This textbook is a bit different from most that you will be exposed to in your collegiate career. One major difference is the breadth – whereas most textbooks focus on a single subject (sociology, chemistry, history, biology, economics, etc.), this textbook encompasses almost all of the traditional fields of academic knowledge. One natural result of this breadth is that you will not learn as much about any one of those disciplines. However, one of the goals of this textbook is to show you how these fields are interrelated, and that by seeing the world through multiple disciplines, you can gain a much greater understanding of the various factors that are responsible for the world and Universe that you see today.
One of the growing fields of study in academics has been the “Big History” movement. The purpose of Big History is to give students a broader perspective of the past. Big History is typically taught in history departments, but this textbook will show you how the events of the past are the result of so many factors that the past must be viewed through multiple disciplines.
The main underlying theme of this book is the need to use science, in various forms, to understand the causes of past events, even in the recent past. We all understand that we use scientific disciplines like physics and astronomy to understand the origins of the Universe, geology to understand the formation of the Earth and movement of the continents, and biology to understand the evolution of life. But we can also use science to understand much more recent phenomena.
When we examine a historical event such as World War II, we frequently look at it from the perspective of the individuals involved in the conflict. For example, we may read about D-Day from the viewpoint of a soldier on a beach in Normandy, facing a barrage of incoming machine gun fire. Or we may read about the women building tanks and airplanes on the assembly lines, and their material contribution to the war effort. Or we may read an account by African American veterans from the 761st Tank Battalion, who had to fight racism in segregated combat units. This form of history is called “narrative,” in which we try to understand a broader event by seeing it from various perspectives, with the hope that, by reading enough narratives and seeing as many perspectives as possible, we will acquire a thorough knowledge of the events. Today this is probably the most common form of history, and one reason is that it is so personally compelling. Narrative allows us to “feel” what it might have been like to be involved in those historic events, and it is natural that we would enjoy this form of history.
But narrative, for all its emotional reward, will not necessarily help us understand the broader forces that drove the events. To understand the causes of past events, we need to step back and examine the events from a distance. In the case of World War II, personal narratives may not help us understand why the Allies won the war. To do that, we must take a more scientific perspective on past events. At the outset of World War II, Japan and Germany had some of the strongest industrial economies in the world. This explains why they were able to build enormous armies, air forces, and navies, and why they were able to be so successful in the early years of the war. But it is important to remember that the United States was not in the war for the first three years, when the Axis powers had so much success. Even before the war, US industrial output was greater than that of all the Axis countries combined. And the US population alone was almost as great as that of Germany and Japan (and the population of the Soviet Union, by 1942 a member of the Allies, was greater still). Once the United States was in the war, it was largely just a matter of time before the economic force of the United States, along with the enormous populations of the USA and the Soviet Union, wore down the Axis. The United States was able to manufacture more tanks, airplanes, and trucks than all the Axis countries combined, whereas the Axis countries ran out of resources and so were literally unable to continue the fight. In fact, a significant aspect of Allied strategy centered on reducing Axis industrial output (bombing factories, mining harbors) while maximizing their own. This is in no way to trivialize the contributions and sacrifices of the individuals engaged in the conflict but just to point out that understanding the bigger picture of a large-scale event like World War II means stepping back and trying to understand the larger forces that determined the outcomes. Scientific historians do exactly this, by examining relationships among, say, resources and power. Do countries with a lot of natural resources and large populations tend to be more powerful than technologically similar countries with fewer resources and smaller populations? Even though it is a historical question, it can be examined in a scientific framework.
This highlights a difference in types of causes. Broadly speaking, scientists talk about two types of causes: proximate and ultimate. In general, a proximate cause is the event that led immediately to the consequence, whereas the ultimate cause is the underlying driver of the circumstances. In World War II, we might say that the bombing of Pearl Harbor on December 7, 1941 by the Japanese Imperial Navy was the cause of the USA entering the war. But that was just the precipitating event. The underlying cause was the expansion of world power by Japan, which had conquered several Asian regions and regarded the United States as a rival in the Pacific Ocean. Under those circumstances, a conflict was more or less inevitable; the question was largely what form it would take. So the attack was the proximate cause, but the ultimate cause was Japanese expansionism.
This applies to biology as well – two male elk may fight for the right to mate with the herd of females (proximate), but the underlying reason is that natural selection has acted to make male elk aggressive, as more aggressive males are more likely to reproduce (ultimate).
What Is Science?
This textbook employs an explicitly scientific perspective on events in the past. So it is probably worthwhile to explore what science is, and why its perspective is so unique. Most of us (even scientists ourselves) have an image in our heads of what science is and what scientists look like. The stereotypical image is of someone in a white lab coat in a laboratory, and the fact is a great deal of science is done that way. Lab coats are useful for keeping your street clothes clean while working with chemicals or dissected animals, and laboratories are useful spaces for keeping materials uncontaminated and out of the elements while working with them, and for keeping unpleasant smells out of the rest of the building. Sometimes we have a positive view of science, particularly when it produces medical breakthroughs, but sometimes we have a negative view of science, for example, when discussing the potential of cloning humans.
But that is not really what science is. Science is simply a way of understanding. In its most basic form, science simply tries to describe the material world in as objective a way as possible. A scientific statement about the world has several important characteristics:
1. Any scientific statement must invoke only forces and causes that can be observed by anyone using empirical methods. Science cannot attribute actions, for example, to “spirits” that can only be seen by one privileged spiritualist.
2. Any statement that one scientist makes about the world must be testable by other scientists. For example, if a scientist attributes a flood to a meteor, based on the presence of a crater, other scientists must be able to examine the crater themselves; they cannot simply take the word of the first scientist.
3. Parsimony: if there are competing explanations, the one with the fewest assumptions will be accepted. For example, if there are two hypotheses on the origin of the Egyptian pyramids, and one requires the presence of alien spacecraft to move the stones, it will be regarded as less likely than an explanation that simply requires the presence of large numbers of workers pushing stones up ramps. The first hypothesis requires assuming something we have no concrete evidence for (aliens) and is therefore less parsimonious.
There are several important implications of this viewpoint. The first is that scientists do not speak from a position of authority. Unlike a spirit medium or palm reader, who claims to have access to privileged knowledge or powers, a scientist does not claim to have unique abilities. Within science, while there are certainly scientists who are widely respected, no true scientist would ever claim to have a perspective that is not subject to challenge. In fact, this is largely how science progresses: one scientist makes a claim that is tested by other scientists, and if the claim is found to be incorrect another may be proposed. If it is not rejected (“falsified,” as scientists often say), then the hypothesis is provisionally accepted. But scientists know that at any time, any scientific hypothesis is subject to refutation. Even if a young scientist is challenging an older, more famous scientist, the claims are examined on their own merits rather than the reputation or position of the older scientist. In this way, science is one of the most democratic forms of knowledge and study – anyone (with sufficient study) can do it, and no one is above challenge, no matter their reputation. In fact, many young scientists have made their names by successfully challenging the long-held theories of senior scientists.
The history of science is replete with hypotheses that have been accepted for a time and then modified or rejected altogether. But with every rejected hypothesis we are refining our knowledge of the Universe – we know what is not the answer, so we know to look elsewhere.
Objections to Science
Although today we often regard science as presenting a relatively authoritative perspective on the Universe, historically there have been many objections to science. One of the most common challenges to science is when a scientific discovery or model contradicts a religious text. The most well known such conflict is the debate between the scientific perspective on the evolution of species (including humans) and Creationism. Some interpretations of the Book of Genesis in the Old Testament have humans as the product of divine creation. Under this model, humans were created as a fully developed species and have no particular biological relationship to other living things on Earth (which were also created). This creationist model (which is not universally accepted by all religious authorities) is in complete contradiction with virtually any modern biological model, under which all species, including humans, evolved from ancestral forms through the forces of evolution.
There have been various responses by society to this conflict. In some of the more religious regions in the USA, one response has been to outlaw the teaching of biological evolution or force the teaching of creationist-based models, although these laws have largely been overturned in the courts during the last half of the twentieth century. Other responses have been to challenge the position of scientists in society, so that they are not seen as reliable and objective.
Another objection to science is that sometimes the implications of applying mechanistic biological models derived from animals to humans challenges our position in the natural world. Many people prefer to see humans as the pinnacle of the natural world, and if we are the product of the same forces that have produced poison ivy, shrimp, algae, and cockroaches, then we may not have any way to distinguish our species. In some ways this critique is accurate, because as far as science is concerned humans are just another species. Science does not “order” nature in any way that treats humans as, in any sense, better. Rather, we are simply different. When we study human evolution in later chapters, we will detail some of the ways in which we are different, and for some of those ways it would be hard to argue that we are superior.
Most of us will have heard the story of how our parents met: how, if one or another parent hadn’t gotten off the bus one stop early, or hadn’t decided at the last minute to come to a party, or any variety of circumstances had been slightly altered, our parents would not have met, and we, as individuals, would literally not exist. The same applies in the natural world – species are the result of random events. One implication of this is that humans, as a species, might well not have existed if any number of circumstances over the last several hundred million years had changed. Although most of us can readily understand how we, as individuals, might not have ever existed, many of us have trouble with the idea that humans, as a species, might not have existed. But this is probably the most important implication of natural selection.
The idea that humans, and the Earth itself, is the product of the random forces of geology, astronomy, biology, and physics, makes some people uncomfortable. If we are, in effect, lonely flecks of space dust in an indifferent Universe, where do we derive purpose and morality? Unfortunately, science offers no solutions here. Science simply attempts to explain the material facts of the Universe, present and past. As a consequence, science offers no statements of morality or purpose – that is the realm of philosophers, theologians, and even artists. But if philosophers and theologians are to offer us useful morals and purposes, they must have a firm understanding of the facts of the Universe, and here science plays its role – as the underlying foundation of all spheres of thought.
The Scope of This Book
One of the premises of this book is that the scientific perspective is appropriate for investigating almost any aspect of the past and present, as long as it is a question of fact. This book starts with the Big Bang, some 14.5 billion years ago, and, covering major events and epochs in the past, passes right up to today. The first part of the book covers topics that traditionally fall under hard science – physics, astronomy, geology, and biology – whereas the second part, after the arrival of humans, encompasses disciplines that are considered “softer” sciences, such as archaeology, psychology, demography, history, political science, and economics. Each of these disciplines has their own topics, methods, and research traditions, and in this book you will learn a great deal about what these many thousands of scientists and researchers have discovered over the last several hundred years. This book is a synthesis, so I am necessarily brief when delving into any one topic, but I hope that you will find enough of interest in these many topics that you will pursue some of them further.
Many of the ideas presented here are still subject to vigorous debate among scientists, and since science is a field that thrives on disagreement, sometimes there will not be a definitive answer. For many working scientists (such as myself), the debates are one of the main things that makes the field interesting. Science is not simply learning a set of facts but debating and arguing about how to interpret them. You should feel free to challenge any ideas you see in this book – look for objective evidence and see what your own conclusions are. But remember the key to scientific claims: any evidence must be available for all to see, and the explanation with the fewest assumptions is the most scientific.
The Question of Scale
One issue we have when examining questions is picking an appropriate scale. For example, when we want to know about the power of a star, we need to look at the atomic or subatomic scale where nuclear fusion occurs. Very specific forces apply at those tiny scales. But when we want to predict how stars move through space, we pull back and look at how large bodies are affected by gravity. At this level, many subatomic forces no longer apply.
In biology, scale is also important. When we want to understand how a single species of dinosaurs behaved, we might focus on a few well-preserved specimens. But when we ask about the cause of the extinction of all dinosaurs, we don’t necessarily study a single individual fossil or even a single species. In that case, we look at the appearance and disappearance of hundreds or thousands of species.
This same principle applies when examining other types of questions. Although we often read histories about individuals, science tends to be interested in broader “why?” questions. For example, the US Civil War is often presented in terms of the actions of individuals, or armies, and the ways in which one individual may have changed the course of a battle, or, potentially, the outcome of the war. One historical question is, for example: could the Confederacy ever have won? A scientific way of examining this question is to ask if a country with an agriculture-based economy has ever conquered a country with a large industrial base. How often has that happened; and if it has never happened, would we say that the Confederacy is unlikely to have won, no matter the actions of a few individuals? That question would be addressed by studying broad historical patterns rather than a single conflict. So, although it is, in a sense, a historical question, it might be answered in a scientific way by picking an appropriate scale of analysis.
Scale again is a factor when talking about events in time. Some events take place over enormous timescales. From the Big Bang to the origin of the Earth was roughly 10 billion years. Time scales of that magnitude are essentially incomprehensible for a human. We are born, live, and die within 1/10,000,000,000 of that timeframe, and so can have no way to truly understand how time passes over such a long period. On Earth, we often talk about species existing for millions or tens of millions of years, and even here we have no ability to understand this on a direct level. We apply numbers to these time intervals, but we have evolved to exist minute by minute or day to day, because we need to avoid threats and find food and water in those timeframes. But for much of world’s history and prehistory, very little large-scale change occurred day to day or even year to year. In fact, large-scale changes occurred that would have been imperceptible in the lifetimes of any animals. Today, that process continues: even as historical events occur daily, the Earth is changing in large and imperceptible (or nearly imperceptible) ways that may have dramatic effects on the future of humanity.
Box 1.1 The Bubble
This book may significantly challenge the way you view the world. This book presents explanations that may surprise you, or contradict what you have learned previously, whatever your political, philosophical, or religious perspective. In fact, that is one of the purposes of this book. An academic education should challenge you. Hopefully, as you read this book you will reexamine the way you view the world. Sometimes you will find your perspective justified, but sometimes you may find yourself comparing two seemingly compelling ideas that are incompatible with each other. That is another purpose of this book – to force you to think rigorously, not just about the Universe but about how you view the Universe. This may occasionally make you uncomfortable, but that is OK – that is what education is about – getting you out of “the Bubble.” The hope is that, by the end of the book, you will have adopted an intellectually rigorous, and sometimes skeptical, perspective that you can employ for the rest of your academic career.
Box 1.2 Progress in science
Science progresses by proposing hypotheses, which are subsequently rejected or refined, or accepted. One of the most famous examples is our understanding of the solar system. The earliest recorded solar systems placed the Earth at the center of the solar system (the “geocentric” model). This model was first formally proposed (as far as we know, based on historical documents) by the ancient Greeks: Animaxander, then Aristotle, and later Ptolemy (although Pythagoras was an early dissenter). This model was broadly consistent with the traditions of several religions, and was therefore not reexamined until almost 2,000 years later.
The track of the Sun and Moon followed predicted paths, but the orbits of the planets proved hard to explain – if they were simply orbiting around the Earth they should traverse the sky much as the Sun and the Moon. But they don’t – they make “loops” as they pass through the night sky. There were some ad hoc explanations (see the image of planetary epicycles in Figure 1.1), but Copernicus observed that it was much simpler to explain the celestial patterns if the Sun was at the center of the Solar System. The “loops” were made by planets orbiting the Sun, as the inner planets, orbiting in faster, smaller orbits, pass by the outer planets. By the turn of the seventeenth century, telescope technology had improved enough that Galileo could observe that the planets were composed of matter much like the Earth, suggesting that the “uniqueness” of the Earth was less than had originally been thought. His persecution by the Roman Inquisition for promoting the “heliocentric” model, with the Sun at the center of the solar system, is well known.
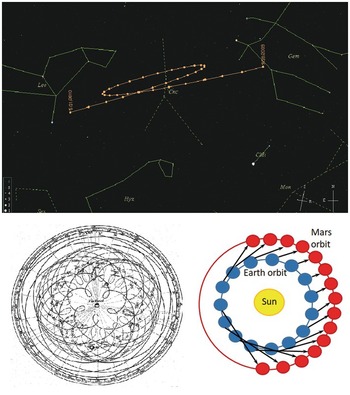
Figure 1.1 Two contrasting models for the apparent movement of Mars. As Mars passes through the sky (top of figure), it loops back over its earlier path. There are two ways that this can be explained. One is that, if Earth is the center of the solar system, Mars must be making loops (epicycles) as it orbits around Earth (lower left). This was the preferred model of the sixteenth-century Catholic Church. The second possible explanation is that Earth and Mars are both orbiting the Sun, and that our perspective on Mars changes since we are in a faster-moving, inner orbit (lower right). As we pass by Mars, it appears to reverse course. Which of these two models do you think are more parsimonious (fewer ad hoc assumptions), and therefore preferred, under the scientific model? Since there seemed to be no way to explain epicycles for orbiting planets, Galileo preferred the second model.
Copernicus and Galileo both argued for a model in which the planets (including Earth) orbited around the Sun in circles. Even seventeenth-century astronomers could see that a circular orbit could not explain the patterns of planetary movement, and it took two mathematicians, Johannes Kepler and Isaac Newton, to determine that the planets, under the attractive forces of gravity, moved in ellipses around the Sun. Subsequent discoveries (particularly general relativity) have refined the explanations, and discoveries in astronomy have moved apace.
The history of planetary astronomy is illustrative of how science proceeds. In the case of the geocentric model, subsequent discoveries and observations made it necessary to throw out the whole model (it was completely “falsified”). But in the case of the identification of elliptical orbits, science refined rather than rejected the heliocentric model. And this is how science progresses today. Sometimes models are completely discarded, but more often existing ideas are refined with the discovery of new observations, or new mathematical models to better explain the observations.
Box 1.3 What does science do and not do?
Science does a lot of things very well but there are some things it does not do at all. It is, at its most basic, simply a conceptual way of examining the Universe. But it is only interested in material facts: How old is the Earth? Where did humans come from? Why did the dinosaurs go extinct? Why is there no gorilla as large as King Kong? What causes malaria? How does rattlesnake venom kill? Are there other inhabitable planets? These are all questions that science can address. Some are very hard to answer, even with modern technology (are there other inhabitable planets?) and some are simple (what causes malaria?). These are questions of fact.
But science cannot tell you whether Vincent van Gogh was a better painter than Edouard Manet (although, in my opinion, he was). We can systematically question people about their preferences, or examine how much the paintings sell for, but this only tells us about the popularity of the painters not which was a “better” painter. Judgments on artistic merit fall under the philosophical field of aesthetics, and there people can debate the merits of either painter, and perhaps (or not) come to a consensus on judgment. But whether or not one painter is “better” is simply not a question that science can address since it does not deal with a question of objective fact. There is nothing to measure or assess objectively.
Similarly, science does not deal with questions of morality. The decision on whether an act is moral or immoral is based on a set of rules established by other people. Morality deals in “oughts” – you ought not do this act (murder), but you ought do another (throw a life preserver to a drowning swimmer). Most societies probably agree with those rules but not all, and so morality is a product of society. It is not something that is inherent to nature. In fact, animals perform acts all the time that we might well regard as immoral if done by a living human.
Sometimes difficulties arise when we see animals, particularly animals we are closely related to or are closely involved with, perform acts we see as immoral. In the early 1970s Jane Goodall, observing chimpanzees in the Gombe Preserve in Tanzania, watched as they behaved in ways that we would consider immoral in humans. They engaged in acts that would be labeled as murder in humans, and even warfare. Now, most of us would agree that it makes no sense to judge their acts in a moral framework, since they are not humans. But even when those acts are performed by humans, science makes no statements about the morality of those actions. Science has no “oughts.”
Science can, however, help provide clear facts that help us to make ethical decisions. It can illuminate the kinds of social circumstances that might lead to violence (do societies with fewer economic opportunities tend to be more violent?), just as it might help understand the social implications of various remedies to violence – for example, does capital punishment deter murder in a society? Although the essential issue of capital punishment is clearly moral, since it deals with killing, the simple objective question of a specific social response (say, a reduced murder rate) to capital punishment is a potential scientific question.
Box 1.4 Pesudoscience
Medical Pseudoscience
Sometimes ideas are presented as science yet are without any of the characteristics of true science. Typically, although not always, it is because somebody wants to sell you something. Having the aura of science can lend a product credibility. This is known as pseudoscience, and it is commonly seen in advertisements for products that claim to have medicinal powers, such as copper bracelets, therapeutic magnets, crystal therapy, “essential oils,” or water with a changed molecular structure (e.g., “memory water”).
Typically, the advertisements are full of scientific jargon that is designed to fool the consumer into thinking the product has been scientifically tested. Often the advertisements will show people with white lab coats holding clipboards or test tubes, along with anecdotal claims by “customers” who have had their pains and aches relieved by the product. But if you keep in mind the actual scientific method, you can determine which products have no basis in science.
The test:
1. Have the products been examined by a respected independent laboratory for effectiveness?
2. Did the laboratory follow normal scientific protocols for medical tests – in other words, did the laboratory test for the “placebo effect”?
3. Are the results of the test open for examination by anybody? Could we interview the test subjects themselves, or do we have to take the word of the company?
It is not always easy to determine if a laboratory is legitimate, and in the United States there are few regulations on products that do not make specific medicinal claims. It is important to keep in mind that just because a few people claim to have been “cured” by the product does not mean it works. If 12 out of 50,000 people claim relief from arthritis pain from copper bracelets, in all likelihood the relief came from another source (the placebo effect perhaps, or another medicine, or just natural healing). But the advertisements may only show the claims of the twelve who found relief.
In the United States there is long tradition of pseudoscientific fraud, going back more than a hundred years, to the salesmen of various tonics that claimed to cure any number of maladies (the original “snake oil” salesmen). The tonics in the nineteenth century were typically just a mixture of opium, cocaine, or alcohol that would temporarily relieve pain, but sometimes they were genuinely dangerous with arsenic and mercury commonly used. Today, with the internet, it is easier than ever for fraudulent hucksters to find people who are genuinely in pain and prey on their hope for relief or cure.
Nonmedical Pseudoscience
But the methods of pseudoscience are also used to convince people of a variety of other types of nonscientific beliefs. For example, claims about the powers of pyramids are sometimes framed using scientific language. Many of the claims about UFOs, especially for evidence of previous visits found in ancient rock art, are pseudoscience, since there is no way for any legitimate scientist to test the interpretations that the figures in the rock art represent ancient aliens or astronauts. This criticism also applies to claims of scientific legitimacy by advocates of extrasensory perception, crop circles, palmistry (palm reading), cryptozoology, and dowsing, among many, many others.
Sometimes you may hear of engines “powered by water” that were suppressed by oil companies or the government. Since the idea of an engine powered by water violates known physics and engineering properties, and there are no examples for scientists to examine, this falls squarely under the category of pseudoscience. Conspiracy theories, in general, fall under the category of pseudoscience, since, by their very nature, the evidence cannot be examined (and there are many – the “Moon-landing conspiracy,” the “suppressed cancer cure” conspiracy, and “cold fusion” are just a few). In the case of the water-powered engine, promotion of the idea was typically investor fraud, and several “designers” have been found guilty of fraudulently taking money from investors knowing full well the engine did not work.
Most of the broadly followed religions today existed prior to the advent of science, but some more recent religions and cults have characteristics of pseudoscience. Scientology has many of the characteristics of pseudoscience since it makes specific claims (through its “Dianetics” program) that cannot be independently examined by scientists. Similarly, “creation science” presents nonscientific ideas in a scientific framework, with the hope that the reader will be fooled into thinking that the ideas are scientific (see the section on ‘Creationism’ in Chapter 5).
In general it is a good idea to be skeptical of any scientific claim if the presenter is selling you something or wants you to join an ideology. If a claim is genuinely scientific, it will have evidence that is open for all to see, and hopefully they will have no interest in separating you from your money. Of course, one of the best ways to know if someone is presenting you with pseudoscience is to learn the actual science, so hopefully, after reading this book, you will be better prepared to defend yourself!
Box 1.5 Math is the language of nature
Most students know that math is necessary for science. This fact actually serves as an obstacle for many students who might be interested in science but don’t feel very strong in their math skills. Long ago, I was one of those kids – I loved reading about scientific discoveries like fossils and distant galaxies and lost cities in the jungle. But I was a poor math student, starting in elementary and middle school, so I accepted that I would have to be a casual consumer of science, reading about the latest finds in National Geographic rather than focusing on science and math coursework. This was my pattern, even through college, where I took only one 100-level science course in four years (I got a B−).
It wasn’t until I was thirty years old, going back to school, that I decided to give it another try. This time, something happened, and suddenly my brain was picking it all up, making connections, seeing the patterns. This is when I realized the importance of math for thinking about all aspects of life and it is part of the inspiration for this textbook.
There is no math in this book, so don’t worry about that. But it is worth exploring, just in a superficial way, why math is critical for a scientific understanding of the Universe and humanity.
The most common use of math in most sciences is the application of statistics. You may have heard that statistics is about probability and that is true, but mostly it is about whether or not we are fooling ourselves. As we will discuss later, human brains are very good at seeing patterns. In fact, they are so good that they often see patterns that aren’t real.
Let’s take an example from epidemiology. Say you hear about an old friend living near a nuclear power plant who suddenly gets cancer (a benign tumor). And you also hear about two other people who live on the same block as your friend who also have a form of cancer. Let’s say that no one on your block has cancer at all, but you are aware of someone who lives between you two who has cancer, the only one on their block. This kind of “coincidence” would make some people think that the proximity to the power plant is causing the cancer (after all, we know that radiation can cause cancer).
Let’s plot that out, since we are scientists. In Figure 1.2 the horizontal axis (X-axis) is the distance of a block to the power plant. The vertical axis (Y-axis) is how many people on that block have cancer.
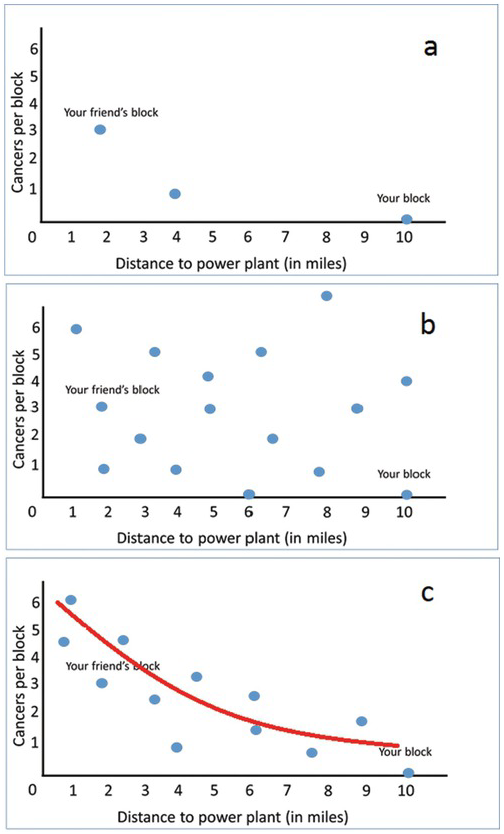
Figure 1.2 The importance of math.
When the initial data are plotted out, it certainly looks like there is a pattern (Figure 1.2a). From this, you might be justified in demanding the closure of the plant, or having the power company pay for new homes further away from the plant.
But what happens when we sample every block in the area? Let’s say we go door to door for blocks around and find out every incidence of cancer in the surrounding blocks. If we do the pattern looks something like Figure 1.2b.
So, it turns out it was a coincidence that your friend and their neighbors had cancer – by chance, there was a high rate of cancer on your neighbor’s block, and a low rate (none) on your block. The cancer rates are randomly scattered on this graph. There is no relationship between proximity to the power plant and the appearance of cancer. This is exactly why statistics was invented – to check whether our guesses are correct.
But if we had seen a pattern like in Figures 1.2c, with a lot more incidents of cancer close to the nuclear power plant, and fewer the further you went out, we would definitely have reason to suspect a radiation leak. The important thing was that we went and examined the real data, and didn’t just trust our initial instinct. We tested our hypothesis.
This is a pretty straightforward example, and you wouldn’t even have to use any math to get to the answer, just plot the data. But sometimes it is a tougher thing to estimate by just looking and we have to use math.
What may surprise you is that most statistics are pretty simple. In this case, the statistic is called a correlation coefficient, and is roughly on the level of a ninth-grade algebra problem (all you need are some averages and square roots). So it is not particularly complex, and there is no reason for students to be intimidated (most of us use statistical software anyway).
Fairly simple math also helps explain why Godzilla and King Kong cannot exist. If you look at an animal like a deer, which is a herding grazer, and compare it with an elk, which is also a herding grazer, you will notice that although the elk is less than twice as tall, it is more than four times as heavy. Why is this? The answer is that animals are three-dimensional things, so increasing size increases size in multiple dimensions. Look at a cube of three feet along each side. It is 3 × 3 × 3 feet = 27. If you double that, it is not 27 × 2 = 54, it is 6 × 6 × 6 = 216. This simple law means that getting larger makes an animal very heavy, and being massive is hard work. A gorilla is only about 30 percent taller than a chimpanzee, but it is three times as heavy (maybe 400 pounds for an adult male). This is why you could never have a gorilla as large as King Kong – a 25-foot gorilla (about five times as large as a normal gorilla) might weigh 25 tons (400 lbs × 5 × 5 × 5). A gorilla skeleton would have trouble supporting that! If you look around at nature and find really big animals, much of their bodies are legs. This is why Tyrannosaurus rex (at a mere 15 tons) had huge legs and tiny arms. And to be really big we have to look for aquatic animals, where water supports the weight.
The world provides us with information like this all the time and it is up to us to make sure we can interpret it correctly. I once met Robert Alvarez, one of the most famous geologists of the last forty years (and discoverer, with his father, Walter, of the crater formed by the huge meteor that struck the Earth 65 million years ago, wiping out the dinosaurs). We were talking about math and he said: “math is the language of nature.” What he meant is that nature is always telling us its secrets, but often we can only really understand them if we bother to understand the math.