1.1 Introduction
This book is intended mainly as an introductory source for researchers focusing on any aspect of estuarine studies, for example, biological, biochemical, geochemical, physical. More generally, this volume is intended for those investigating all facets of semienclosed coastal bodies of water. The text presents information at a basic level, but perhaps moves up to an intermediate level in some sections. The book should provide fundamental concepts to interdisciplinary studies in these systems and to advanced hydrodynamics investigations. The core purpose of this text is to offer relevant concepts accessibly. In some parts, the offerings are succinct and in others they are reiterative. The reason for occasionally restating or reiterating concepts is to try to present them from different angles in search of lucidity.
Unquestionably, there is a surging need to understand the wide array of processes that occur in semienclosed coastal bodies of water, including estuaries. These systems appear sprinkled along coastlines throughout the world. Close to 75% (26 out of 35) of the largest cities in the world, in terms of population, are found in or influenced by estuarine systems. A higher percentage has a direct impact on estuarine waters through river connections. Worldwide, estuaries serve various purposes: they help preserve freshwater resources in rivers by limiting saltwater intrusion; they provide habitat and nursey grounds for commercially and ecologically pivotal species; they act as river-related sediment and pollutant receptacles, hampering contamination in coastal waters; they enable tourism and recreational activities; they host harbors and related commercial activities like fishing; and they could potentially provide clean energy. Threats from sea-level rise, from increased pollution, saltwater intrusion, freshwater consumption, and storm intensity, as well as threats from changes in river discharge, are already occurring in coastal regions. Therefore, we need to understand current conditions affecting semienclosed basins so that we can propose possible consequences to all of their functions (some of them mentioned above).
1.2 Classification
When studying the hydrodynamics of semienclosed basins, we may sometimes be distracted by trying to determine whether the basin is an estuary. According to the originally proposed definition, an estuary has three main characteristics. First, it is a semienclosed basin or body of water. Second, the basin has free communication or connection with the ocean. Third, land-derived drainage dilutes ocean salinity concentrations within the basin. Under these conditions, tidally averaged flows, or flows averaged over days, months, or years, should display buoyant outflow of estuarine waters and inflow of relatively heavier oceanic waters. This circulation is referred to as gravitational circulation as it results from density gradients under gravity’s action. Gravitational circulation can also be called density-driven exchange flow as it describes mean or residual flows moving in opposite directions.
We need to remember that the concept of gravitational circulation disregards instantaneous tidal flows. We also shall consider that there are examples of semienclosed basins that display gravitational circulation with no dilution of ocean waters (e.g., Chapter 11). These basins may not fit the “formal” definition of an estuary but certainly behave dynamically as one. Moreover, there are basins that show opposite or reverse (or inverse) gravitational circulation with inflow of relatively buoyant waters and outflow of heavy (or hyperpycnal) basin waters. Such a condition appears in highly evaporative basins where ocean water becomes concentrated with salts. Again, just because evaporative basins fall short in fulfilling the “formal” definition of an estuary, it does not preclude them from dynamically behaving as one. Therefore, defining an estuary becomes a challenging proposition: difficult to define but we know when we encounter one.
Classifying estuaries or semienclosed basins in different categories may provide general information on how they behave dynamically. However, classifications should be used with caution as they overlook crucial details of each system. Nonetheless, we present here different ways of classifying estuaries on the basis of their geomorphology, their water balance, and their water column stratification. More comprehensive classifications are described in Chapter 12, after understanding basic physical mechanisms in estuaries.
1.2.1 Classification Based on Geomorphology
Based on their geological origin, semienclosed basins may be grouped into the following categories: drowned river-valley or coastal plain; fjord or drowned glacier-valley; tectonic and bar-built (Figure 1.1).

Figure 1.1 Classification based on geomorphology showing the four different types, with typical cross-sections along transect L to L′.
Drowned river-valley or coastal plain estuaries were river basins more than ~15,000 years ago, during the last glacial maximum, when mean sea levels were roughly 120 m below present day. Drowned-river valley estuaries took shape between 15,000 and 8,000 years ago, as sea levels rose because of ice melting, and flooded the former river basins. Typical examples in the United States include the Chesapeake Bay, Delaware Bay, and the Hudson River. River basins continue to modify these estuarine basins, which typically are influenced by comparatively heavy sediment loads and have relatively shallow depths (order 10 m).
Fjords were formed in a similar way as drowned river-valleys. Instead of having been rivers, fjords were glaciers or “rivers of ice.” Fjords took shape as glaciers melted through flooding the glacier valley with relatively warmer ocean waters. These basins are relatively deep (order 100 m) and have steep-sided bathymetries. Many fjords receive freshwater input from river discharge. At the highest latitudes, however, some fjords can still be fed by glaciers (tidewater glaciers or ocean-terminating glaciers). Fjords appear in relatively high latitudes (e.g., Scandinavia, Greenland, Antarctica, British Columbia, Alaska, Chile, and New Zealand).
Fjords with tidewater glaciers provide direct information on climate change. The interaction between the fjord’s gravitational circulation and the ocean-terminating glacier can represent a feedback mechanism that accelerates glaciers’ melting rates. The more the glacier melts, the stronger the gravitational circulation and the larger the heat fluxes from warm ocean waters to the submerged glacier face, thus enhancing its melting. Implications of this feedback are also far-reaching as melt water from land, through its seaward advance in the glacier, can contribute to rising sea levels.
Tectonic estuaries have been formed by earthquakes or faults that suddenly or gradually shift the Earth’s crust adjacent to the ocean over distances on the order of kilometers. Such motions of the crust, which can be horizontal or vertical, allow ocean incursions. In some cases, such as in the San Francisco Bay in California, tectonic faults may also allow channelization of river valleys. Other examples of tectonic semienclosed basins are found in the Galician Rias of Spain, Guaymas Bay, Mexico, and Manukau Harbor, New Zealand.
Bar-built estuaries have become semienclosed basins because of sediment drift along the coast, or littoral drift. This sediment transport elongates from a headland, giving way to a spit or sand bar that separates the mainland from the ocean. Bar-built basins are characterized by a relatively narrow inlet, of order 100 m up to 1 km. They often display several inlets that interrupt barrier islands and sand bars. Bar-built estuaries may also be formed by rising sea levels that isolate barrier islands separated by inlets. This type of estuary is widely found in temperate, subtropical, and Mediterranean climates. Examples abound in the east coast of the USA.
1.2.2 Classification Based on Water Balance
On the basis of water balance, semienclosed basins may be characterized in three types: positive or typical estuaries, negative or inverse estuaries, and low-discharge basins (Chapter 11). Positive or typical estuaries are the most widely studied. They are basins influenced by a positive water balance, that is, gains of fresh water. This means that freshwater input from river discharge plus precipitation (rain), plus ice melting and submarine groundwater discharge exceeds water losses from evaporation plus freezing and seepage through the ground. Positive estuaries display relative buoyant water inside the basin compared to the ocean’s water density. Over the long term (days to months to years), the situation described by a positive density gradient (Figure 1.2a) favors the gravitational circulation that consists of outflow of buoyant waters and inflow of ocean waters. Typical estuaries are found predominantly in temperate regions or in weak-seasonality tropical climates.
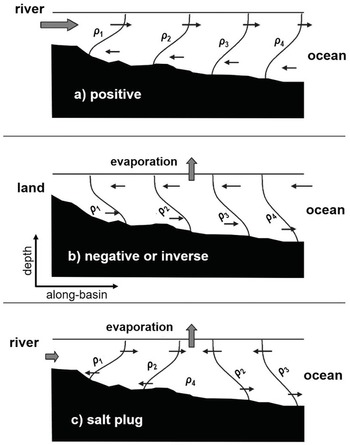
Figure 1.2 Classification based on water balance showing the three different types, with typical along-basin cross-sections of density and flow. Arrows are for illustrative purposes as they are not scaled proportionately to other frames. In all density isolines (isopycnals), .
Inverse estuaries are found in arid and semiarid areas where water losses prevail over water gains. This situation establishes negative density gradients that are inverse to those in typical estuaries. Inverse density gradients drive, over the long term, inflow of relatively buoyant waters and dense-water outflow (Figure 1.2b). Because of net water losses, inverse estuaries exhibit sluggish water flushing and should be more susceptible to water quality problems than typical estuaries.
Low-discharge basins are also found in arid and semiarid regions, including Mediterranean climates. In these systems, evaporation rates may be on the same order of magnitude as freshwater input rates. Depending on whether the buoyancy inputs are from heat fluxes or water fluxes, the basin’s circulation may vary from typical to inverse or, in some cases, may exhibit salt-plug characteristics (Figure 1.2c). In the salt-plug situation, the landward portion of the basin displays gravitational circulation. The portion connecting to the ocean exhibits inverse gravitational circulation because of a region of local salinity maximum inside the basin. These low-discharge basins attract the attention of an entire chapter (Chapter 11) in this volume.
1.2.3 Classification Based on Water Column Stratification
This approach considers the qualitative competition between stratifying and mixing agents in a semienclosed basin. Stratification is typically induced by buoyancy forcing from river discharge while mixing arises mostly from tidal or wind forcing. Another way of regarding the buoyancy vs. mixing competition is through comparison of volume of the tidal prism (tidal range times surface area) versus volume of freshwater input (e.g., Chapter 10). The result of the mixing vs. buoyancy effects ultimately determines the strength of water column stratification (Figure 1.3).

Figure 1.3 Classification based on water column stratification showing the four different types. Along-basin sections display mean density and flow fields. Freshwater volume decreases from top to bottom and tidal prism increases from top to bottom. Arrows are not scaled.
When tidal currents are relatively strong and river discharge is relatively weak, vertical stratification will be absent or relatively weak. This situation is analogous to tidal prisms overwhelming freshwater volumes from buoyancy input. The mismatch in volumes results in vertically mixed or vertically homogeneous semienclosed basins, such as coastal lagoons, as well as wide and shallow embayments. Expected mean velocity profiles in vertically homogeneous basins are unidirectional with depth (Figure 1.3). Exchange flows will then be segregated laterally (laterally sheared), as studied in Chapters 7 and 8.
With river discharge increasing, vertical stratification should increase and result in weakly stratified or partially mixed basins. This occurs with moderate to strong tidal forcing and relatively weak to moderate river discharge. In this scenario, vertical stratification can vary greatly within one tidal cycle. Partially mixed conditions may be observed in lagoons or in estuaries with relatively low river discharge. Many temperate estuaries fall in this category and may become moderately stratified. The mean salinity profile displays a barely appreciable pycnocline or nearly continuous stratification throughout the water column. Mean flows tend to display the most vigorous volume exchange, compared to other stratification conditions (Figure 1.3). This vigorous exchange ensues from vertical mixing that maintains robust horizontal density gradients.
With further increased river discharge and comparatively decreased tidal forcing, the basin becomes strongly stratified. This situation can result from moderate to relatively large river discharge and relatively weak to moderate tidal forcing. Strong stratification (say surface-to-bottom salinity difference >5 g/kg) persists throughout the tidal cycle as in fjords and other deep (>20 m deep) estuaries. The mean salinity profile exhibits a pronounced halocline (pycnocline) with small (<2–3 g/kg) vertical variations in the profile above and below the halocline. Mean exchange flows are well developed but not as robust as in partially mixed basins because of comparatively reduced vertical mixing and decreased horizontal density gradients (Figure 1.3).
Salt-wedge basins develop with large river volume inputs compared to the tidal prism. There are examples of this type of basin throughout the world where tidal ranges are relatively small (microtidal basins). In most of these basins, salt wedges are markedly tidal. Flood tides wedge salty waters into a riverine basin. Wedge-type stratification appears in this tidal phase in which a layer of fresh water is separated by a bottom salty-water layer by a sharp pycnocline. Ensuing ebb tides are dominated by vertically homogeneous riverine waters. Therefore, salt wedges appear only in flood tides. In deep enough basins where the ebbing fresh waters remain from reaching the bottom, the wedge is semi-permanent and displays relatively short horizontal excursions.
1.3 Take-Home Message
As seen, any given semienclosed basin may change from vertically homogeneous to strongly stratified in one tidal cycle. These stratification changes can occur at various temporal scales and that is why it may sometimes be irrelevant to classify a basin with these guidelines unless we specify the gamut of variability and its temporal scales. A basin typically changes geomorphology over hundreds to thousands of years and its classification will likely remain the same in our lifetime. However, a basin may change water balances in monthly to seasonal scales and vertical stratification in even shorter periods. Because of this variability, we should be cautious when trying to classify any semienclosed basin. Other robust approaches to classifying these basins are presented in Chapter 12, which includes a dynamical approach. So, before understanding such an approach, we need to familiarize ourselves with the fundamentals of the dynamics of semienclosed basins. This acquaintance is formulated in the following Chapters (2–11).