Introduction
Microzooplankton occupy a key position in marine food-webs as major consumers of primary producers. They act as an intermediate between primary producers and copepods and are key component of the microbial loop (Sherr and Sherr, Reference Sherr and Sherr2002; Calbet and Landry, Reference Calbet and Landry2004; Calbet and Saiz, Reference Calbet and Saiz2005; Calbet, Reference Calbet2008). The microbial loop comprises bacteria, flagellates, ciliates and other microzooplankton (less than 200 μm in diameter) in the water column (Mclachlan and Defeo, Reference Mclachlan and Defeo2018). These heterotrophic and mixotrophic organisms of 20–200 μm include many protists, such as ciliates, dinoflagellates, foraminifera, metazoans (like copepod nauplii and some copepodites) and some meroplanktonic larvae (Calbet, Reference Calbet2008). Microzooplankton are also important contributors to the diet of mesozooplankton (copepods), and have key roles in marine ecosystems as nutrient recyclers, and CO2 producers (Calbet and Alcaraz, Reference Calbet and Alcaraz2007). They are also the main consumers of phytoplankton in tropical and subtropical oligotrophic waters (Calbet, Reference Calbet2008). Their consumption represents about 50% of the phytoplankton biomass per day (Calbet and Landry, Reference Calbet and Landry2004). In Atlantic-influenced polar system, microzooplankton play a significant role in the carbon cycle which has the capacity to control primary production in both ice-covered and open waters (Lavrentyev et al., Reference Lavrentyev, Franzè and Moore2019). Microzooplankton herbivory on average removed 72% (in two experiments >100%) of daily primary production in sea ice cover.
Among planktonic groups, dinoflagellates are known for their species richness, morphological diversity and the heterogeneity of their habitat (Smayda and Reynolds, Reference Smayda and Reynolds2003). They can directly or indirectly have a significant effect on marine ecosystems, as they have important ecological implications in terms of their nutritional status (Smayda and Reynolds, Reference Smayda and Reynolds2003). Some of them can cause harmful algal blooms through their ability to produce toxins, and when they can invade existing ecosystems (Ignatiades and Gotsis-Skretas, Reference Ignatiades and Gotsis-Skretas2010). Ciliates are morphologically very diverse (Lynn, Reference Lynn2008). In the marine food web, they play a major role in grazing picoplankton and nanoplankton while at the same time serving as prey for metazoans; thus, acting as an intermediate for energy transfer to higher trophic levels (Stoecker and Capuzzo, Reference Stoecker and Capuzzo1990). Both dinoflagellates and ciliates are important in the functioning of aquatic ecosystems because they are ubiquitous and abundant in all habitat types (Laybourn-Parry and Parry, Reference Laybourn-Parry and Parry2000). Information on microzooplankton distribution is available, mainly from the western and the central basin of the north part of the Mediterranean Sea (Paraskevi et al., Reference Paraskevi, Giannakourou and Christaki2001). In the southern part, few studies have examined the microzooplankton diversity and abundance with rare evaluations of the species richness, especially in the Algerian coast.
Habibas Islands of Alboran Sea are located at 26 miles from Cape Figalo west of Oran, 10 miles from the port of Bouzedjar and 5.8 miles from the nearest continental point at Madagh II (Figure 1 and Table 1). These Islands are among the most important marine ecosystems in Algeria that constitute an essential habitat of endemic and rare terrestrial and marine species. Executive Decree n°03-147 of March 29th 2003 classifying the Habibas Islands (wilayaof Oran) as a marine nature reserve. Published in Official Journal no. 23, then as Specially Protected Area of Mediterranean Interest (SPAMIs) in 2005, during the Barcelona Convention framework (UNEP/MAP-SPA/RAC, 2020). This biological hotspot is characterized by the presence of various Mediterranean marine protected species (e.g. Lithophyllum lichenoides, Patella ferruginea, Centrostephanus longispinus, Epinephelus marginatus, Pinna nobilis) including marine mammals (e.g. Stenella coeruleoalba) (SPAMI Collaborative Platform, 2019), coralligenous substratum and the presence of underwater caves, dark or semi-obscure, in addition to the fine and/or coarse sand grounds.

Figure 1. Sampling stations in coastal waters of Algeria (southwestern Mediterranean Sea).
Table 1. Sampling station coordinates in coastal waters of Algeria

Some researchers were interested to study the biodiversity of coastal waters of Algeria such as the ecological aspects of endangered species (Larbi Doukara, Reference Larbi Doukara2019), the distribution of the Eleonora's Falcon Falco eleonorae (Peyre et al., Reference Peyre, Telailia, Benhartiga and Beddek2018) and different aspects of gorgonians populations (Benabdi, Reference Benabdi2020). From pelagic organisms, taxonomic studies of zooplankton from coastal waters of Algeria were only performed on mesozooplankton and macrozooplankton (Chaouadi and Hafferssas, Reference Chaouadi and Hafferssas2018; Khames and Hafferssas, Reference Khames and Hafferssas2019; Kherchouche and Hafferssas, Reference Kherchouche and Hafferssas2020) with no information on the microzooplankton in this area. Other research was conducted on microphytoplankton (Drira et al., Reference Drira, Hassen, Hamza, Rebai, Bouain, Ayadi and Aleya2009), prokaryotic and eukaryotic microorganisms (Elloumi et al., Reference Elloumi, Guermazi, Ayadi, Bouain and Aleya2009) and phytoplankton and ciliates (Drira et al., Reference Drira, Hamza, Hassen, Ayadi, Bouain and Aleya2010; Hannachi et al., Reference Hannachi, Drira, Hassen, Hamza, Ayadi and Aleya2011) along the Tunisian coast. However, this study reflects on the pelagic ecosystem in Algeria to bridge the gap of plankton community ecology in coastal waters of Algeria. It provides information on biodiversity and abundance of dinoflagellates and ciliates in an important Mediterranean hotspot, because of its ecological importance in marine food webs.
The main objectives of this paper are as follows: (1) study the composition of dinoflagellates and ciliates; (2) characterize their variations during the development period (planktonic bloom and post-bloom) corresponding to spring and summer according to Hinder et al. (Reference Hinder, Hays, Edwards, Roberts, Walne and Gravenor2012); and (3) analyse the influence of environmental parameters on their structure and distribution pattern.
Materials and methods
Sampling areas
The oceanographic cruise was carried out in coastal waters of Algeria from May 2012 to July 2012. A total of 47 samples were collected by Niskin-Type Plastic Water Sampler (HYDRO6BIOS PWS) at six stations from the surface mixed layer at depths of 5, 15, 30 and 50 m, then subdivided into two sub-samples. The first sub-sample consisted of 1 litre of water fixed in acid Lugol's iodine at 5%, and stored in the dark for subsequent analysis in the laboratory. It was intended for qualitative and quantitative study of microzooplankton. The second sub-sample (100 ml) was immediately frozen at −20 °C. Later it was defrosted, and an auto-analyser (SKALAR SAN PLUS) was used for setting the dosage of nutrients (nitrate, nitrite, silicate, phosphate). Temperature and salinity were instantly measured after sampling using a multi-parameter (HI 9828-12202/Romania). For the chlorophyll a, satellite images were downloaded using the internet website of National Aeronautics and Space Administration (2022). The extraction of the information from these satellite images was performed using ENVI 4.8 software. The results obtained were classified in a spreadsheet for each station.
Microscopic analysis
To identify and quantify microzooplankton, 10 ml was settled in sedimentation chambers after pre-concentration of 1 litre sub-sample for 72 h (Edler and Elbrächter, Reference Edler and Elbrächter2010). These settled samples were examined using a Zeiss Axiovert25 inverted microscope, at a magnification of ×200 and ×400. Dinoflagellates and ciliates were counted and identified to genus or species level using key identification references (Tomas, Reference Tomas1997; Strüder-Kypke et al., Reference Strüder-Kypke, Kypke, Agatha, Warwick and Montagnes2001; Avancini et al., Reference Avancini, Cicero, Di Girolamo, Innamorati and Magaletti2006; Abboud-Abi Saab, Reference Abboud-Abi Saab2008; Dolan et al., Reference Dolan, Montagnes, Agatha, Coats and Stoecker2012a). Furthermore, metazoan microzooplankton were only counted but not identified and classified as other zooplankton.
Data analysis
Microzooplankton abundance was estimated and expressed as number of individuals per litre (LeGresley and McDermott, Reference LeGresley and McDermott2010). Dominance was calculated for each season as follows:

where n i represents abundance of the i species, N represents abundance of all species and f i represents occurrence frequency of the i species. According to Xu and Chen (Reference Xu and Chen1989), species is dominant when Y ≥ 0.02.
The commonly used diversity parameters, including species richness, Pielou's evenness (E) and Shannon–Wiener diversity index (H′) (Magurran, Reference Magurran2013), were calculated for each sample. The non-parametric Mann–Whitney test was used to assess the difference of diversity indices between the two seasons.
Non-metric multidimensional scaling (NMDS), based on the Bray–Curtis distance, was used to identify different samples assemblage. They were compared using analysis of similarity (ANOSIM), a non-parametric multivariate analysis of variance test (Clarke, Reference Clarke1993). The main species responsible for creation of similarity patterns between groups assessed by ANOSIM were identified using similarity percentage analysis (SIMPER) (Clarke, Reference Clarke1993). In order to assess the changes of microzooplankton community through seasons relative to environmental variables, multivariate statistical analyses were used (Borcard et al., Reference Borcard, Gillet and Legendre2018). To check if unimodal constrained ordination methods were appropriate an initial detrended correspondence analysis (DCA) was performed (Smilauer and Leps, Reference Smilauer and Leps2014). The length gradient was more than 3 units of standard deviation, indicating heterogeneous dataset for which canonical correspondence analysis (CCA) were appropriate. The most significant environmental factors (temperature, salinity, chlorophyll a, nitrate, silicate and seasons) were selected among ten initial factors (i.e. temperature, salinity, NO2, NO3, PO4, SiO2, Chlorophyll a, depth, stations and other microzooplankton) using the model-building method to create the best model for CCA (Gardener, Reference Gardener2014). The significance of each environmental variable was tested with a Monte Carlo permutation test (999 permutations). Also, Spearman correlation test was used to evaluate pairwise correlations between species abundances and environmental factors. All statistical analyses were performed using R (R Core Team, 2020) and python (Van Rossum, Reference Van Rossum2021).
Results
Environmental factors
In coastal waters of Algeria, the sea surface temperature ranged between 15.2 and 19.9 °C in spring (average of 16.89 °C) and 17.10 and 24.50 °C in summer (average of 21.55 °C) (Figure 2). The temperature gradient was wider in summer (5–45 m) than in spring (5–20 m) (Figure 3). Statistical difference of sea surface temperature was found between spring and summer (P < 0.05, Mann–Whitney test). For the sea surface salinity, significant differences were noted between the two seasons (P < 0.05, Mann–Whitney test). Values were lower in spring, ranging from 34 to 34.90 with an average of 34.52, than summer with a minimum of 34.70, a maximum of 35.20 and an average value of 35.00 (Figure 2). The vertical variation of salinity was more evident in spring than in summer (Figure 3).

Figure 2. Box plot of physico-chemical parameters (A: temperature, B: salinity, C: nitrite, D: phosphate, E: silicate, F: nitrate) in spring (green) and summer (red). The vertical lines (whiskers) represent the range of the data outside of the central 50% of the data, which is represented by the box. The dots outside of the whiskers represent outliers.

Figure 3. Temperature and salinity contour maps of sampling stations in the coastal waters of Algeria in spring and summer.
Significant differences were observed between spring and summer (P < 0.05, Mann–Whitney test) concentrations of chlorophyll a. Maximum values were in May (0.41–0.47 mg m−3), while minimum was registered in July with values that did not exceed 0.30 mg m−3 (Figure 4). The nitrite concentrations recorded in spring were low (0.02–0.33 μmol l−1) compared to summer (0.04–1.11 μmol l−1) and showed a significant difference between spring and summer (P < 0.05, Mann–Whitney test). For silicate and phosphate concentrations, significant differences were noted between both seasons (P < 0.05, Mann–Whitney test) too. In fact, silicate concentrations during spring were higher (from 2.22 to 3.14 μmol l−1) comparatively to summer (from 1.12 to 2.22 μmol l−1). Phosphate concentrations were also high in spring (from 0.11 to 2.07 μmol l−1) conversely to summer (from 0.013 to 0.17 μmol l−1). The nitrate concentration did not show any significant difference between seasons (P > 0.05, Mann–Whitney test) ranging from 0.05 to 0.99 μmol l−1 in spring and from 0.009 to 1.32 μmol l−1 in summer (Figure 2).

Figure 4. Chlorophyll a of surface layer (5 m) of the six sampling stations in coastal waters of Algeria in spring and summer.
Species composition
A total of 98 dinoflagellates, eight tintinnids and seven naked ciliates were identified during the cruise (Table 2). Species richness recorded 76 species (72 dinoflagellates, three naked ciliates and one tintinnid) in spring and 81 species in summer (67 dinoflagellates, seven tintinnids, seven ciliates). There were only 38.9% of species (41 dinoflagellates and three naked ciliates) that were common between spring and summer, while 61.1% of species were specific to each season (Figure 5).
Table 2. List of microzooplankton taxa recorded per season in coastal waters of Algeria

+, present; −, absent.

Figure 5. Seasonal distribution (Venn diagram) of dinoflagellates and ciliates species in coastal waters of Algeria in spring (green) and summer (red) (values indicate the number of species found in each season).
Total abundances
Abundances of microzooplankton were higher in spring compared to summer (Figure 6 and Table 3). In spring, high microzooplankton abundances were mainly located at 5 m between station 3 (716 ind l−1) and station 6 (305 ind l−1) (Figure 6). The low values were found in layers inferior to 30 m (<200 ind l−1). In summer, microzooplankton abundances between 5 m and 30 m were low (<237 ind l−1). These values increased at 50 m (between 68 and 601 ind l−1) (Figure 6). Therefore, total abundance levels differed significantly between spring and summer (P < 0.05, Mann–Whitney test).

Figure 6. Scatterpie plot of total and relative abundance of microzooplankton groups in coastal waters of Algeria (A: spring, B: summer).
Table 3. Abundances (ind l−1) of microzooplankton with seasons and depths sampled in coastal waters of Algeria

Dinoflagellate abundances were most prevalent in spring (between 595 ind l−1 (50 m) and 1541 ind l−1 (5 m)) and summer (between 434 ind l−1 (5 m) and 1507 ind l−1 (50 m)). Ciliates appeared mostly during summer period, at stations and depths with high abundance (between 43 ind l−1 (15 m) and 79 ind l−1 (5 m)) compared to spring (between 0 ind l−1 (5 m) and 37 ind l−1 (15 m)) (Figure 6 and Table 3).
Community structure
Medians of the three main diversity indices, including species richness (18 and 22.5, respectively in spring and summer), evenness index (E spring: 0.77; E summer: 0.84) and Shannon–Wiener index (H′ spring: 3.31; H′ summer: 3.70 bits ind−1) showed no significant differences between spring and summer (P > 0.05, Mann–Whitney test) (Figure 7). However, we found a significant difference in total abundances between spring (median = 144.9 ind l−1) and summer (median = 89.89 ind l−1) (P < 0.05, Mann–Whitney test). The comparison of median partial abundances of ciliates (spring median = 0, summer median = 6) and dinoflagellates (spring median = 173.65, summer median = 86.33) groups between both seasons showed significant differences too.
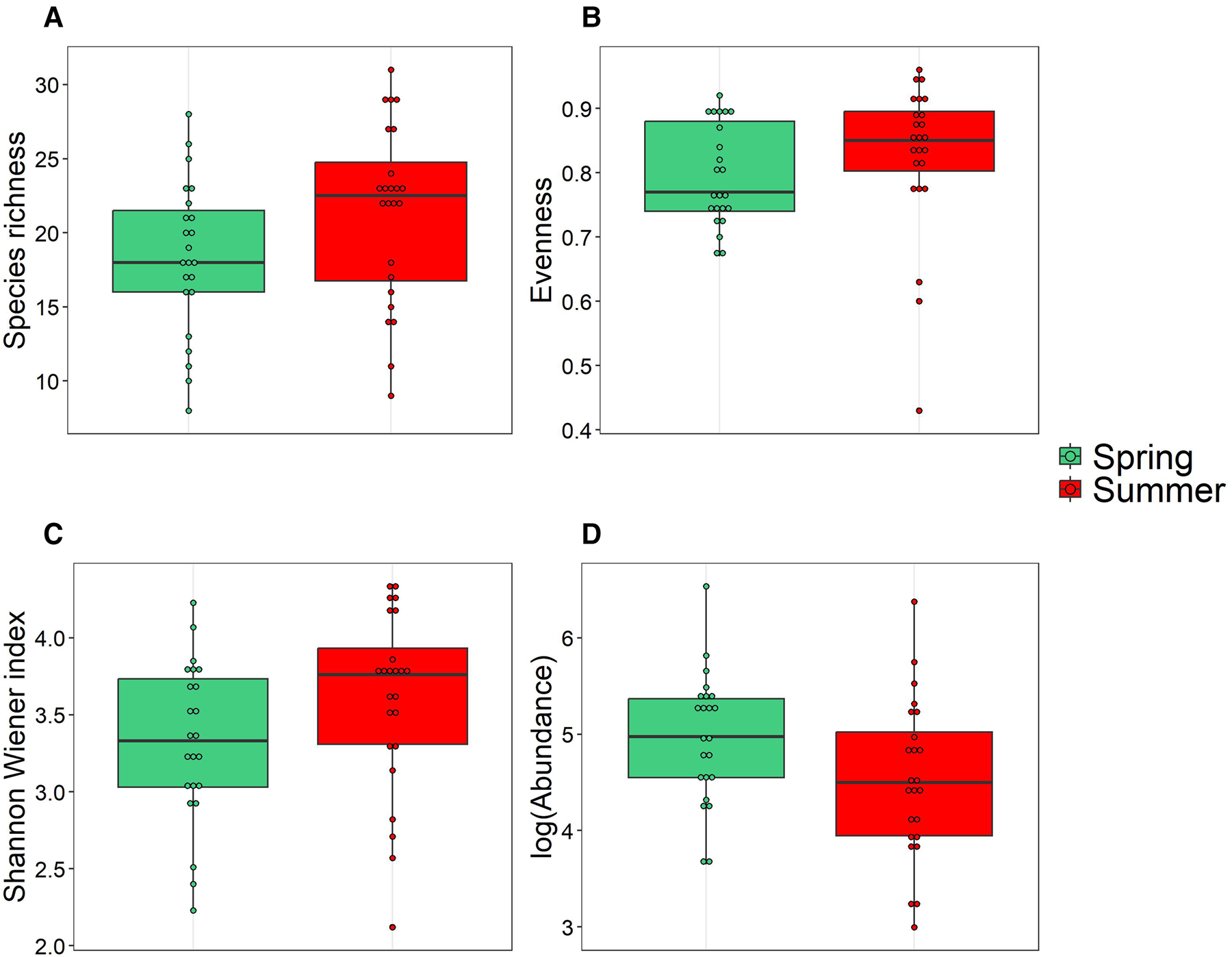
Figure 7. Boxplots of the diversity (species richness, evenness and Shannon–Wiener indices) and Logabundance of microzooplankton communities sampled in coastal waters of Algeria during spring (green) and summer (red). The vertical lines (whiskers) represent the range of the data outside of the central 50% of the data, which is represented by the box. The dots outside of the whiskers represent outliers.
The difference in microzooplankton abundances as shown in the NMDS plot (stress of 0.15) (Figure 8) clearly distinguished two groups (spring and summer) and was statistically confirmed by similarity analyses (P < 0.05, ANOSIM test). Average dissimilarity (separation) between spring and summer was high (75.86%, Simper, Table 4). As shown in Table 4, species that contribute to 70% of the dissimilarity were divided according to their dominance in three groups: (1) dominant species in spring and summer (DS) (Alexandrium spp., Tripos furca, Tripos fusus, Dinophysis caudata, Dinophysis ovum, Triadinium polyedricum, Goniodoma sphaericum, Gonyaulax polygramma, Gonyaulax spinifera, Gyrodinium fusiforme, Protoperidinium conicum, Protoperidinium divergens, Protoperidinium globulus, Protoperidinium tuba, Scrippsiella acuminata); (2) dominant species in one season and absent in the other (DA) (Tripos massiliense, Craspedotella pileolus, Diplopsalis lenticula, Gyrodinium spirale, Oxytoxum viride, Prorocentrum balticum, Protoperidinium pellucidum, Strombidium spp., Torodinium robustum); (3) dominant species in one season and rare in the other (DR) (Tripos horridus, Leegaardiella sol, Ornithocercus magnificus, Tryblionella compressa, Protoperidinium oceanicum, Protoperidinium subinerme).

Figure 8. Non-multidimensional scaling ordination (NMDS) of microzooplankton abundances from the 47 samples collected in coastal waters of Algeria.
Table 4. Results of the similarity percentage (SIMPER) analysis and the analysis of similarity (ANOSIM) of variance between two seasons

ANOSIM test: R = 0.597; P value = 0.001; average dissimilarity = 75.86.
DS, dominant species in spring and summer; DA, dominant species in one season and absent in the other; DR, dominant species in one season and rare in the other.
Dominant species in spring and summer were responsible for 42% of the dissimilarity. Species that occurred in one season and absent in the other contributed to 17.91% of the dissimilarity. Dominant species in one season and rare in the other were responsible for 11.01% of the dissimilarity.
In order to identify the relationships between microzooplankton distributions and environmental factors, CCA was illustrated in Figure 9 and Table 5. Six parameters (i.e. temperature, salinity, nitrate, silicate, chlorophyll a and seasons) were selected using the model building for shaping patterns of microzooplankton community. CCA ordination showed that the six factors were able to explain 28.5% (axe 1: 34.6%; axe 22.41%) of the total variability of community composition, across all sampling sites (Figure 9A). The samples collected during the summer period were positively related to water temperature and salinity, while samples performed in spring were positively related to silicate and chlorophyll a (Figure 9A). Ordinated abundances showed that abundances of dinoflagellates were negatively correlated with water temperature and salinity (Figure 9B). However, abundances of ciliates were negatively correlated with silicate and chlorophyll a, but positively correlated with temperature and salinity. Other zooplankton were negatively correlated with water temperature and salinity, but positively correlated with silicates and chlorophyll a.

Figure 9. (A) Canonical correspondence analysis triplot of microzooplankton abundances showing the distribution of the 47 samples (green points: spring samples; red points: summer samples) and environmental variables (blue arrows) in coastal waters of Algeria. The total explained inertia (28.5%), and partial explained inertia (axe 1: 34.6%; axe 22.41%). NO3: nitrate; SiO2: silicate. (B) Scatterpie plot of ordinated total and partial microzooplankton group abundances based on CCA simple scores.
Table 5. ANOVA-like permutation test for constrained correspondence analysis for environmental parameters through seasons and depth of sampling in coastal waters of Algeria

***Very significant, *significant, ns: not significant.
The projection on the ordination plan of dominant dinoflagellates such as S. acuminata, P. globulus, G. fusiforme and T. furca, highlighting a strong dissimilarity (Table 4), showed that abundances of P. globulus and T. furca were significantly abundant in spring, whereas G. fusiform was abundant in summer (P < 0.05, Mann–Whitney test) (Figure 10). S. acuminata did not show any significant difference between spring and summer (P > 0.05, Mann–Whitney test). However, pair-wise Spearman correlations (Figure 11) revealed that S. acuminata and P. globulus were negatively correlated with temperature and salinity, but positively with depth. P. globulus was positively correlated with silicate and phosphate, but negatively with nitrite. G. fusiforme was positively correlated with depth and nitrate but negatively with silicate. Also, a negative correlation was observed for T. furca associated to nitrite and depth whereas positive ones with silicate and phosphate.

Figure 10. Balloon plot of ordinated species abundances based on CCA sample scores sampled in coastal waters of Algeria.

Figure 11. Graphical display of a pairwise Spearman correlation matrix between species abundances and environmental factors of coastal waters of Algeria. Temp: temperature; Sal: salinity; Si(OH)4: silicate; NO3: nitrate; NO2: nitrite.
Discussion
The present assessment contributes to improve knowledge on dinoflagellates and ciliates distribution communities, in Habibas Islands, the first Algerian marine protected area. It elaborates a taxonomic list of these important groups in the marine pelagic ecosystems, with little available taxonomic information in the studied area. For a better understanding of its ecology, the spatial and spring–summer variability of dinoflagellates and ciliates communities was explored according to environmental factors.
Species composition
In this study, 98 species of dinoflagellates were identified belonging to 28 genera. However, a high number corresponding to 181 taxa was observed by Boudjenah et al. (Reference Boudjenah, Mokrane and Soualili2019) along the Algerian coastline. In the western Mediterranean Sea, Gómez (Reference Gómez2003) reported that 151 and 179 dinoflagellates species were recorded respectively in the Algerian and Alboran coasts. In the eastern Mediterranean, 157 species were identified by Lakkis and Novel-lakkis (Reference Lakkis and Novel-lakkis1981) followed by 174 taxa (Polat and Koray, Reference Polat and Koray2007). Moreover, the number of planktonic ciliates (17 species) of this study was the same number found by Rekik et al. (Reference Rekik, Ben Salem, Ayadi and Elloumi2016). However, it was very low than observed in the south coast of Sfax and the Gulf of Gabes (Kchaou et al., Reference Kchaou, Elloumi, Drira, Hamza, Ayadi, Bouain and Aleya2009; Elloumi et al., Reference Elloumi, Drira, Hamza and Ayadi2015; Rekik et al., Reference Rekik, Kmiha-Megdiche, Drira, Pagano, Ayadi, Zouari and Elloumi2021), which recorded between 56 and 63 taxas. These differences in microzooplankton diversity are linked to the study sites, the climate variability and the sampling period that affect the planktonic composition and distribution (Irigoien et al., Reference Irigoien, Huisman and Harris2004; Hays et al., Reference Hays, Richardson and Robinson2005).
Variation of abundances with environmental factors
In this study, temperature and salinity were the most relevant factors in shaping the species distribution patterns, followed by chlorophyll a, silicate and nitrate. All these factors had a significant impact on the distribution of microzooplankton abundances. This result was confirmed by comparing the indices (abundance, Shannon–Wiener, evenness and species richness). The only significant difference was between total abundances and it was confirmed by the dissimilarity analysis. Moreover, the ecological characterization of dinoflagellates and ciliates showed mature populations according to the evenness index (Magurran, Reference Magurran2013). This statement leads to oligotrophic environment.
NMDS and CCA ordinations have separated the samples into two groups according to the sampling seasons (spring and summer). By comparing the indices (abundance, Shannon–Wiener, evenness and species richness), we can state that the seasonal transition affected the abundances (high in spring and low in summer) but had no significant effect on the diversity. This was also confirmed by similarity percentage analysis (SIMPER). Moreover, the ecological characterization of dinoflagellates and ciliates showed mature populations according to the evenness index. This statement leads to oligotrophic environment.
Temperature and salinity were the most relevant factors in shaping the species distribution patterns, followed by chlorophyll a, silicate and nitrate. All these factors had a significant impact on the distribution of microzooplankton abundances.
The seasonal evolution of chlorophyll a distribution still follows the typical succession of temperate regions, characterized by a phytoplankton abundance increase in spring, a decrease during the summer season (Siokou-Frangou et al., Reference Siokou-Frangou, Christaki, Mazzocchi, Montresor, D'Alcala Ribera, Vaqúe and Zingone2010; Gasol et al., Reference Gasol, Cardelús, Morán, Balagué, Forn, Marrasé, Massana, Pedrós-Alió, Sala and Simó2016; El Hourany et al., Reference El Hourany, Abboud-abi Saab, Faour, Mejia, Crépon and Thiria2019). Similar observations were reported in distinct regions of the northern Adriatic Sea (Mozetič et al., Reference Mozetič, Francé, Kogovšek, Talaber and Malej2012). Phytoplankton, in the north-eastern Adriatic Sea in the period 2010–2017, showed a maximum abundance characterized by a bloom in spring, with a peak in May (Cerino et al., Reference Cerino, Fornasaro, Kralj, Giani and Cabrini2019). Relatively high values (up to 1.7 g C m−2 d−1) have been reported in the Catalan front area in March (Moran and Estrada, Reference Morán and Estrada2005) and in the Alboran Sea in May–June (Lohrenz et al., Reference Lohrenz, Wiesenburg, DePalma, Johnson and Gustafson1988).
Microzooplankton are a major predator for phytoplankton in the ocean (Sherr and Sherr, Reference Sherr and Sherr2002; Calbet and Landry, Reference Calbet and Landry2004), and they are themselves important prey for zooplankton (Calbet and Saiz, Reference Calbet and Saiz2005). Then, changes in the temperature could have also significant direct effects on the composition, abundance, feeding activities and growth efficiency of local microzooplankton species (Rose and Caron, Reference Rose and Caron2007; Caron and Hutchins, Reference Caron and Hutchins2013).
During spring, high dinoflagellate abundances were observed in coastal waters of Algeria in May. Similar observations were reported in the southwestern Mediterranean, at Tunis (Daly Yahia-Kéfi et al., Reference Daly Yahia-Kéfi, Souissi, Gómez and Yahia2005) and Ville-Franche bays (Gómez and Gorsky, Reference Gómez and Gorsky2003), where dinoflagellates were numerous from March to May. In summer, the abundance of dinoflagellates decreased. Their maximum values were registered in deep layers when temperature and salinity were low. Dale et al. (Reference Dale, Edwards and Reid2006) reported that under unfavourable conditions, dinoflagellates have a competitive advantage due to their ability to swim to deeper layers (characterized by low temperatures and nutrients). In addition to physical variables such as temperature and depth, food requirements are related to dinoflagellates population dynamics. Most of them are mixotrophic or heterotrophic (Ismael, Reference Ismael2003). Their diverse feeding mechanisms allow them the ability to feed on diverse prey items including bacteria, picoeukaryotes, nanoflagellates, diatoms, other dinoflagellates and ciliates (Stoecker et al., Reference Stoecker, Hansen, Caron and Mitra2017).
In this study, high abundances of ciliates were associated with high temperatures and low silicate concentrations. According to Ohtsuka et al. (Reference Ohtsuka, Yamaguchi and Hanamura2011), high abundance of ciliates was recorded with increasing temperature values, suggesting that this factor controlled their abundance, which could also affect their life cycle. Our results were similar to those of Makhlouf et al. (Reference Makhlouf, Touahria and Seridji2014) where low abundance of ciliates was observed during spring diatom bloom, occurred in coastal waters of Algeria. The dominance of diatoms coincided with the highest silicate concentration (Rekik et al., Reference Rekik, Ben Salem, Ayadi and Elloumi2016). Thus, this may be attributed to the inability of microzooplankton and particularly ciliate population to graze on large-cell phytoplankton (Froneman, Reference Froneman2004; Dolan et al., Reference Dolan, Pierce, Yang and Kim2012b).
S. acuminata, P. globulus and T. furca in this study were largely accounted with increasing spring dinoflagellates abundance. Observations from many studies indicated that blooms of plankton coincided with the increasing of diatom abundance (Martin-Jézéquel et al., Reference Martin-Jézéquel, Hildebrand and Brzezinski2000; Menden-Deuer et al., Reference Menden-Deuer, Lessard, Satterberg and Grünbaum2005; Kremp et al., Reference Kremp, Tamminen and Spilling2008). In the Bay of Tunis and during spring, Scrippsiella spp. was associated with the bloom-forming diatoms. Also, Gribble et al. (Reference Gribble, Nolan and Anderson2007) reported that the heterotrophic Protoperidinium had the potential to consume up to 80% of dinoflagellates or diatoms. However, since the majority of Protoperidinium species were widespread in the spring in our study area, food availability may be the most important factor in regulating the seasonal dynamics of different Protoperidinium species. Generally, heterotrophic dinoflagellates are considered important diatom grazers (Tiselius and Kuylenstierna, Reference Tiselius and Kuylenstierna1996; Sherr and Sherr, Reference Sherr and Sherr2007; Löder et al., Reference Löder, Meunier, Wiltshire, Boersma and Aberle2011). Strom et al. (Reference Strom, Fredrickson and Bright2019) showed that microzooplankton grazers in the Gulf of Alaska consumed nearly all of the small phytoplankton production and an average of half the production in the larger (diatom) size fraction, in spring and summer. Moreover, high concentrations of dinoflagellates with a remarkable dominance of T. furca have been recorded many times during this period in Ligurian sea (Gómez and Gorsky, Reference Gómez and Gorsky2003; Tunin-Ley et al., Reference Tunin-Ley, Labat, Gasparini, Mousseau and Lemée2007). This trend is the result of the mixotrophic capacity of this species (Gómez et al., Reference Gómez, Moreira and López-García2010). According to Gómez and Gorsky (Reference Gómez and Gorsky2003), when the microzooplankton reached phototrophic peaks, they can feed on the organic matter reserve. In addition, T. furca is able to prey on a wide variety of prey (small dinoflagellates, ciliates and flagellates) with a preference for small ciliates (Smalley et al., Reference Smalley, Coats and Adam1999). During spring, S. acuminata, P. globulus and T. furca appeared under high phosphate levels and were positively associated with silicate concentrations. In comparison with other phytoplankton groups, dinoflagellates have higher levels of nucleic acids that could imply higher phosphate requirements (Costas and Lopez-Rodas, Reference Costas and Lopez-Rodas1991). In the bay of Tunis, Daly Yahia-Kéfi et al. (Reference Daly Yahia-Kéfi, Souissi, Gómez and Yahia2005) asserted that high silicate values were related to dinoflagellates. In parallel, when dinoflagellates (thecate and athecate species) feed on diatoms, pallium-feeding thecate dinoflagellates reject empty silicate frustules (Jacobson and Anderson, Reference Jacobson and Anderson1986). Whereas athecate dinoflagellates produce mini-faecal granules composed mainly of frustules (Strom and Strom, Reference Strom and Strom1996; Horner et al., Reference Horner, Postel, Halsband-Lenk, Pierson, Pohnert and Wichard2005; Saito et al., Reference Saito, Ota, Suzuki, Nishioka and Tsuda2006). Then, diatom consumption by heterotrophic dinoflagellates also has an impact on biogeochemical cycles, since diatoms produce biogenic silica cell walls, which are recycled of Si (OH)4 in the microbial loop when grazed (Schultes et al., Reference Schultes, Lambert, Pondaven, Corvaisier, Jansen and Ragueneau2010).
In this study, the genera Gyrodinium peaks during summer as reported by Gómez and Gorsky (Reference Gómez and Gorsky2003). Despite the presence of some dinoflagellate species capable to form red tides such as T. furca, this phenomenon was not detected in this study. It is likely due to poor nutritional conditions of the studied area with low nitrate concentrations (Ferrier-Pages and Rassoulzadegan, Reference Ferrier-Pages and Rassoulzadegan1994). Scrippsiella and Gyrodinium presented maximum abundances in summer at low nutrient concentrations and consequently a mixo-heterotrophic behaviour can be expected. Mixotrorophy has been reported in S. acuminata (Stoecker, Reference Stoecker1999) and heterotrophy in G. fusiforme (Ismael, Reference Ismael2003). Also, Daly Yahia-Kéfi et al. (Reference Daly Yahia-Kéfi, Souissi, Gómez and Yahia2005) reported genus Scrippsiella as the most abundant species in the Bay of Tunis during summer.
In conclusion, our study clearly showed that the community structure of planktonic ciliates and dinoflagellates varied greatly between seasons and denoted a seasonal affinity in most species. During spring and summer, the environmental factors such as temperature and salinity influenced the abundance and the distribution pattern of dinoflagellates and ciliates in coastal waters of Algeria. Their ecological characterization showed mature populations according to the evenness index, leading to oligotrophic environment. The observed oligotrophy of this islandic environment is a characteristic of this region, especially during the summer with low chlorophyll a and nutrients. However, the seasonal transition influenced significantly the abundance variation of both dinoflagellates and ciliates, due to changes in environmental factors. In contrast, the diversity is less affected by the spring–summer transition in coastal waters of Algeria.
In order to better understand the ecology of the microzooplankton community in the Algerian coast, we suggest more intensive studies on the mesoscale spatial distribution of their abundances and biomasses, in relation to environmental factors. Also, further investigations using molecular tools could be interesting to study the physiology of cells, to characterize the diet and highlight the mixotrophy behaviour.
Acknowledgements
This research is a part of a thesis study, which is being carried out in Biological Oceanography and Marine Environment Laboratory, at the University of Science and Technology Houari Boumediene. We thank the National Coastal Center (CNL) and the National School of Marine Sciences and Coastal Planning (ENSSMAL). We also would like to thank Dr Glaucia Fragoso from the Trondheim Biological Station of the Norwegian University of Science and Technology, for her constructive comments on the previous version of the manuscript. We thank MEFTI Mohamed Abdelghafour, TAHAR BELKACEM Maria and SELMI nihed achouak for their English review of this paper, SELLAMI Abdelaziz and BELKHEIR Amine for their help in designing the database.
Author contributions
R. S. carried out the sampling and experimentation, summarized the results and performed statistical analysis. G. E. Y. K. contributed to the study conceptualization, data collecting and statistical expertise. Z. A. helped in the discussion of the results and writing and editing the manuscript and R. S. A. supervised the work.
Competing interest
None.