while the extinction led to a disproportionate loss of grazers (Smith et al., 2016), multiple browsers (e.g., mastodon and flat-headed peccary) and mixed feeders (e.g., camels and gomphotheres) were also lost, which likely reduced the competitive pressure on survivors across all herbivore foraging guilds. Additionally, C4 grazers in central Texas likely fed predominantly on grasses (Smith et al., Reference Smith, Elliott Smith, Villaseñor, Tomé, Lyons and Newsome2022), which are difficult to digest and require specialized physiology, such as foregut fermentation, hypsodonty dentition, increased gut retention times and overall larger gut capacity (Chivers, Reference Chivers1994; Stevens and Hume, Reference Stevens and Hume2004; Janis, Reference Janis, Gordon and Prins2008). This may have prevented or slowed surviving browser species from switching/expanding their diet. In contrast, grazers may be more readily able to switch to less recalcitrant browse (Bergmann et al., Reference Bergmann, Craine, Robeson and Fierer2015; Craine, Reference Craine2021; Pardi and DeSantis, Reference Pardi and DeSantis2021). Indeed, morphological studies suggest bison were more ecologically flexible in the late Pleistocene than their modern congeners today (Rivals et al., Reference Rivals, Solounias and Mihlbachler2007; Rivals and Semprebon, Reference Rivals and Semprebon2011; Kelly et al., Reference Kelly, Miller, Wooller, Seaton, Druckenmiller and DeSantis2021).
Among small herbivores, a group that emerged from the Pleistocene–Holocene transition largely unscathed, isotopic paleoecology studies demonstrate a high level of adaptive capacity and mixed responses to the extinction (Tomé et al., Reference Tomé, Elliott Smith, Lyons, Newsome and Smith2020, Reference Tomé, Lyons, Newsome and Smith2022; Smith et al., Reference Smith, Elliott Smith, Villaseñor, Tomé, Lyons and Newsome2022). For example, in central Texas, several herbivorous rodents – the cotton rat (Sigmodon hispidus) and woodrat (Neotoma sp.) – responded quite differently to the Pleistocene–Holocene transition (Figure 5). The δ 13C values of S. hispidus tracked the relative proportion of local grazers, frugivores and granivores, as well as minimum temperature (Tomé et al., Reference Tomé, Elliott Smith, Lyons, Newsome and Smith2020). However, isotopic values of Neotoma predominantly tracked climatic and vegetational changes (Tomé et al., Reference Tomé, Lyons, Newsome and Smith2022); there was also likely a species replacement from N. floridana to Neotoma albigula/micropus as Texas habitats shifted toward more warm and arid environmental conditions. Among lagomorphs, mean δ 13C values of both Lepus sp. and Sylvilagus sp. increased significantly following the extinction, a finding consistent with greater consumption of C4 resources (Figure 5; Smith et al., Reference Smith, Elliott Smith, Villaseñor, Tomé, Lyons and Newsome2022). In sum, trends demonstrate the complex responses by animals to the multiple effects of the megafauna extinction and highlight the varying ecological adaptations of both large and small mammals.
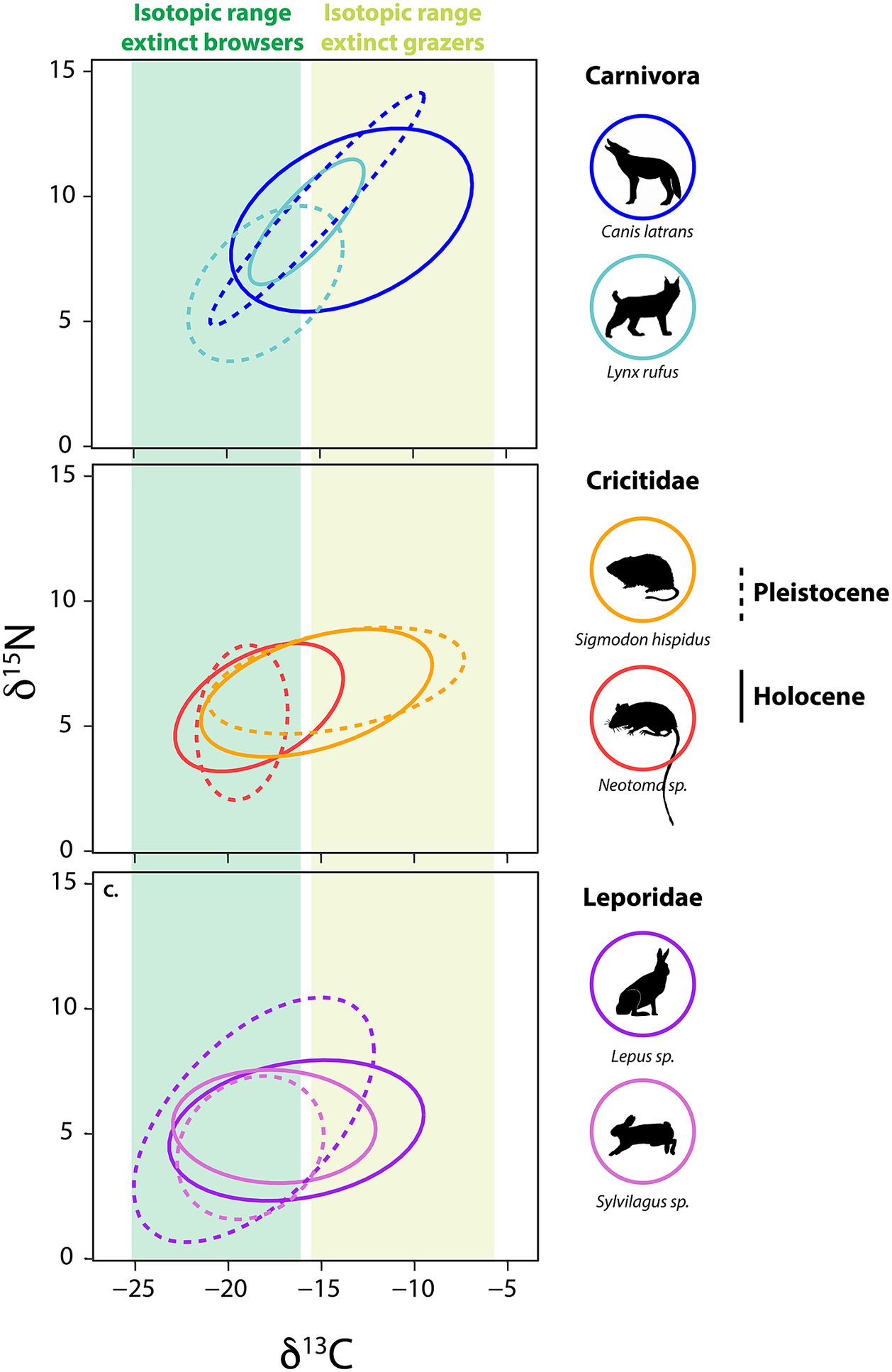
Figure 5. Bulk δ13C and δ15N values from bone collagen of mammalian survivors pre- and post- the terminal Pleistocene megafauna extinction. Data from Smith et al. (Reference Smith, Elliott Smith, Villaseñor, Tomé, Lyons and Newsome2022) and references therein. Ellipses represent the bivariate isotopic space occupied by each group (function stat_ellipseProgram R). Dashed lines show isotopic niche space of species prior to the extinction event, solid lines show isotopic niche post-extinction in the Holocene. Colored bars show the range of δ13C values of extinct grazers and browsers. Animal silhouettes come from Phylopic; illustration credit to Nina Skinner, C. Camilo Julián-Caballero and Gabriela Palomo-Munoz.
Differential dietary responses to the late Pleistocene extinction have also been observed in carnivore guilds, which likely relate to differences in ecological specialization. It appears that carnivores with broader diets had better outcomes (Galetti et al., Reference Galetti, Moleón, Jordano, Pires, Guimaraes, Pape, Nichols, Hansen, Olesen, Munk and de Mattos2018; Smith et al., Reference Smith, Elliott Smith, Villaseñor, Tomé, Lyons and Newsome2022), which may explain the large dietary breadth found among most carnivores today. Among the Canidae, neither coyotes (Canis latrans), or wolves (Canis lupus) in central Texas exhibited changes in their isotopic niche following the extinction (Smith et al., Reference Smith, Elliott Smith, Villaseñor, Tomé, Lyons and Newsome2022). Similar results were found for gray wolves in the Yukon Territory of Canada (Landry et al., Reference Landry, Kim, Trayler, Gilbert, Zazula, Southon and Fraser2021), although DeSantis et al. (Reference DeSantis, Crites, Feranec, Fox-Dobbs, Farrell, Harris, Takeuchi and Cerling2019) reported changes in coyote foraging behavior following the megafauna extinction in coastal southern California. In the central Texas community, coyotes and wolves consistently displayed wide ranges in measured isotope values, suggesting intrinsically high degrees of ecological and dietary plasticity in both the Pleistocene and Holocene (Smith et al., Reference Smith, Elliott Smith, Villaseñor, Tomé, Lyons and Newsome2022). In contrast, fossils of the extinct dire wolf, Aenocyon (Canis) dirus, from the same region exhibited a narrower isotopic niche space (Smith et al., Reference Smith, Elliott Smith, Villaseñor, Tomé, Lyons and Newsome2022). This dietary (isotopic) variability in coyotes and C. lupus may have buffered these species from extinction risk, but also made it difficult to detect any directional changes in diet. Similarly, no ecological shifts were noted in mesocarnivores (e.g., Mephitidae and Procyonidae) or bears (Ursidae) following the extinction (Smith et al., Reference Smith, Elliott Smith, Villaseñor, Tomé, Lyons and Newsome2022).
In contrast, the megafauna extinction led to highly significant isotopic changes within central Texas Felidae (Smith et al., Reference Smith, Elliott Smith, Villaseñor, Tomé, Lyons and Newsome2022). In the Pleistocene, the two sabertooth cats (Homotherium serum and Smilodon fatalis) were apex carnivores. Both sabertooth cats had high δ 13C and δ 15N values and fairly narrow isotopic ranges indicative of specialization on large grazers (Smith et al., Reference Smith, Elliott Smith, Villaseñor, Tomé, Lyons and Newsome2022). Enamel δ 13C values from H. serum and S. fatalis found in La Brea, California also suggest a high degree of dietary specialization (DeSantis et al., Reference DeSantis, Crites, Feranec, Fox-Dobbs, Farrell, Harris, Takeuchi and Cerling2019, Reference DeSantis, Feranec, Antón and Lundelius2021). Following the extinction of these large cats in Texas, the surviving felids shifted in bivariate stable isotope space (Smith et al., Reference Smith, Elliott Smith, Villaseñor, Tomé, Lyons and Newsome2022). Lynx (Lynx rufus) and jaguar (Panthera onca) exhibited higher δ 13C values following the extinction with L. rufus also having higher δ 15N values in the Holocene (Figure 5). P. onca became the new apex predator in the system, taking over what was left of the ecological niche space once occupied by the extinct cats. The mountain lion (Puma concolor) also appears in the community by the early Holocene. These findings support the idea of ‘mesopredator release’ (i.e., the expansion in diet, range and/or abundance of a smaller predator following the reduction or removal of a larger congener; Soulé et al., Reference Soulé, Bolger, Alberts, Wrights, Sorice and Hill1988; Polis et al., Reference Polis, Myers and Holt1989) within the Felidae. However, the ecological role of the surviving predators was likely vastly different from the megacarnivores they ‘replaced’. Indeed, because these new apex predators were so much smaller, they would have fed on smaller and different prey (Carbone et al., Reference Carbone, Mace, Roberts and Macdonald1999; Hayward, Reference Hayward and Kerley2005, Reference Hayward, Henschel, O’Brien, Hofmeyr, Balme and Kerley2006, Reference Hayward, Kamler, Montgomery, Newlove, Rostro-García, Sales and VanValkenburgh2016).
The dietary responses of surviving species to the ecological vacancies left by the extinction of megafauna were clearly multifaceted and varied widely depending on trophic level and dietary guild. Upon reflection, this result is unsurprising, as food webs are complex, highly localized systems held in a delicate balance by direct and indirect interactions among species (e.g., Chesson and Kuang, Reference Chesson and Kuang2008. It is also important to note that the patterns we describe here are based on a handful of studies and, in some cases, a handful of data points. Broad trophic guild categorizations like carnivore, grazer and browsers, often do not adequately capture the dietary complexity of many taxa, particularly when sample sizes are small. Thus, we encourage our colleagues, or any aspiring historical or paleoecologist, to employ a geochemical (i.e., stable isotope analysis; Box 1) perspective to ask similar questions in other localities in North and South America (see Smiley et al., Reference Smiley, Cotton, Badgley and Cerling2016; Terry, Reference Terry2018; Taylor, Reference Taylor2019). Interesting macroecological patterns in species responses may emerge with additional datasets, as will a more comprehensive understanding of the full range of ecological plasticity in our extant mammalian fauna.
Morphological changes
It is generally recognized that body mass is the most important trait of an animal (Thompson, Reference Thompson1942; Bonner, Reference Bonner2006; Smith and Lyons, Reference Smith and Lyons2011, Reference Smith and Lyons2013; Smith et al., Reference Smith, Payne, Heim, Balk, Finnegan, Kowalewski, Lyons, McClain, McShea, Novack-Gottshall and Anich2016d; Smith, Reference Smith2021). The body size of a mammal is not only highly heritable (Smith et al., Reference Smith, Brown, Haskell, Lyons, Alroy, Charnov, Dayan, Enquist, Morgan Ernest, Hadly and Jones2004), but also strongly influences all biological rates and processes, including the essential activities of metabolism, reproduction and growth (Peters, Reference Peters1983; Calder, Reference Calder1984; Schmidt-Nielsen, Reference Schmidt-Nielsen1984). Because of the critical role body size plays in governing the physiology, life history, behavior and ecology of animals, it mediates how they interact with other animals and their environment (Smith, Reference Smith2021).
Morphological shifts are a common response to changing environmental conditions across spatial gradients and over evolutionary time (James, Reference James1970; Smith et al., Reference Smith, Betancourt and Brown1995; Smith and Betancourt, Reference Smith and Betancourt1998, Reference Smith and Betancourt2003, Reference Smith and Betancourt2006; Millien et al., Reference Millien, Lyons, Olson, Smith, Wilson and Yom‐Tov2006; Gardner, Reference Gardner2011; Secord et al., Reference Secord, Bloch, Chester, Boyer, Wood, Wing, Kraus, McInerney and Krigbaum2012; Balk et al., Reference Balk, Betancourt and Smith2019) and are also influenced by ecological factors such as competition (e.g., Hutchinson and MacArthur, Reference Hutchinson and MacArthur1959; Peters, Reference Peters1983). Certainly, body size plays an important role today in structuring mammal communities in Africa (De Iongh et al., Reference De Iongh, De Jong, Van Goethem, Klop, Brunsting, Loth and Prins2011). As new niches opened up following the megafauna extinction, one response may have been for the surviving lineages of smaller-bodied animals to become larger so as to better exploit – either physiologically or ecologically – these newly available resources. Thus, here we explore shifts in body size among survivors of the late Pleistocene megafauna extinction. Body size is fairly easy to characterize with fossils because virtually all cranial and postcranial elements scale with mass in mammals (e.g., Damuth and MacFadden, Reference Damuth and MacFadden1990; Smith, Reference Smith2021; Smith et al., Reference Smith, Elliott Smith, Villaseñor, Tomé, Lyons and Newsome2022).
While considerable work has focused on the morphological responses of animals to the climate changes of the late Quaternary (e.g., Smith et al., Reference Smith, Betancourt and Brown1995; Smith and Betancourt, Reference Smith and Betancourt1998, Reference Smith and Betancourt2003, Reference Smith and Betancourt2006; Barnosky et al., Reference Barnosky, Hadly and Bell2003; MacDonald et al., Reference MacDonald, Bennett, Jackson, Parducci, Smith, Smol and Willis2008; Gardner, Reference Gardner2011; Hoffman and Sgrò, Reference Hoffman and Sgrò2011), few studies to date have explicitly examined how biodiversity loss at the terminal Pleistocene may have driven body size changes (but see Tomé et al., Reference Tomé, Elliott Smith, Lyons, Newsome and Smith2020a, Reference Tomé, Lyons, Newsome and Smith2022; Smith et al., Reference Smith, Elliott Smith, Villaseñor, Tomé, Lyons and Newsome2022). At our Hall’s Cave site in central Texas, we find no consistent pattern in morphological responses to the biodiversity loss at the late Pleistocene. Indeed, the shifts in morphology from the Pleistocene to Holocene were quite species-specific, with some animals (i.e., deer and jackrabbits) becoming significantly larger, and others significantly smaller (i.e., bison and cottontails), and still others exhibiting no change in body size at all (i.e., pronghorn, raccoons and coyotes; Smith et al., Reference Smith, Elliott Smith, Villaseñor, Tomé, Lyons and Newsome2022). These results suggest that most species were responding to other factors, and that if they responded to biodiversity loss, it was in other ways – perhaps through changes in abundance or distribution (see section ‘Changes in distribution and abundance’).
Interesting exceptions include several small mammal taxa that did exhibit changes in morphology associated with the extinction. For example, changes in community structure (i.e., alpha and beta diversity, proportion of each tropic guild) were associated with changes in body size and diet in the hispid cotton rat, S. hispidus. Prior to the extinction when the community had many grazers, insectivores and browsers, cotton rats were smaller. As the community composition shifted toward a greater proportion of browsers and omnivores, the body size of cotton rats increased. The changes in morphology seen over this time may reflect shifts in resource availability (Tomé et al., Reference Tomé, Elliott Smith, Lyons, Newsome and Smith2020a). But body size responses were not consistent across small-bodied taxa. While Sigmodon was quite sensitive to community turnover and associated vegetation change, the morphology of other small-bodied rodents such as Neotoma were strongly correlated to climate change at the site and not biodiversity (Tomé et al., Reference Tomé, Elliott Smith, Lyons, Newsome and Smith2020a, Reference Tomé, Lyons, Newsome and Smith2022). As yet, we have not characterized responses by the entire small-bodied mammal guild, so it is unclear whether patterns may yet prove to be consistent along trophic affiliation, body size or other ecological gradients. Such individualistic body size responses to biodiversity loss demonstrate the complex nature of communities and ecological interactions within them. Future work should investigate whether there is a regularity to how taxa respond morphologically to biodiversity loss across a spectrum of body sizes (i.e., shrews to deer), and habitat and resource preferences (i.e., deserts, grasslands and forest).
Changes in distribution and abundance
Range shifts were common during the late Pleistocene and Holocene (Grayson, Reference Grayson1987, Reference Grayson2000; Graham et al., Reference Graham, Lundelius, Graham, Schroeder, Toomey, Anderson, Barnosky, Burns, Churcher, Grayson and Guthrie1996; Lyons, Reference Lyons2003). Because the geographic distribution is a dynamic reflection of a taxon’s realized ecological niche, which is constrained by physiological tolerances to the abiotic environment as well as interactions with other species (Grinnell, Reference Grinnell1917; Hutchinson, Reference Hutchinson1957; Loehle and LeBlanc, Reference Loehle and LeBlanc1996), it is particularly sensitive to changes in abiotic and biotic factors (Brown et al., Reference Brown, Stevens and Kaufman1996). The late Pleistocene megafauna extinctions were accompanied by significant climate change meaning that both factors may have influenced range shifts (Grayson, Reference Grayson1987, Reference Grayson2000; Graham et al., Reference Graham, Lundelius, Graham, Schroeder, Toomey, Anderson, Barnosky, Burns, Churcher, Grayson and Guthrie1996; Lyons, Reference Lyons2003).
Quantification of mammal species range shifts across the Pleistocene–Holocene transition found that the centroid of species ranges shifted in different directions, and to different extents; moreover, the magnitude of the change in range size differed among species (Lyons, Reference Lyons2003). These differences highlight the individual responses of species to ecological change, as evidenced in both body size and diet shifts (see sections ‘Dietary changes’ and ‘Morphological changes’). We can see this clearly at Hall’s Cave. Currently, this site lies outside of the modern geographic range of margay (Leopardus wiedii), white-throated woodrat (N. albigula), Southern bog lemming (Synaptomys cooperi), meadow jumping mouse (Zapus hudsonius), the common shrew (Sorex cinereus), prairie shrew (Sorex haydeni), Southeastern shrew (Sorex longirostris) and the Southern short-tailed shrew (Blarina carolinensis), yet all these species co-occurred during the Pleistocene (Smith et al., Reference Smith, Tomé, Elliott Smith, Lyons, Newsome and Stafford2016c). Examination of the fossil record of two of these species (S. cooperi and B. carolinensis; Figure 6), shows that while neither are found together today (and indeed, they have quite distinct distributions), they were both present at Hall’s Cave in the Pleistocene. Over the Holocene, S. cooperi shifted the southern boundary of its geographic range further north, while the western boundary of B. carolinensis shifted east.
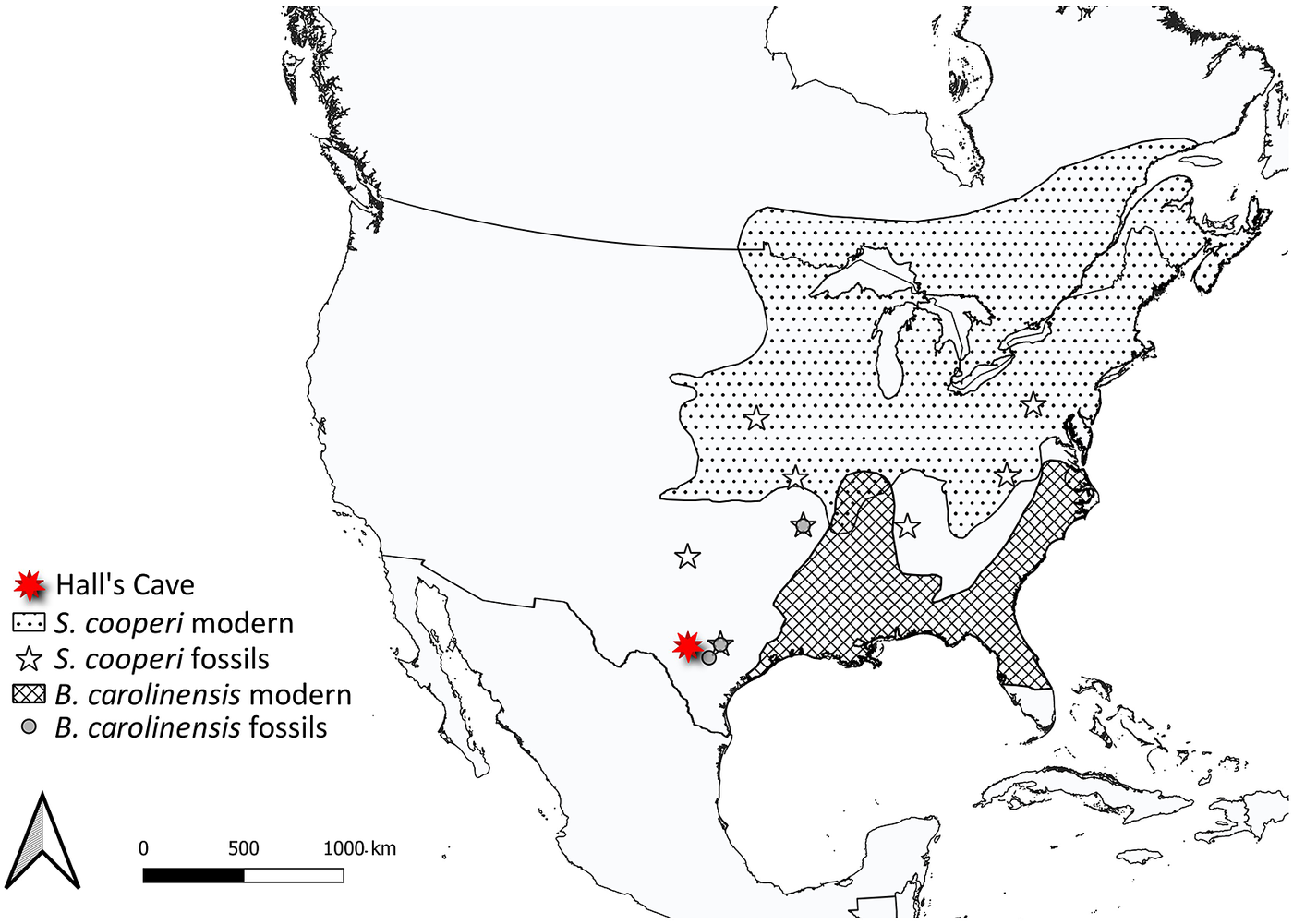
Figure 6. Fossil localities and modern distributions of Synaptomys cooperi and Blarina carolinensis; examples of species found outside of their modern geographic distribution at Hall’s Cave. These species occurred at Hall’s Cave in the past and have changed their respective geographic ranges individualistically. Late Quaternary fossil occurrences come from the Neotoma database (Williams et al., Reference Williams, Grimm, Blois, Charles, Davis, Goring, Graham, Smith, Anderson, Arroyo-Cabrales and Ashworth2018) with modern distributions superimposed (Marsh et al., Reference Marsh, Sica, Burgin, Dorman, Anderson, del, Mijares, Vigneron, Barve, Dombrowik, Duong and Guralnick2022).
To what extent were such shifts in the geographic range of mammals driven by biodiversity loss? Niche modeling of late Pleistocene and early Holocene distributions indicates that climate tracking drove some, but not all, of the observed changes, suggesting a role for biotic interactions (Martínez-Meyer et al., Reference Martínez-Meyer, Peterson and Hargrove2004; Veloz et al., Reference Veloz, Williams, Blois, He, Otto‐Bliesner and Liu2012; Maguire et al., Reference Maguire, Nieto-Lugilde, Fitzpatrick, Williams and Blois2015; Pardi and Smith, Reference Pardi and Smith2016). At the end of the Pleistocene, the largest surviving herbivores lived in communities with fewer competitors and fewer large predators; thus, changing biotic interactions likely impacted range shifts. Herbivores respond to resource availability, but they also compete with one another and increase vigilance and avoid areas where they are susceptible to predation (Brown et al., Reference Brown, Laundré and Gurung1999). The presence of large predators influences herbivore density and their use of the landscape, and thus can affect herbivory pressure and plant communities (Ripple et al., Reference Ripple, Larsen, Renkin and Smith2001; Ripple and Beschta, Reference Ripple and Beschta2003). A macroecological approach assessing carnivore body size and maximum prey size (Van Valkenburgh et al., Reference Van Valkenburgh, Hayward, Ripple, Meloro and Roth2016) demonstrated that large Pleistocene carnivores could have hunted megaherbivores and effectively limited their populations. This enhanced landscape of fear would have been felt throughout ecosystems, and top-down forces likely had strong impacts on the abundance and distributions of some species (Ripple and Van Valkenburgh, Reference Ripple and Van Valkenburgh2010).
The megafauna extinction brought with it the loss of interactions with large competitors. Carnivores experience strong intraguild interactions; they not only compete but also engage in frequent intraguild killing where the larger species is typically dominant (Polis, Reference Polis1981; Polis et al., Reference Polis, Myers and Holt1989). Competition between carnivores and the ability to defend territory are strong forces dictating geographic distributions, to the extent that smaller carnivores will avoid the territories of larger competitors (Polis et al., Reference Polis, Myers and Holt1989; Fedriani et al., Reference Fedriani, Fuller, Sauvajot and York2000; Aunapuu et al., Reference Aunapuu, Oksanen, Oksanen and Korpimaki2010). As a result, the extinction of the very largest carnivores at the end of the Pleistocene likely changed these interactions (see section ‘Dietary changes’), and impacted the geographic distribution of surviving carnivore species. For example, three large canid morphotypes existed at the end of the Pleistocene (i.e., dire wolves, gray wolves and Beringian wolves) and partitioned the landscape, at least in part, through competitive exclusion (Dundas, Reference Dundas1999; Meachen et al., Reference Meachen, Brannick and Fry2016). Ecological niche modeling has demonstrated that the geographic ranges of surviving Canidae did not track climate change following the extinction (Pardi and Smith, Reference Pardi and Smith2016). Instead, changing interactions, such as release from intraguild competition with congeners, was important. Body size played a role in these changes as the ranges of the largest survivors, wolves and coyotes, deviated the most from climate predictions; in contrast, the smallest survivors, foxes, more closely tracked Holocene climate shifts (Pardi and Smith, Reference Pardi and Smith2016).
Another probable response to the biodiversity loss in the late Pleistocene was numerical; taxa may have increased in abundance to exploit newly available resources. The absence of millions of large-bodied mammals on the landscape left many potentially vacant ecological niches. However, abundance is notoriously difficult to characterize in the fossil record because of taphonomic biases and thus little work has explored this. Interestingly, recent modeling efforts of bison – the largest bovid that survived the extinction in North America – suggests intriguing changes in the abundance and distribution of bison herds over the late Pleistocene and Holocene (Martin et al., Reference Martin, Short, Plumb, Markewicz, Van Vuren, Wehus-Tow, Otarola-Castillo and Hill2022; Wendt et al., Reference Wendt, McWethy, Widga and Shuman2022). Prior to the megafauna extinction, animals were widely scattered across North America into regional clusters. This changed by the late Holocene when the bison were spatially contiguous through much of central and western North America (Wendt et al., Reference Wendt, McWethy, Widga and Shuman2022). While the authors attribute much of the shifts in both distribution and abundance of bison herds to tracking of favorable environmental conditions, they do note a spike in abundance at the terminal Pleistocene and early Holocene (Wendt et al., Reference Wendt, McWethy, Widga and Shuman2022). These increases in distribution and abundance in the early Holocene may have represented expansion due to the loss of competitors (Wendt et al., Reference Wendt, McWethy, Widga and Shuman2022).
Species adaptations and range shifts are highly individualistic responses to biodiversity loss and environmental change; taking both possibilities into consideration demonstrates the complex nature of communities and ecological interactions within them. To understand how animal morphology may respond to extinction, future work needs to examine responses across a spectrum of body sizes (i.e., shrews to deer), and habitat and resource preferences (i.e., deserts, grasslands and forest). Further advances in occupancy modeling should allow the characterization of abundance shifts in species.
Changes in communities and ecosystems
Modern studies demonstrate that the extirpation of the largest animals in a community profoundly changes the ecological interactions and behavior of surviving animals and the energy flow through the ecosystem (Estes et al., Reference Estes, Terborgh, Brashares, Power, Berger, Bond, Carpenter, Essington, Holt, Jackson and Marquis2011); thus, the late Pleistocene extinctions likely led to similar perturbations. These might include shifts in biotic interactions (i.e., competition and predation), food webs and aspects of community structure such as co-occurrence patterns and functional diversity. We discuss changes in ecological interactions among taxa and the shifts in the structure of ecological communities below. Where possible, we use Hall’s Cave as a case study to contextualize patterns found at other sites and scales.
Ecological interactions within communities
The loss of megafauna led to profound changes in ecological interactions within communities. Not only were some biotic interactions entirely lost when both species were extirpated, but many interactions also involving surviving species and (now) extinct ones were also lost. This led to a cascade of effects that percolated throughout the community and included changes to the connectivity and stability of food webs (Nenzen et al., Reference Nenzen, Montoya and Varela2014; Pires et al., Reference Pires, Koch, Fariña, de Aguiar, dos Reis and Guimarães2015; Fricke et al., Reference Fricke, Hsieh, Middleton, Gorczynski, Cappello, Sanisidro, Rowan, Svenning and Beaudrot2022). We discuss these below.
Species interaction networks and food webs
The loss of megafauna from ecosystems had numerous and diverse effects on species interactions. The most obvious, of course, was the loss of biotic interactions between megafauna and other mammals, which resulted in food webs with fewer connections between taxa (Nenzen et al., Reference Nenzen, Montoya and Varela2014; Fricke et al., Reference Fricke, Hsieh, Middleton, Gorczynski, Cappello, Sanisidro, Rowan, Svenning and Beaudrot2022). However, the effects were exacerbated because of the size-selectivity of the extinction. This is because the reduction of connectivity in food webs is not directly proportional to biodiversity loss; large-bodied mammals form more links per species than do smaller-bodied mammals (Sinclair et al., Reference Sinclair, Mduma and Brashares2003; Owen-Smith and Mills, Reference Owen-Smith and Mills2008; Pires et al., Reference Pires, Koch, Fariña, de Aguiar, dos Reis and Guimarães2015; Fricke et al., Reference Fricke, Hsieh, Middleton, Gorczynski, Cappello, Sanisidro, Rowan, Svenning and Beaudrot2022). Thus, the disproportionate extirpation of large-bodied mammals in the late Pleistocene meant food webs lost many more links than expected (Fricke et al., Reference Fricke, Hsieh, Middleton, Gorczynski, Cappello, Sanisidro, Rowan, Svenning and Beaudrot2022). Current biodiversity loss is continuing this trend; the extinction of remaining megafauna will have an overwhelming effect on Earth’s already depauperate food webs (Fricke et al., Reference Fricke, Hsieh, Middleton, Gorczynski, Cappello, Sanisidro, Rowan, Svenning and Beaudrot2022).
The reorganization of food webs began after human arrival on a continent and intensified after the start of European colonization and the industrial revolution (Fricke et al., Reference Fricke, Hsieh, Middleton, Gorczynski, Cappello, Sanisidro, Rowan, Svenning and Beaudrot2022). For example, despite being robust to secondary extinctions for 850,000 years, extinctions significantly altered mammal food webs in Iberia in the Holocene (Nenzen et al., Reference Nenzen, Montoya and Varela2014). The loss of large-bodied mammals led to less robust food webs, which were less resilient to future extinction (Nenzen et al., Reference Nenzen, Montoya and Varela2014; Pires et al., Reference Pires, Koch, Fariña, de Aguiar, dos Reis and Guimarães2015). Moreover, the type of mammal lost matters; large predators tend to have broad dietary niches and interact with a large number of other species. Thus, they have higher per capita interaction strengths than large herbivores or smaller predators. As a result, the addition of a large novel predator like humans had a greater effect in communities with a diverse predator guild such as the late Pleistocene of North America (Pires et al., Reference Pires, Koch, Fariña, de Aguiar, dos Reis and Guimarães2015). South American communities had fewer large predators relative to their large herbivore guild (Fariña, Reference Fariña1996; Fariña et al., Reference Fariña, Vizcaíno and De Iuliis2013). Not surprisingly, then, the greater proportion of carnivore extinctions in North America led to a greater decrease in stability than in South America (Pires et al., Reference Pires, Koch, Fariña, de Aguiar, dos Reis and Guimarães2015). African communities underwent a reduction in large carnivore diversity earlier in the Pleistocene, arguably due to competition with early Homo species (Lewis and Werdelin, Reference Lewis, Werdelin, Bobe, Alemseged and Behrensmeyer2007; Werdelin, Reference Werdelin2012; Werdelin and Lewis, Reference Werdelin and Lewis2013) leaving modern African communities with a relatively depauperate large predator guild. Hence, present day African communities are more robust to the extinction of a similar magnitude (Pires et al., Reference Pires, Koch, Fariña, de Aguiar, dos Reis and Guimarães2015).
Another reason the terminal Pleistocene extinction left such lasting effects on modern mammalian food webs is because of the unique functional roles of the species that went extinct (see section ‘Functional changes within communities’ on functional diversity). Because large-bodied herbivores are often ecosystem engineers (Owen-Smith, Reference Owen-Smith1988), their loss has cascading effects on surviving species (e.g., Estes et al., Reference Estes, Terborgh, Brashares, Power, Berger, Bond, Carpenter, Essington, Holt, Jackson and Marquis2011; Ripple et al., Reference Ripple, Newsome, Wolf, Dirzo, Everatt, Galetti, Hayward, Kerley, Levi, Lindsey and Macdonald2015). For example, as discussed in Section ‘Dietary changes’, an in-depth analysis of the mammal community at Hall’s Cave using stable isotopes (Box 1) found that, with the exception of the felid guild, the dietary niches vacated by extinct large-bodied species were not refilled (Smith et al., Reference Smith, Elliott Smith, Villaseñor, Tomé, Lyons and Newsome2022). The isotopic niche occupied by mammoth, mastodon, horses, camels, and extinct pronghorn and bison remained empty; surviving canids did not colonize the niche previously occupied by the dire wolf (Aenocyon (Canis) dirus). However, a trophic cascade was likely caused by the loss of the apex felid predators (i.e., Smilodon fatalis, Homotherium serum, Pathera leo atrox; Smith et al., Reference Smith, Elliott Smith, Villaseñor, Tomé, Lyons and Newsome2022). This led to the jaguar (P. onca), a former mesopredator, moving into the apex predator role and may have had impacts on species interactions at every level of the community (e.g., Estes et al., Reference Estes, Tinker, Williams and Doak1998, Reference Estes, Terborgh, Brashares, Power, Berger, Bond, Carpenter, Essington, Holt, Jackson and Marquis2011, Reference Estes, Heithaus, McCauley, Rasher and Worm2016).
Although the temporal resolution is lacking to track these hypothesized trophic cascades across the entire Holocene, the mammal community at Hall’s Cave, Texas likely experienced a profound and lasting loss of ecological complexity (Smith et al., Reference Smith, Elliott Smith, Villaseñor, Tomé, Lyons and Newsome2022). Simply tracking the loss or shifts in dietary niches does not yield a complete picture of how the extinction altered the community. Mammals with seemingly similar dietary niches based on stable isotope analyses or traditional ecological categories (e.g., Elton traits; Wilman et al., Reference Wilman, Belmaker, Simpson, de la Rosa, Rivadeneira and Jetz2014) can coexist if they further partition their niche based on body size (e.g., De Iongh et al., Reference De Iongh, De Jong, Van Goethem, Klop, Brunsting, Loth and Prins2011). Prior to the extinction, Hall’s Cave functioned in a similar way. In particular, browsers overlapped greatly in isotopic niche space, but occupied a diverse set of body sizes (Smith et al., Reference Smith, Elliott Smith, Villaseñor, Tomé, Lyons and Newsome2022). By combining information from both stable isotopes and body size, Smith et al. (Reference Smith, Elliott Smith, Villaseñor, Tomé, Lyons and Newsome2022) found that not only was there a reduction in dietary niche space, but also the variation in body sizes within niches was also reduced. If this trend was typical for mammal communities before and after the megafaunal extinction, and it likely was, it may explain much of the loss of biotic interactions (Tóth et al., Reference Tóth, Lyons, Barr, Behrensmeyer, Blois, Bobe, Davis, Du, Eronen, Faith and Fraser2019) and changes in species associations (Lyons et al., Reference Lyons, Amatangelo, Behrensmeyer, Bercovici, Blois, Davis, DiMichele, Du, Eronen, Tyler Faith and Graves2016b) found at other spatial scales (see section ‘Species co-occurrence’ for more information).
Species co-occurrence
The loss of large-bodied mammals at the late Pleistocene profoundly altered the behavior of surviving mammals, and especially their ecological interactions with each other (Lyons et al., Reference Lyons, Amatangelo, Behrensmeyer, Bercovici, Blois, Davis, DiMichele, Du, Eronen, Tyler Faith and Graves2016b; Smith et al., Reference Smith, Tomé, Elliott Smith, Lyons, Newsome and Stafford2016c; Tóth et al., Reference Tóth, Lyons, Barr, Behrensmeyer, Blois, Bobe, Davis, Du, Eronen, Faith and Fraser2019). The tendency for a species’ presence to be correlated with the presence or absence of another species (i.e., form a ‘pair’) is known as ‘co-occurrence’ (Gotelli and Ulrich, Reference Gotelli and Ulrich2010). For hundreds of millions of years, if two species formed a significant association, they tended to aggregate or co-occur (Lyons et al., Reference Lyons, Amatangelo, Behrensmeyer, Bercovici, Blois, Davis, DiMichele, Du, Eronen, Tyler Faith and Graves2016b). However, there was a fundamental reversal of this pattern in the mid-Holocene and today most significant species pairs are segregations (Lyons et al., Reference Lyons, Amatangelo, Behrensmeyer, Bercovici, Blois, Davis, DiMichele, Du, Eronen, Tyler Faith and Graves2016b). That this switch was likely tied to the extinction of megafauna was demonstrated by Tóth et al. (Reference Tóth, Lyons, Barr, Behrensmeyer, Blois, Bobe, Davis, Du, Eronen, Faith and Fraser2019). By decomposing the association strengths of species pairs into abiotic and biotic components, they were able to assess the relative influence of these components on species co-occurrence patterns over the late Quaternary. They found that while the influence of climate was constant, the influence of biotic interactions declined significantly following the megafauna extinction (Tóth et al., Reference Tóth, Lyons, Barr, Behrensmeyer, Blois, Bobe, Davis, Du, Eronen, Faith and Fraser2019). Analyses at local scales have found similar patterns, which provide additional context for understanding these continental scale changes. For example, the co-occurrence structure of mammals within the Hall’s Cave community in Texas also changed with the extinction (Smith et al., Reference Smith, Tomé, Elliott Smith, Lyons, Newsome and Stafford2016c). Pre-extinction significant aggregations and segregations were common, although there were some differences among trophic guilds. In particular, extinct carnivores were much more likely to form aggregations than segregations, owing to tightly linked predator–prey pairs (Smith et al., Reference Smith, Tomé, Elliott Smith, Lyons, Newsome and Stafford2016c). Co-occurrence patterns changed considerably after the extinction, with few significant pairs found among carnivores. This suggested profound changes in how ecological processes influenced the structure of the mammal community.
The loss of interactions within food webs (Nenzen et al., Reference Nenzen, Montoya and Varela2014; Pires et al., Reference Pires, Koch, Fariña, de Aguiar, dos Reis and Guimarães2015; Fricke et al., Reference Fricke, Hsieh, Middleton, Gorczynski, Cappello, Sanisidro, Rowan, Svenning and Beaudrot2022), ecological complexity (Smith et al., Reference Smith, Elliott Smith, Villaseñor, Tomé, Lyons and Newsome2022) and biotic interactions (Tóth et al., Reference Tóth, Lyons, Barr, Behrensmeyer, Blois, Bobe, Davis, Du, Eronen, Faith and Fraser2019) over the late Quaternary has consequences for macroecological patterns at larger scales. For example, the relationship between trophic structure and body size in mammals forms a U shape when ordered along an axis of increasing protein in the diet (Figure 7). Indeed, the average body size of herbivores and carnivores is much larger than that of omnivores and invertivores (Hiiemae, Reference Hiiemae and Schwenk2000; Price and Hopkins, Reference Price and Hopkins2015; Pineda-Munoz et al., Reference Pineda-Munoz, Evans and Alroy2016; Cooke et al., Reference Cooke, Gearty, Chapman, Dunic, Edgar, Lefcheck, Rilov, McClain, Stuart-Smith, Kathleen Lyons and Bates2022). This relationship is consistently found across vertebrate taxa including mammals, birds, and fish, and began in mammals at least as early as 66 Ma (Cooke et al., Reference Cooke, Gearty, Chapman, Dunic, Edgar, Lefcheck, Rilov, McClain, Stuart-Smith, Kathleen Lyons and Bates2022). Mammals in different biomes also consistently show a U-shaped relationship between diet and body size suggesting this is a fundamental way for mammals to divide up energy within an ecosystem (Cooke et al., Reference Cooke, Gearty, Chapman, Dunic, Edgar, Lefcheck, Rilov, McClain, Stuart-Smith, Kathleen Lyons and Bates2022). However, as a result of the megafaunal extinction, this pattern is beginning to flatten. Because the largest herbivores and carnivores were disproportionately lost, the average body size of these trophic guilds has decreased. Future extinctions are likely to exacerbate this process (Cooke et al., Reference Cooke, Gearty, Chapman, Dunic, Edgar, Lefcheck, Rilov, McClain, Stuart-Smith, Kathleen Lyons and Bates2022).
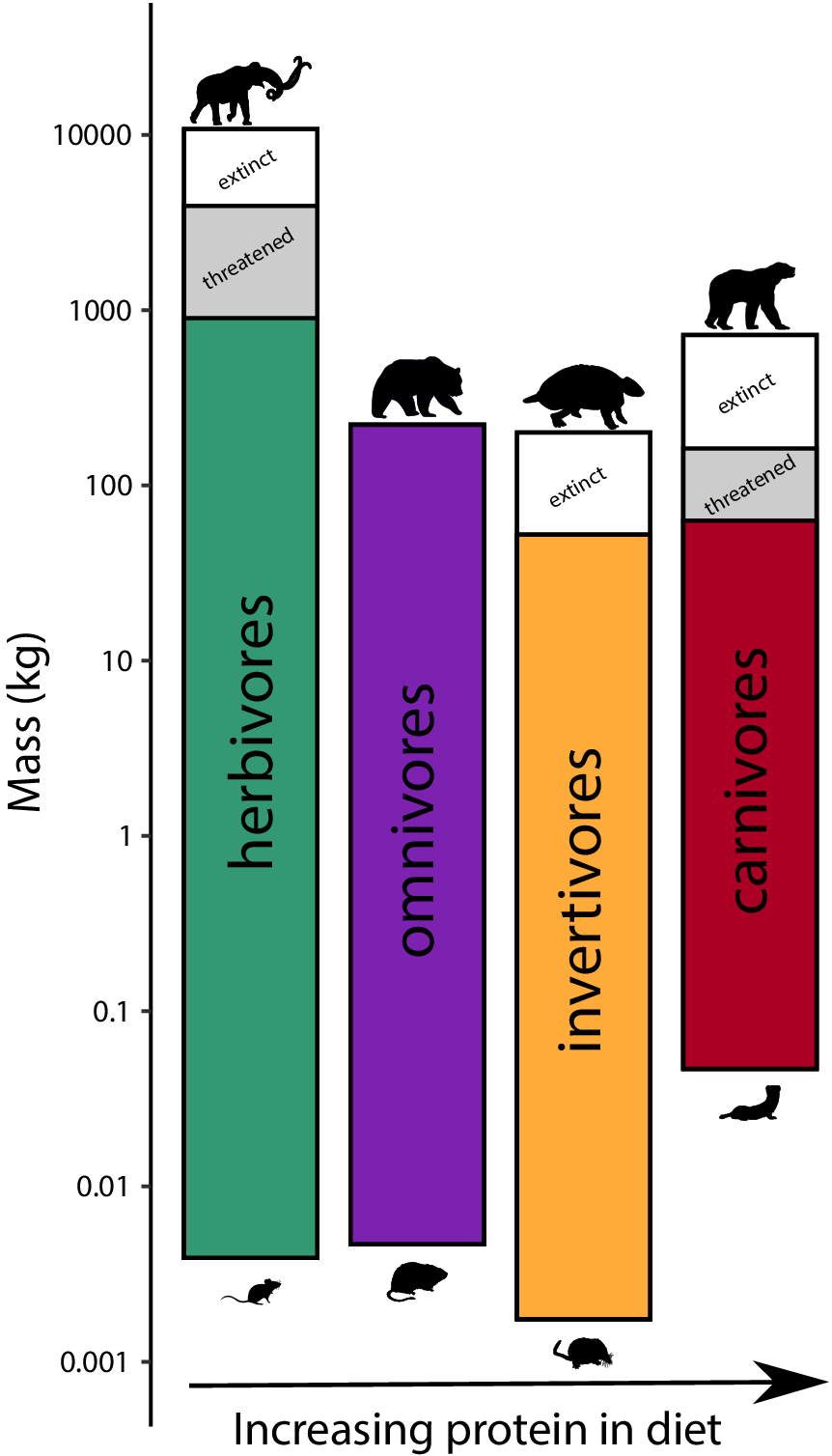
Figure 7. Relationship between body size and trophic guild for late Pleistocene Terrestrial mammals. Colored and gray boxes indicate the minimum and maximum sizes of extant mammals in each trophic guild. White boxes indicate maximum size when including extinct late Pleistocene species. The gray boxes indicate the decrease in body size expected in each trophic guild if all threatened and endangered species on the IUCN Red List go extinct. Data are from Smith et al., Reference Smith, Lyons, Ernest, Jones, Kaufman, Dayan, Marquet, Brown and Haskell2003. The largest and smallest species for each trophic guild are represented by silhouettes (PhyloPic.org). Creator credits: (top, left to right) U.S. National Park Service, xgirouxb, Xvazquez, Tracy A. Heath; (bottom, left to right) Daniel Jaron, Natasha Vitek, Becky Barnes, Ferran Sayol. Figure modified from Cooke et al., Reference Cooke, Gearty, Chapman, Dunic, Edgar, Lefcheck, Rilov, McClain, Stuart-Smith, Kathleen Lyons and Bates2022.
Changes in community structure
All of the responses documented in previous sections (e.g., changes in species movement patterns, foraging, nutrient cycling, species distributions and ecological interactions) have resulted in changes to the structure and function of ecological communities. In addition to being depauperate, mammal communities in the Holocene show changes in many of the standard metrics used to quantify community structure (Graham et al., Reference Graham, Lundelius, Graham, Schroeder, Toomey, Anderson, Barnosky, Burns, Churcher, Grayson and Guthrie1996; Lyons and Smith, Reference Lyons, Smith, Smith and Lyons2013; Lyons et al., Reference Lyons, Smith and Ernest2019; Smith et al., Reference Smith, Elliott Smith, Lyons, Payne and Villaseñor2019, Reference Smith, Elliott Smith, Villaseñor, Tomé, Lyons and Newsome2022; Fraser et al., Reference Fraser, Villaseñor, Tóth, Balk, Eronen, Andrew Barr, Behrensmeyer, Davis, Du, Tyler Faith and Graves2022; Hedberg et al., Reference Hedberg, Lyons and Smith2022). These include a profound increase in the similarity of communities across space (Graham et al., Reference Graham, Lundelius, Graham, Schroeder, Toomey, Anderson, Barnosky, Burns, Churcher, Grayson and Guthrie1996; Fraser et al., Reference Fraser, Villaseñor, Tóth, Balk, Eronen, Andrew Barr, Behrensmeyer, Davis, Du, Tyler Faith and Graves2022) and a loss of functional diversity (Davis, Reference Davis2017; Hatfield et al., Reference Hatfield, Davis and Thomas2022; Hedberg et al., Reference Hedberg, Lyons and Smith2022).
Community homogenization
The severe size-selective biodiversity loss at the late Pleistocene led to increased homogenization of mammalian fauna and biogeographic provinces in North America over the Holocene (Graham et al., Reference Graham, Lundelius, Graham, Schroeder, Toomey, Anderson, Barnosky, Burns, Churcher, Grayson and Guthrie1996). They speculated that this homogenization was due to climate change associated with deglaciation and the resulting changes in the environment (Graham et al., Reference Graham, Lundelius, Graham, Schroeder, Toomey, Anderson, Barnosky, Burns, Churcher, Grayson and Guthrie1996). Fraser et al. (Reference Fraser, Villaseñor, Tóth, Balk, Eronen, Andrew Barr, Behrensmeyer, Davis, Du, Tyler Faith and Graves2022) tested this hypothesis by examining beta diversity of mammalian communities across the last 35,000 years in 5,000-year increments. Like Graham et al. (Reference Graham, Lundelius, Graham, Schroeder, Toomey, Anderson, Barnosky, Burns, Churcher, Grayson and Guthrie1996), they found a significant decrease in beta diversity (i.e., species turnover) immediately post-extinction in North America (Fraser et al., Reference Fraser, Villaseñor, Tóth, Balk, Eronen, Andrew Barr, Behrensmeyer, Davis, Du, Tyler Faith and Graves2022). However, they did not find an association between climate change and community homogenization. Ecological communities had retained their distinctiveness over the previous 20,000 years despite climate changes associated with deglaciation (Fraser et al., Reference Fraser, Villaseñor, Tóth, Balk, Eronen, Andrew Barr, Behrensmeyer, Davis, Du, Tyler Faith and Graves2022). In addition, the magnitude of the homogenization was especially pronounced when considering only mammals larger than 1 kg, suggesting that the result was not due to reduced preservation potential of smaller-bodied species (Behrensmeyer et al., Reference Behrensmeyer, Kidwell and Gastaldo2000; Miller et al., Reference Miller, Behrensmeyer, Du, Lyons, Patterson, Tóth, Villaseñor, Kanga and Reed2014). Thus, they concluded the underlying mechanism was biodiversity loss due to the extinction and the expansion of geographic ranges by survivors (Lyons, Reference Lyons2003; Tóth et al., Reference Tóth, Lyons, Barr, Behrensmeyer, Blois, Bobe, Davis, Du, Eronen, Faith and Fraser2019; Fraser et al., Reference Fraser, Villaseñor, Tóth, Balk, Eronen, Andrew Barr, Behrensmeyer, Davis, Du, Tyler Faith and Graves2022). The increase in geographic range size was larger than expected based simply on the newly available land area due to the retreat of glaciation (Fraser et al., Reference Fraser, Villaseñor, Tóth, Balk, Eronen, Andrew Barr, Behrensmeyer, Davis, Du, Tyler Faith and Graves2022). However, the infilling of species ranges did not increase; occupancy of species’ geographic ranges was highly patchy (Tóth et al., Reference Tóth, Lyons, Barr, Behrensmeyer, Blois, Bobe, Davis, Du, Eronen, Faith and Fraser2019; Fraser et al., Reference Fraser, Villaseñor, Tóth, Balk, Eronen, Andrew Barr, Behrensmeyer, Davis, Du, Tyler Faith and Graves2022). A second wave of homogenization began in the last 2,000 years coincident with the intensification of agriculture in North America at this time (Fraser et al., Reference Fraser, Villaseñor, Tóth, Balk, Eronen, Andrew Barr, Behrensmeyer, Davis, Du, Tyler Faith and Graves2022). Although early agriculture did not produce monocultures, or alter the landscape to the extent that modern farming does (Smith, Reference Smith1994; Price, Reference Price2009), early anthropogenic impacts including increasing human population size, landscape alteration and increasing land area under cultivation (Marlon et al., Reference Marlon, Bartlein, Daniau, Harrison, Maezumi, Power, Tinner and Vanniére2013) likely contributed to biotic homogenization in the late Holocene.
Functional changes within communities
Animals fill roles within ecosystems that vary in importance to the community. These are determined by the combination of their specific ecological or morphological traits (i.e., body size, diet, period of activity, social behavior; Box 2) and how these traits interact with their local environment (Owen-Smith, Reference Owen-Smith1988; Geremia et al., Reference Geremia, Merkle, Eacker, Wallen, White, Hebblewhite and Kauffman2019; Cox et al., Reference Cox, Gardner and Gaston2021). Quantifying the range and distribution of functional traits within a community can provide a link between community composition and ecosystem function (Mouillot et al., Reference Mouillot, Villéger, Scherer-Lorenzen and Mason2011). In this section, we discuss changes in functional diversity across the terminal Pleistocene megafaunal extinction through the modern and explore how these are manifest at different spatial scales. We describe how shifts in functional composition may be linked to demonstrated alterations in ecosystem function, including habitat structure, plant diversity, biogeochemical cycling and other topics of this review. Finally, we discuss the potential role of modern exotic and domesticated large mammals in restoring late Pleistocene functional composition and the degree to which they create novel communities.
Box 2. What is functional diversity?
Functional diversity is typically quantified in multi-dimensional space constructed from relevant ecological trait axes. Taxa are plotted based on their trait values and various individual and community-level metrics calculated based on the position and distribution of the entire assemblage in functional space. Relevant metrics are listed below.
Community metrics
FVol: Functional volume, calculated using kernel density hypervolume estimation around all taxa, measures overall functional richness of a community (Blonder et al., Reference Blonder, Morrow, Maitner, Harris, Lamanna, Violle, Enquist and Kerkhoff2018; Mammola and Cardoso, Reference Mammola and Cardoso2020).
FRic: Functional richness, calculated by measuring the volume of the minimum convex hull enclosing all taxa, measures maximum functional range of a community (Villéger et al., Reference Villéger, Mason and Mouillot2008).
FDis: Functional dispersion, calculated as the average distance of all taxa to the centroid, measures functional spread of a community (Laliberte and Legendre, Reference Laliberte and Legendre2010).
FDiv: Functional divergence, calculated as the evenness in distances of all taxa to the centroid, measures functional symmetry of a community (Villéger et al., Reference Villéger, Mason and Mouillot2008).
Individual metrics
Functional distinctiveness: Mean distance to all other taxa, measures rarity of trait values compared to community as a whole (Violle et al., Reference Violle, Thuiller, Mouquet, Munoz, Kraft, Cadotte, Livingstone and Mouillot2017).
Functional uniqueness: Distance to nearest taxon, measures similarity to nearest functional neighbor (Violle et al., Reference Violle, Thuiller, Mouquet, Munoz, Kraft, Cadotte, Livingstone and Mouillot2017).
During periods of biodiversity decline such as the terminal Pleistocene, changes in functional diversity can be decoupled from changes in species richness (Petchey and Gaston, Reference Petchey and Gaston2002; Boyer and Jetz, Reference Boyer and Jetz2014). Unlike taxonomic affiliation, which inherently assumes equal contribution to diversity, the functional contribution of an animal varies depending on the redundancy or distinctiveness of their ecological traits relative to the entire community (Mouillot et al., Reference Mouillot, Bellwood, Baraloto, Chave, Galzin, Harmelin-Vivien, Kulbicki, Lavergne, Lavorel, Mouquet and Paine2013a; Violle et al., Reference Violle, Thuiller, Mouquet, Munoz, Kraft, Cadotte, Livingstone and Mouillot2017). This means that the consequences of extinction can be magnified – or ameliorated – by the particular subset of species that go extinct. Recent work exploring the ecological impacts of late Pleistocene biodiversity loss on communities has used a functional trait-based approach, allowing concrete ecological comparisons between communities across broad temporal and/or spatial scales (Dineen et al., Reference Dineen, Fraiser and Sheehan2014; Foster and Twitchett, Reference Foster and Twitchett2014; Davis, Reference Davis2017; Pimiento et al., Reference Pimiento, Griffin, Clements, Silvestro, Varela, Uhen and Jaramillo2017; Lundgren et al., Reference Lundgren, Ramp, Rowan, Middleton, Schowanek, Sanisidro, Carroll, Davis, Sandom, Svenning and Wallach2020; Hedberg et al., Reference Hedberg, Lyons and Smith2022).
The late Pleistocene extinctions resulted in a severe decline in functional diversity at local scales (Hedberg et al., Reference Hedberg, Lyons and Smith2022). For example, at Hall’s Cave, the loss of megafauna led to a significant reduction in both functional richness and volume within the community (Box 2; Figure 8A, B). Notably, the reduction in these metrics exceeded that expected if species loss were random, suggesting the large mammals extirpated contributed distinct ecological function (Hedberg et al., Reference Hedberg, Lyons and Smith2022). Indeed, an entire region of functional space was truncated (Figure 8E), leading to significant declines in functional dispersion and divergence (i.e., the spread and symmetry of taxa around the centroid of functional space, respectively) in the Holocene (Figure 8C, D; Hedberg et al., Reference Hedberg, Lyons and Smith2022).
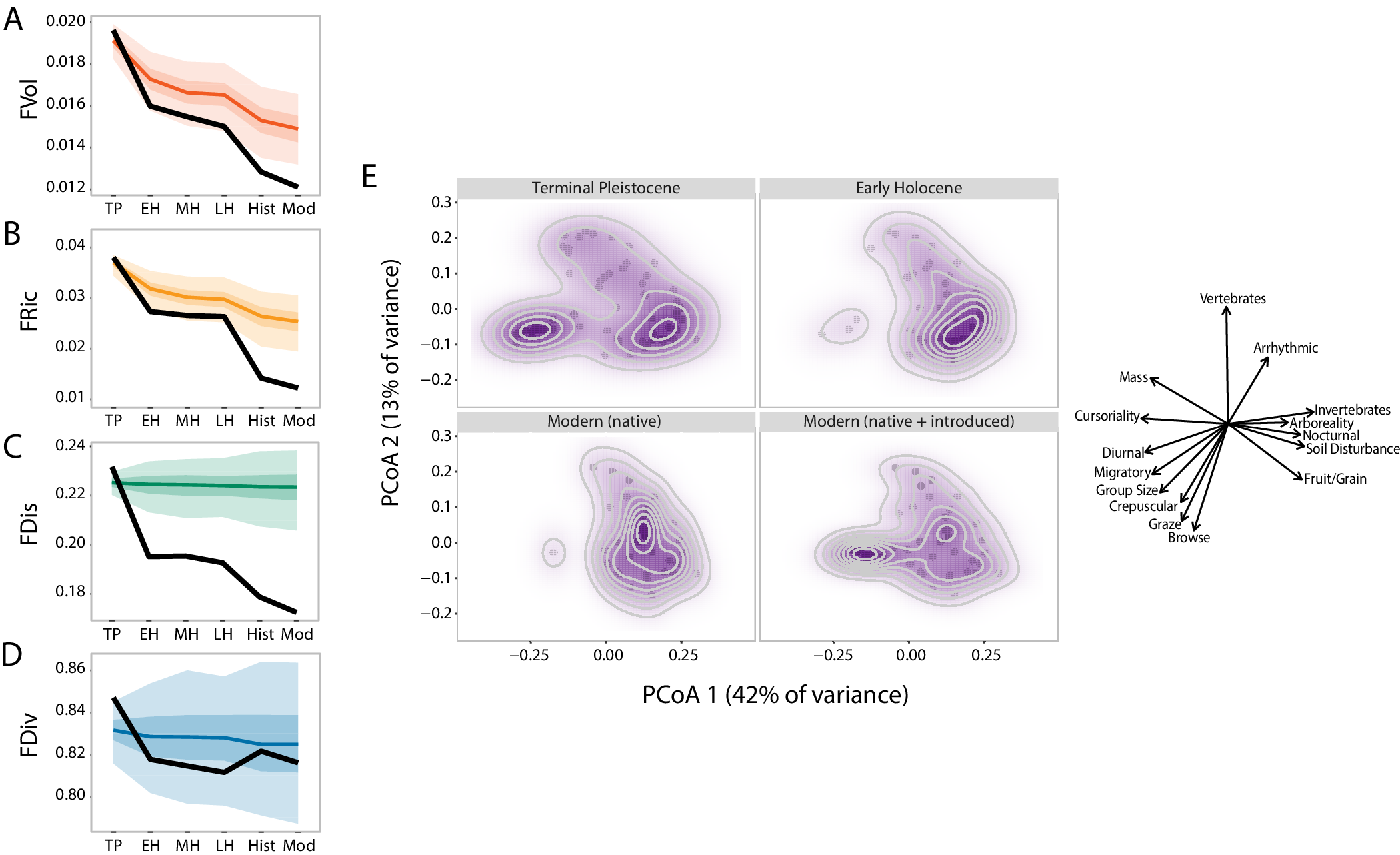
Figure 8. Key functional diversity metrics at Hall’s Cave over time. (A) Functional volume (FVol). (B) Functional richness (FRic). (C) Functional dispersion (FDis). (D) Functional divergence (FDiv). Black lines represent empirical values; colored lines represent median values from null models simulating random species loss. Shading around colored lines indicates the 50% confidence interval (darker) and 95% confidence interval (lighter) of null model values. Terms: TP, terminal Pleistocene; EH, early Holocene; MH, middle Holocene; Hist, historical; Mod, modern. (E) Functional trait space of select temporal communities at Hall’s Cave visualized on principal coordinates analysis (PCoA) axes 1 and 2 (55% of variance). Arrows on the right indicate how traits load onto each axis, with PCoA 1 primarily reflecting mass (r = −.921), invertebrate consumption (r = .996) and migration habit (r = −.901) and PCoA 2 primarily reflecting vertebrate consumption (r = .999) and browse (r = −.914) or graze (r = −.833) consumption. Each point represents a species, and fill color represents their density in functional space.
In contrast, changes in functional diversity of the entire North American megafaunal assemblage at the continental scale were less acute across this same interval. While both functional richness and dispersion declined following the Terminal Pleistocene megafaunal extinction, the loss was within the expected range of random species decline (Davis, Reference Davis2017). However, this analysis was limited to species greater than ~4 kg and declines in functional diversity became increasingly distinct from random expectation with lower minimum mass thresholds, supporting the notion that large mammals are indeed more functionally distinct compared to their smaller-bodied counterparts (Davis, Reference Davis2017). Furthermore, large mammals make up a greater proportion of local versus regional or continental-scale biotas due to greater spatial turnover in small and medium-sized mammals (Brown and Nicoletto, Reference Brown and Nicoletto1991). Thus, the ecological impact of megafaunal species loss may be more evident at smaller, more local spatial scales.
Perhaps more important than quantifying changes in aggregate functional diversity is understanding how the loss of megafauna shifted the distribution of ecological traits in surviving communities. For example, in addition to a significant truncation of the body size distribution (Smith et al., Reference Smith, Tomé, Elliott Smith, Lyons, Newsome and Stafford2016c), the Holocene community at Hall’s Cave shifted toward lower diurnal activity and lower prevalence of browse and graze consumption. Invertebrate and fruit and seed consumption became significantly more prevalent, as did fossorial and arboreal life modes (Hedberg et al., Reference Hedberg, Lyons and Smith2022). These changes in functional composition potentially signified a fundamental shift in energy flow through the ecosystem that could underpin changes in vegetation structure and composition (Asner et al., Reference Asner, Levick, Kennedy-Bowdoin, Knapp, Emerson, Jacobson, Colgan and Martin2009; Bakker et al., Reference Bakker, Gill, Johnson, Vera, Sandom, Asner and Svenning2016a). Indeed, the cave pollen record over this interval reflects an increase in tree abundance and a change from more open to closed habitats (Cordova and Johnson, Reference Cordova and Johnson2019). Though climate was also in transition over the late Pleistocene, it is likely the loss of large-bodied herbivores that strongly contributed to this ecosystem shift. Non-random changes in trait-distributions were also observed at the continental scale, with the surviving biota similarly characterized by increased levels of fossoriality and arboreality and declines in graze and browse diets (Davis, Reference Davis2017). Thus, the changes in local functional composition in central Texas were likely not unique, potentially leading to broader landscape-scale changes in ecological function. Indeed, documented cases of ecosystem transitions and changes in fire regimes and biogeochemical cycling following megafauna decline are detailed in Sections ‘Foraging’ and ‘Digestion’. Further work exploring other communities is warranted.
Functional redundancy – a specific trait or suite of traits shared by multiple members of a community – can provide ecological resilience as a buffer against loss of function following extinction or other disturbances (Fonseca and Ganade, Reference Fonseca and Ganade2001; Mouillot et al., Reference Mouillot, Bellwood, Baraloto, Chave, Galzin, Harmelin-Vivien, Kulbicki, Lavergne, Lavorel, Mouquet and Paine2013a; Oliver et al., Reference Oliver, Heard, Isaac, Roy, Procter, Eigenbrod, Freckleton, Hector, Orme, Petchey and Proença2015, Biggs et al., Reference Biggs, Yeager, Bolser, Bonsell, Dichiera, Hou, Keyser, Khursigara, Lu, Muth, Negrete and Erisman2020, Pimiento et al. Reference Pimiento, Bacon, Silvestro, Hendy, Jaramillo, Zizka, Meyer and Antonelli2020b). Redundancy is typically split into two metrics: functional distinctiveness and functional uniqueness, which refer to the mean distance to all other taxa and the distance to the nearest taxon in functional space, respectively (Violle et al., Reference Violle, Thuiller, Mouquet, Munoz, Kraft, Cadotte, Livingstone and Mouillot2017; Box 2). As implied by the severe loss of functional richness and dispersion at Hall’s Cave, Pleistocene megafauna were significantly more functionally distinct than surviving taxa (Hedberg et al., Reference Hedberg, Lyons and Smith2022). Interestingly, they were not particularly functionally unique, which suggests that although they may have possessed distinct functional traits compared to the community at large, many taxa were somewhat functionally redundant with each other. Thus, the megafaunal extinction led to a severe reduction in the functional breadth of the community but had little effect on the overall density of species within remaining functional space (Figure 8E). The surviving large mammals, however, were now the remnant occupants of a once much more populated area of functional space, and consequently, their unique functional contribution skyrocketed in the Holocene. Interestingly, many of these species, such as bison and gray wolf, are now considered keystone species in the ecosystems they still occupy (Knapp et al., Reference Knapp, Blair, Briggs, Collins, Hartnett, Johnson and Towne1999; Fortin et al., Reference Fortin, Beyer, Boyce, Smith, Duchesne and Mao2005; Ripple and Beschta, Reference Ripple and Beschta2012; Ratajczak et al., Reference Ratajczak, Collins, Blair, Koerner, Louthan, Smith, Taylor and Nippert2022).
Of course, the decline in large-bodied mammals did not end at the terminal Pleistocene. Increases in human population and land use over the last centuries have led to the continued extirpation of megafauna from many parts of the globe, including North America (Cardillo et al., Reference Cardillo, Mace, Jones, Bielby, Bininda-Emonds, Sechrest, Orme and Purvis2005; Estes et al., Reference Estes, Terborgh, Brashares, Power, Berger, Bond, Carpenter, Essington, Holt, Jackson and Marquis2011; Dirzo et al., Reference Dirzo, Young, Galetti, Ceballos, Isaac and Collen2014; Ripple et al., Reference Ripple, Wolf, Newsome, Betts, Ceballos, Courchamp, Hayward, Van Valkenburgh, Wallach and Worm2019). This includes the extirpation of bison and jaguar from much of their former geographic range (Hornaday, Reference Hornaday1887, Flores, Reference Barnosky, Hadly, Gonzalez, Head, Polly, Lawing, Eronen, Ackerly, Alex, Biber, Blois, Brashares, Ceballos, Davis, Dietl, Dirzo, Doremus, Fortelius, Greene, Hellmann, Hickler, Jackson, Kemp, Koch, Kremen, Lindsey, Looy, Marshall, Mendenhall, Mulch, Mychajliw, Nowak, Ramakrishnan, Schnitzler, Das Shrestha, Solari, Stegner, Stegner, Stenseth, Wake and Zhang2016, Sanderson et al., Reference Sanderson, Redford, Chetkiewicz, Medellin, Rabinowitz, Robinson and Taber2002). Indeed, in large part due to the inflated functional uniqueness of surviving large mammals, declines in functional diversity in the historical and modern periods at Hall’s Cave were as great if not greater than those following the terminal Pleistocene extinction (Figure 8A–D). Critically, this suggests that modern ecosystems have reduced ecological resiliency resulting from millennia of past biodiversity erosion, and may be more vulnerable to adverse ecological impacts with continued declines. A similar pattern of accelerating functional loss with past or predicted biodiversity decline has been found in marine megafauna (Pimiento et al., Reference Pimiento, Leprieur, Silvestro, Lefcheck, Albouy, Rasher, Davis, Svenning and Griffin2020a), and for large, slow reproducing species across the tree of life (Carmona et al., Reference Carmona, Tamme, Pärtel, De Bello, Brosse, Capdevila, González-M, González-Suárez, Salguero-Gómez, Vásquez-Valderrama and Toussaint2021).
The functional space lost in the Pleistocene has not gone completely unused. Humans have changed the function of communities by introducing exotic species to novel habitats. This includes many large-bodied herbivores such as zebra, oryx, gazelle and elephants brought into the United States for sport hunting (Butler et al., 2005). Especially for free-ranging populations that become naturalized, exotics may restore portions of lost ecological function. This is particularly true in South America and Australia, where including introduced herbivores to the native assemblage restored 47% to >100% of lost late Pleistocene functional richness, respectively (Lundgren et al., Reference Lundgren, Ramp, Rowan, Middleton, Schowanek, Sanisidro, Carroll, Davis, Sandom, Svenning and Wallach2020). Similarly, in central Texas, the modern non-native fauna has ‘filled in’ areas of functional space once occupied by Pleistocene megafauna (Figure 8E). However, restoring functional richness is not always the same as restoring functional composition; including introduced species generated highly distinct trait distributions from both the modern and terminal Pleistocene communities (Hedberg et al., Reference Hedberg, Lyons and Smith2022). Nonetheless, these are provocative results. They suggest that the once outlandish idea of introducing functional replacements into ecosystems (i.e., ‘rewilding’; Martin, Reference Martin1992; Donlan et al., Reference Donlan2005, Reference Donlan, Berger, Bock, Bock, Burney, Estes, Foreman, Martin, Roemer, Smith and Soulé2006) may indeed be a path forward to help preserve key ecological processes previously provided by extinct megafauna (Zimov, Reference Zimov2005; Root-Bernstein and Svenning, Reference Root-Bernstein and Svenning2016; Bakker and Svenning, Reference Bakker and Svenning2018; Lundgren et al., Reference Lundgren, Ramp, Rowan, Middleton, Schowanek, Sanisidro, Carroll, Davis, Sandom, Svenning and Wallach2020).
Summary and future directions
The extinction of all terrestrial mammals above ~600 kg in the Americas and Australia (Lyons et al., Reference Lyons, Smith and Brown2004) marked the beginning of a fundamental transition of Earth’s ecosystems; a shift from a world dominated by wildlife to one dominated by people and their livestock (Barnosky, Reference Barnosky2008; Smith et al., Reference Smith, Hammond, Balk, Elliott, Lyons, Pardi, Tomé, Wagner and Westover2016b). Continued study of the consequences of this event is making the enduring legacy of the extinction increasingly evident. As we have demonstrated here, the influence of late Pleistocene megafauna extinction extended to all aspects of the Earth system – the atmosphere, geosphere, hydrosphere and biosphere. Indeed, many contemporary ecosystems still exhibit the effects of this extinction.
Because the rate of biodiversity loss is rapidly increasing (Ceballos et al., Reference Ceballos, Ehrlich, Barnosky, García, Pringle and Palmer2015, Reference Ceballos, Ehrlich and Dirzo2017), scientists do not have time to wait for the results of long-term experimental studies before proposing potential mitigation strategies. Moreover, to date most modern studies of the ecosystem function of large-bodied mammals focus on a single system – the influence of African savanna elephants on vegetation structure and biodiversity (Hyvarinen et al., Reference Hyvarinen, Te Beest, le Roux, Kerley, de Groot, Vinita and Cromsigt2021). Other megaherbivores and aspects of ecosystem function are comparatively neglected leading to considerable deficiencies in our understanding of the ecological role of megaherbivores (Hyvarinen et al., Reference Hyvarinen, Te Beest, le Roux, Kerley, de Groot, Vinita and Cromsigt2021). By integrating paleoecology into conservation biology, we can gain unique long-term perspectives relevant to modern conservation efforts (Dietl and Flessa, Reference Dietl and Flessa2011; Barnosky et al., Reference Barnosky, Hadly, Gonzalez, Head, Polly, Lawing, Eronen, Ackerly, Alex, Biber, Blois, Brashares, Ceballos, Davis, Dietl, Dirzo, Doremus, Fortelius, Greene, Hellmann, Hickler, Jackson, Kemp, Koch, Kremen, Lindsey, Looy, Marshall, Mendenhall, Mulch, Mychajliw, Nowak, Ramakrishnan, Schnitzler, Das Shrestha, Solari, Stegner, Stegner, Stenseth, Wake and Zhang2017; Smith, Reference Smith2021). Future research should continue to examine the responses of survivors to the late Pleistocene extinctions and work toward integrating results across taxa and ecosystems. A better understanding of the synoptic role of large-bodied mammals can help garner support for conservation efforts and guide future management decisions.
Open peer review
To view the open peer review materials for this article, please visit http://doi.org/10.1017/ext.2023.6.
Acknowledgments
Many thanks to R.E. Elliott Smith, who provided analytical and computation assistance and to W. Gearty for help with Figure 7. We thank the Smith Lab at UNM for their work on the Hall’s Cave site over the years and the Texas Memorial Museum (particularly Drs. Chris Sagebiel and Ernie Lundelius) for access to collections and assistance in locating references, specimens or information about the fossil sites. We would also like to thank Drs. R. Toomey and T. Stafford, Jr. for their foundational work at Hall’s Cave, without which much of our work would not be possible, and the Hall family for graciously allowing generations of paleontologists to work at this very important late Quaternary site.
Author contributions
All authors contributed substantially to the conceptualization and writing of the manuscript.
Financial support
This work was supported by the National Science Foundation Division of Environmental Biology (Grant Numbers 1555525 and 1744223; F.A. Smith PI; S.K. Lyons and S.D. Newsome, co-PIs), and a National Science Foundation Division of Environmental Biology Research Coordination grant (2051255; S.K. Lyons PI; S.A.F. Darroch, C.V. Looy and P.J. Wagner, co-PIs). E.A.E.S. was supported by a National Science Foundation Postdoctoral Research Fellowship in Biology (DBI-1907163), and a Peter Buck Postdoctoral Fellowship from the Smithsonian Institution.
Competing interest
The authors declare none.
Comments
Dear Colleagues,
Attached please find the review I was asked to write on the ecological consequences of the late Quaternary extinctions. I think we have done an excellent job reviewing the state of the science regarding these events, and hope you will agree. I have included a 'graphical abstract', which is actually our figure 1. I wasn't sure if they could be the same thing! I have also included the names of several reviewers, which I hope will be useful as you evaluate the manuscript.
Best
Felisa Smith (and co-authors)